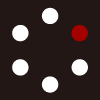
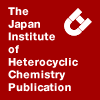
HETEROCYCLES
An International Journal for Reviews and Communications in Heterocyclic ChemistryWeb Edition ISSN: 1881-0942
Published online by The Japan Institute of Heterocyclic Chemistry
e-Journal
Full Text HTML
Received, 7th June, 2010, Accepted, 3rd August, 2010, Published online, 5th August, 2010.
DOI: 10.3987/COM-10-S(E)49
■ Immobilisation of the Vitamin B12 Derivative B12-Tyramide on Electrode Surfaces
Luisa M. Abrantes, Jorge P. Correia, Ana M. Tenreiro, and Reinhart Keese*
Institut für Organische Chemie, Universität Bern, Freiestrasse 3, CH-3012 Bern, Switzerland
Abstract
The hexamethyl cob(III)yrinic acid c-tyramide was prepared for its immobilisation on electrodes. The electropolymerisation of this compound onto Pt and glassy carbon electrodes was successful and details of these reactions were explored. The grafting procedure, in which tyramine was first electropolymerized on the electrode followed by the electro-oxidative attachment of the B12-tyramide provided a further method for this immobilisation. Exploratory experiments for the electrocatalytic activity of these coated electrodes are reported.INTRODUCTION
The preparation of surface-modified electrodes is of great interest for fundamental studies as well as for applications.1 The functionalisation of electrode surfaces leads to materials with a wide range of useful properties. Microelectronics, (bio)sensing, electrocatalysis and photo-electrochemistry are some of the areas where such modified electrodes play an important role.2 The immobilisation of catalysts on electrode surfaces has unique advantages such as, among others, the utilisation of small amounts of catalytically active species. The analysis of the molecular character of these surfaces is achieved by many of the analytical tools available for the investigation of surfaces.3,4 With the basic knowledge about the structural features, the catalysis of the polymer coated electrodes can appropriately be addressed.5
Most of the studies of modified electrodes promoted catalysis focus on metal complexes which are known to act as efficient catalysts in electron transfer reactions. Due to its intriguing redox chemistry, vitamin B12 and its congeners as well as other Co complexes have found considerable attention for their unique electroorganic reactions,6-12 for waste water treatment,13,14 and in sensing applications.15,16
Surface modifications of metal electrodes and semiconductors have been widely reported. For instance, self-assembled monolayers (SAMs) are readily formed on Au surfaces by using terminal thiol groups or disulfides.16-22 The immobilisation through self assembly of vitamin B12 disulfide derivatives is not well suited for catalytic reactions, because the reduction of the dicyano corrin [Co(III)/Co(I)] takes place very near to the potential domain where the monolayer is reductively desorbed.18
The use of polymer matrices to host catalytic species has been reported. After the pioneering work on the immobilization of the B12 complex using epoxy-resins,23,24 the most recent approach deals with the modification of electrodes by electronically conducting polymer films bearing the catalyst.25,26 The most common monomers used for the immobilisation by electrochemical polymerisation are compounds with a pyrrole- or thiophene-headgroup.27-31 A further concept is based on the incorporation of electro-catalytically active metal complexes into a sol-gel matrix.32
The authors already reported the synthesis of a corrin ring bearing a pyrrole head group in a peripheral alkyl chain and developed the conditions for its electropolymerisation. Although the interesting catalytic activity revealed by these electrodes is undoubtedly due to the presence of the B12 complex, the obtained film appears to be formed by short chains and displays poor conducting character.29,33
Here we report the synthesis of a novel monomer of a vitamin B12 derivative, bearing the electropolymerisable tyramide head group in a side chain, and developed the conditions for its immobilisation on a Pt and a glassy carbon electrode. Cyclic voltammetry and ellipsometric analysis were used for investigation of the structural features. Exploratory results of catalytic reactions are also reported.
RESULTS AND DISCUSSION
Preparation of the vitamin B12 derivative ‘B12-Ty’
Coα,Coβ-(Di(cyano-κC)-Nc-(2-(4-hydroxyphenyl)-ethyl) cob(III)yrinic Acid-c-amide a,b,d,e,f,g-Hexa-methyl Ester (2) (Scheme): The solution of 0.178 g (0.166 mmol) c-acid [Coα,Coβ-(Di(cyano-κC) cob(III)yrinic Acid-a,b,d,e,f,g-Hexamethyl Ester] (1a)34 in 15 mL CH2Cl2 was degased with Ar for 15 min. After addition of 48 mg (0.194 mmol) 2,2,2-trichloro-t-butylchloroformate (97%) and 78 mg (0.77 mmol) (C2H5)3N at – 5 °C and stirring for 2 h, 25.3 mg (0.245 mmol) tyramine (Aldrich) was added. After stirring over night the reaction mixture was poured on ice, extracted with H2O (pH = 7) (x2), treated with a small amount of KCN, washed with H2O and dried over Na2SO4. The crude product (0.209 g) in CH2Cl2 was absorbed on 3 g SiO2, and after evaporation added to 20 g SiO2 (Ar). CC with CH2Cl2-MeOH (0.01% HCN) = 50:1 and 30:1 gave 53 mg product (yield: 26.5%). A pure sample was obtained by TLC with CH2Cl2-CH3OH (0.01% HCN) = 25:1 as eluent.
Rf (CH2Cl2-MeOH (0.1% HCN) = 10:1): 0.66; IR (KBr): 3333(br)m, 2954s, 2122w, 1737s, 1662s, 1503s,1438s, 1403s, 1368s, 1355s, 1149s, 1107s, 1004s; UV/Vis (4.354x10-5 mol/L in CH2Cl2, λmax, (lgε)): 589 (3.98), 549 (3.88), 516 (3.66), 420 (3.34), 371 (4.40), 355 sh (4.07), 317 (3.94), 278 (4.03); NMR 1H: 1.25-1.40 (12 H), 1.41-1.5 (3 H), 1.52-1.75 (6 H), 1.76- 2.30 (17 H), 2.38-2.55 (12 H), 2.58-2.72 (1H), 2.73-2.86 (1H), 2.95-3.10 (2 H), 3.25-3.42 (1), 3.58-3.74 (18 H), 5.51 (1 H), 6.59 (d, J = 8.3 Hz, 2H), 6.83 (d, J = 8.5 Hz, 2H), 7.05-7.19 (1 H), 7.30-7.45 (br.,1 H) ; 13C: 15.2 (q), 15.3 (q), 16.7 (q), 18.2 (q), 19.2 (q), 19.6 (q), 21.9 (q), 24.7 (t), 25.6 (t), 25.7 (t), 29.5(t), 30.6 (t), 30.8(t), 31.4 (q), 31.6 (t), 32.3 (t), 33.5 (t), 34.7 (t), 39.1 (d), 41.4 (t), 41.6 (t), 45.9 (s), 46.9 (s), 47.0 (t), 51.3 (s), 51.6 (q), 51.6 (q), 51.6 (q), 51.8 (q), 51.8 (q), 52.4 (q), 53.4 (d), 56.6 (d), 58.4 (d), 58.5 (d), 74.6 (d), 77.2 (s), 82.6 (s), 91.4 (d), 102.3 (s), 106.5 (s), 115.1 (2d), 129.5 (d), 129.8 (d), 136.2 (s), 155.1 (s), 161.2 (s), 163.4 (s), 169.7 (s), 171.3 (s), 171.3 (s), 171.6 (s), 172.5 (s), 172.8 (s), 173.5 (s), 173.8 (s), 175.4 (s), 175.9 (s), 175.9 (s). ESI-MS [C61H80CoN7O14; M = 1194.27]: 1168.43 (76%) [M - CN]+, 1167.40 (100%) [M – CN - H]+, 595.27 (71 %) [M – CN + Na]2+, 570.88 ( 31%) [M – 2 CN]2+. HR-MS: m/z calc. for C60H80CoN6O14 [M – CN]+, 1167.5064, found 1167.51.
Electrochemical behaviour of vitamin B12-Tyramide
The voltammograms obtained for the heptamethyl dicyano cob(III)yrinate (1b)and for the B12-Ty derivative (2) at polycrystalline Pt are shown in Figure 1. The reduction [Co(III)/Co(II)] takes place at ~ −0.9 V and the redox conversion [Co(II)/Co(I)] occurs at rather negative values (E <−1.0 V), whereas the corrin oxidation is noticed by the two anodic peaks at about 1.0 and 1.3 V, developed when the potential is scanned towards positive values.35 The current response recorded with B12-Ty (2) shows the anodic peak at E = 0.98 V, expected for the corrin oxidation followed by a sharp current increase. This peak area consists of two oxidation steps; one is assigned to the irreversible oxidation of the tyramide group and the other one at 1.3 V is due to the second oxidation step of the corrin moiety.
Although analogous features are obtained with the GC electrode, it should be noticed that the reduction processes of the derivative take place at less negative values than on Pt. Within the larger cathodic range of the GC electrode, a new peak is seen at E = -1.4 V which is very likely due to the direct conversion Co(III)/Co(I). Both these characteristics suggest that the B12-Ty (2) is adsorbed by the GC electrode, enhanced by the interaction of the tyramide with its phenolic group. Such interactions lower the overpotential for the involved redox processes and allows to distinguish the conversion Co(III)/Co(I) in a potential window where the electrolyte solution is still stable.
Immobilisation of the B12-Ty complex (2) on electrode surfaces
In spite of observing the oxidation of tyramine at E ≥ 1.1 V, continuous potential cycling from 0.0 to 1.3 V or holding the potential at E ≥ 1.1 V was not effective to produce any change on the Pt or GC surface. Probably the monomer oxidation gives rise to short oligomers that diffuse into the solution. To overcome this difficulty, the adsorption of the B12 derivative (2) at the electrode surface must be promoted, which can be achieved through the reduction of the Co corrin leading to the release of one CN group.29,36 Accordingly, the potentiodynamic polymerisation within a wide potential range (from -1.2 to 1.3 V) has been attempted and Figure 2 illustrates the recorded cyclic voltammograms. The data for the Pt electrode show that the time lag for holding the potential at the cathodic limit plays an important role in the subsequent electrode modification and in the amount of the material deposited (Figure 2a). The well defined anodic peaks, observed at E > 1.0 V only convert into a broad wave centered at 1.0 V when the holding period is 60 s (inset of Figure 2a). Under these conditions, the large cathodic current markedly decreases from the first to the second cycle. With an increasing number of potential cycles, a new phase develops on the Pt surface. The low conducting character of this phase is responsible for the remarkable decrease in the currents associated with the redox couple [Co(II)/Co(I)], the fall in the corrin oxidation wave to E = ~1.0 V, and the delay of further oxidation of the species in solution. It is worth mentioning that this behaviour has also been observed for the electropolymerisation of tyramine itself.37 Upon further cycling, new cathodic peaks appear at -0.55 V and -0.94 V. They are attributed to the successive oxidation of the Co complex immobilised on the surface. The weak increase with the number of potential cycles is due to the poor conducting character of the hitherto formed polymer, thereafter designated as P(B12-Ty). According to the ellipsometric analysis of the deposited film a very thin layer of 11 nm thickness was formed after four polymerisation cycles.
On glassy carbon the characteristics of the first potential cycle (started at 0.0 V and scanned to negative values) showed similar features to those observed for the Pt electrode, coated with a polymer of (2) (Figure 2b). This suggests that the B12 derivative (2) is partially adsorbed on the electrode surface leading to a decrease of the overpotential for the redox processes of the Co species when compared to that of the compound in solution. However, the voltammetric characteristics drastically change with the number of the potential cycles: the current due to the oxidation of the tyramide group increases but the profile levels out with an increasing number of cycles. Instead of two cathodic peaks a broad wave centered at -0.89 V preceded by a shoulder at -0.51 V is observed.
Also, the anodic current peaks, assigned to the conversion Co(I)/Co(II) (of species adsorbed and in solution) and to Co(II)/Co(III), vanish and are replaced by a broad current wave centered at 0.31 V (Figure 2b). Undoubtedly, the electrode surface is coated by polymer formed from the B12-Ty (2), but the finger print of the deposited material is indeed different from that obtained on Pt.
Attempts to copolymerize B12-Ty (2) and tyramine from solution containing both compounds were fruitless. However, when the Pt electrode, first coated with a poyltyramine film (P-Ty), potentiodynamically electrogenerated in 30 growth cycles,37 was immersed into a solution containing B12-Ty (2), the current response revealed a continuous modification of the underlying polymer layer (Figure 3). The similarity of the profiles of this Pt electrode, designated as Pt-(P-Ty)/P(B12-Ty) to those obtained with the Pt or GC electrode, coated directly with B12-Ty (2) (Figure 2a,b) is taken as strong evidence for the successful immobilization of (2) by this two-layer procedure. Moreover, within that potential window, equivalent electric charges can be retrieved from Figure 3 for the Pt electrode, coated by the two-step procedure.
Characterisation of the modified electrodes
The electrochemical responses of the modified electrodes are depicted in Figure 4. In the case of the Pt electrode (Figure 4a), coated with P(B12-Ty), the conversion Co(II)/Co(I) at E= -0.98 V and the wave for the redox step Co(III)/Co(II) at E = -0.58 V as well as their counterparts can be clearly seen. These profiles resemble closely those previously reported by the authors for the Pt electrode coated with P(B12-Pyrrol).29 Thus, the observed behaviour provides unambiguous evidence for the successful immobilization of B12-Ty (2) on the Pt electrode surface, very likely by formation of a polymeric film, P(B12-Ty).
For the modified GC electrode, GC-P(B12-Ty), the voltammogram is almost featureless within the same potential window (Figure 4b). Besides the wave at about 0.19 V, no further correspondence with the processes observed for the modified Pt electrode, Pt-P(B12-Ty), can be found (Figure 4a); in particular, the absence of the oxidation peak at -0.78 V, and the much smaller charges involved in the anodic and cathodic processes, suggest that the B12 derivative was irreversibly changed during the immobilisation procedure.
In spite of the strong dependence of the shape and intensity on the number of potential cycles used for grafting B12-Ty (2) onto the P-Ty film on the Pt electrode (Figure 4c), the electrochemical behaviour of this modified electrode, where all conversions associated with the Co center of the corrin are apparent, strongly supports that this two-step procedure was successful. It is noteworthy that the amount of B12-Ty, grafted onto the poly-tyramine, although restricted to the available surface area of the P-Ty film, appears to be higher than that embedded in the polymer formed on the Pt electrode directly. Moreover, this modified electrode, designated as Pt-(P-Ty)/P(B12-Ty) is very stable in the potential range of -1.2 to +1.1 V(vs SCE).
Electrocatalytic activity of the modified electrodes – exploratory experiments
The catalytic activity of the Pt-P(B12-Ty) electrode towards oxidation reactions, was first probed in the oxidation of NO2- solutions containing sulphuric acid (Figure 5a). In comparison with the response of a bare Pt electrode, a 25% increase in the anodic current peak is observed, concomitant with a 40 mV shift towards a less positive value.
More relevant is the electrocatalytic character of the modified electrodes with regard to reductive pathways. This is exemplified by the dissolved oxygen reduction reaction (ORR) using the Pt-P(B12-Ty) electrode (Figure 5b). The impressive increase in the current, due to the reduction of O2 by the Co center of the B12 derivative, is larger than the one obtained for the same reaction using self-assembled monolayers prepared from B12 disulfide derivatives19 or with P(B12-Pyrrol).29
It is well known that vitamin B12 is particularly important as catalyst of reductive reactions taking place at negative potentials beyond the stability range of a Pt electrode.38 Since the polymer film on Pt is porous and may decompose in the electrolyte solution at the negative potentials desired, a glassy carbon electrode is to be preferred. As shown in Figure 6, the working potential window can be significantly enlarged when a glassy carbon electrode is used instead of Pt. Thus the modified GC electrode GC-P(B12-Ty) was used for exploration of reductions in the potential range inaccessible for Pt.
For the catalytic reduction of NO, KNO2 solutions in a phosphate buffer of pH = 4 were used as source of NO. Under these conditions about 43% of NO2- is converted into NO.39 The GC electrode coated with P(B12-Ty) exhibits high electrocatalytic activity towards the NO reduction (Figure 7). The potential values where the reaction is observed are consistent with those reported in the literature for the NO conversion into ammonia and hydroxylamine by vitamin B12 adsorbed on vitreous carbon.38 The particular property of vitamin B12 to catalyze this reaction has been assigned to the ability of the Co centre of the corrin ring to coordinate the NO group after the release of one or more CN- groups.38
For other reactions also requiring very negative potentials, such as the dehalogenation of 1,2-dichloroethane, this modified electrode displays no better electrocatalytic properties than those observed for the bare GC electrode. The dehalogenation of 3-bromo-1-propene was also studied with the Pt-P-Ty/P(B12-TY) electrode. First results are intrerpreted in terms of the reduction occurring at a slightly more negative potential than the conversion Co(II)/Co(I) (Figure 8).
The significant increase in current, the well defined peak at -1.5 V and in particular the similarity of the anodic counterpart with that recorded in the absence of the halogenated compound, provide clear indication of the unique electrocatalytic properties of this type of modified electrode toward dehalogenation reactions. In spite of these promising results, the initial response could not be recovered after subjecting the electrode to controlled potential electrolysis. Studies envisaging other electrochemical modes for the preparation of electrodes, coated with P-Ty/P(B12-Ty) in the two-step procedure, namely potentiostatic approaches to link the B12 derivative (2) to the polytyramine film, are in progress.
CONCLUSIONS
In the quest for new ways of immobilisation of vitamin B12 derivatives we have prepared the novel vitamin B12 derivative B12-tyramide (2).40 We have shown that this monomeric compound can be electropolymerised on a Pt as well as on a glassy carbon electrode. The two-layer procedure, i.e. the electropolymerisation of B12-Ty on a Pt electrode, coated with polytyramine, provides a new way for immobilisation of this Co complex. First results show these electrodes to be active in electrocatalysis. Studies envisaging other electrochemical modes for the preparation of electrodes coated with P-Ty/P(B12-Ty) by the two-layer procedure are in progress. It is hoped that the stability of the polymer P-Ty/P(B12-Ty) on GC electrodes can be improved and leads to efficient dehalogenations.
EXPERIMENTAL
General: The reactions were carried out with Romil super purity solvents. The reagents used were: Fluka: triethylamine (99.5%), 2,2,2-trichloro-t-butylchloroformate (97% purity), potassium cyanide (p.a.), Hoffman-La Roche: Vitamin B12 (cryst). For the flash chromatography (FC) and column chromatography (CC), distilled commercial-grade solvents; silica gel (0.04-0.063 mm) from Fluka. In TLC: Alugram SIL G/UV, plates with a layer 0.25 mm of silica gel, visualisation by UV. In NMR: Bruker- AC-500 Bruker- AC-300 (1H, 300 MHz; 13C, 75 MHz): δ in ppm relative to TMS (lock signal CDCl3: δ (H) 7.24, δ (13C) 77.00; 13C resonances of CH-, CH2- and CH3-groups: DEPT spectrum, specified as (d), (t) and (q) respectively. Spin-multiplicity abbreviation: singlet (s), doublet (d), triplet (t), quadruplet (q); multiplet (m). MS: Varian MAT CH7A; ionization energy 70 eV. Peaks as m/z with relative intensity (%) to the base peak. ESI-MS: Fisons Instrument Micromass Platform, Single stage quadrupol mass spectrometer; positive ion measurements (3.5 kV). Peaks as m/z with relative intensities (%) of the base peak; charges as + and ++ respectively; matrix: DTT/DTE. HR-MS: FTICR-MS Bruker Apex Ultra (7 Tesla) with an Apollo II Combi Source; ESI positive mode in the range 100 to 1400 m/z. External calibration was performed with LHRH sequence fragments produced by QCID. IR: Perkin Elmer 1600 FT-IR Spectrophotometer; KBr pills. Shift in cm-1; relative intensities: s (strong), m (medium).
Other chemicals: HPLC grade MeCN (Merck) was dried over CaH2 and distilled. Lithium perchlorate was dried in oven at 60 ºC for 24 h prior to use. Tyramine has been purchased from Sigma and used as received. The detailed electrosynthesis of polytyramine is described elsewhere.35 Aqueous solutions were prepared with ultra pure water (nominal resistivity 18 MΩcm at 25 ºC) and using reagent grade chemicals.
Electrochemical experiments: All electrochemical measurements were performed with a three electrodes/two compartments cell. Specpure Pt or glassy carbon (GC) disks (∅ = 5 mm) mounted in teflon supports, were used as working electrodes (WE). A platinum grid and a saturated calomel electrode (SCE) were the counter and reference electrodes, respectively. The WEs were polished to a mirror finishing with wet alumina powder (Buehler Alpha Micropolish II) down to 0.3 μm grade, rinsed with water and with distilled acetonitrile. Prior to each experiment, the electrolyte solutions were thoroughly de-oxygenated directly in the cell with nitrogen (purity > 99.9997%) The electrocatalytic essays were performed at room temperature. The electrochemical measurements were controlled by a potentiostat/galvanostat EG&G PAR model 273a.
Characterisation techniques: The films thickness were estimated from data obtained with a SENTECH SE 400 Ellipsometer, working with a He-Ne laser (λ=632.8 nm) at 70 º incident angle.
ACKNOWLEDGEMENT
The work at Bern was generously supported by the Swiss National Science Foundation, Project No. 1 20-43565.95 and the Bundesamt für Bildung und Wissenschaft (Project BBW 950606 within the European Research Program TMR (Contract No. ERBFMRXCT 960018). We also acknowledge Fundação para a Ciência e Tecnologia (FCT) for the project REDE/1501/REM/2005 and Carlos Cordeiro for providing data from the FTICR-MS at the Faculty of Sciences of the University of Lisboa, Portugal.
References
1. W. H. Saunders, Jr., Techniques of Chemistry, Vol. XXII, Molecular Design of Electrode Surfaces, ed. by R. W. Murray, J. Wiley & Sons, Inc. New York, 1992.
2. A. J. Bard and L. R. Faulkner, Electrochemical Methods, Fundamentals and Applicatons, J. Wiley & Sons, New York, 1980.
3. Handbook of Surface Imaging and Visualization, ed. by A. T. Hubbard, CRC Press, Boca Raton, 1995.
4. H. Arwin, Phys. Stat. Sol. (a), 2006, 188, 1331.
5. C. P. Andrieux and J.-M. Savéant, chapt. V in [1].
6. T. Darbre, V. Siljegovic, A. Amolins, T. Otten, R. Keese, L. M. Abrantes, and J. P. Correia in Novel Trends in Electroorganic Synthesis, ed. by S. Torii, Springer Verlag, Tokyo, 1998, pp. 395-398.
7. a) Y. Hisaeda in Novel Trends in Electroorganic Synthesis, ed. by S. Torii, Springer Verlag, Tokyo, 1998, pp. 399-402; b) Y. Hisaeda, Y. Inoue, K. Asada, and T. Nishioka, ibid. pp. 403-404.
8. R. Banerjee and S. W. Ragsdale, Annu. Rev. Biochem., 2003, 72, 209. CrossRef
9. M. C. Lagunas, D. S. Silvester, L. Aldous, and R. G. Compton, Electroanalysis, 2006, 18, 2263. CrossRef
10. M. Bühl, I. V. Vrček, and H. Kabrede, Organometallics, 2007, 26, 1494. CrossRef
11. J. E. Argüello, C. Costentin, S. Griveau, and J.-M. Savéant, J. Am. Chem. Soc., 2005, 127, 5049. CrossRef
12. J. Shey, C. M. McGinley, K. M. McCauley, A. S. Dearth, B. T. Young, and W. A. van der Donk, J. Org. Chem., 2002, 67, 837. CrossRef
13. K. M. McCauley, D. A. Pratt, S. R. Wilson, J. Shey, T. J. Burkey, and W. A. van der Donk, J. Am. Chem. Soc., 2005, 127, 1126. CrossRef
14. A. D. Follett and K. McNeill, J. Am. Chem. Soc., 2005, 127, 844. CrossRef
15. M. S. Lin, H. J. Leu, and C. H. Lai, Anal. Chim. Acta, 2006, 561, 164. CrossRef
16. F. H. Zelder, Inorg. Chem., 2008, 47, 1264. CrossRef
17. A. S. Viana, L.M. Abrantes, G. Jin, S. Foate, R. J. Nichols, and M. Kalaji, Phys. Chem. Chem. Phys., 2001, 3, 3411. CrossRef
18. E. Szöcs, L. Durrer, R. Luginbühl, N. Simic, A. S. Viana, L. M. Abrantes, R. Keese, and H. Siegenthaler, Surf. Sci., 2006, 600, 43. CrossRef
19. A. S. Viana, M. Kalaji, and L. M. Abrantes, Electrochim. Acta., 2002, 47, 1587. CrossRef
20. A. S. Viana, S. Leupold, F.-P. Montforts, and L. M. Abrantes, Electrochim. Acta, 2005, 50, 2807. CrossRef
21. M. Salman, N. F. Durand, C. Roche, and C. M. Pradier, Surf. Interface Anal., 2006, 38, 1276. CrossRef
22. C . J. Evans and G. P. Nicholson, Sensor Actuat. B-Chem., 2005, 105, 204.
23. A. Ruhe, L. Walder, and R. Scheffold, Helv. Chim. Acta, 1985, 68, 1301. CrossRef
24. Y. Murakami, Y. Hisaeda, T. Osaki, and Y. Matsuda, J. Chem. Soc., Chem. Commun., 1989, 1094. CrossRef
25. A. Mourato, A. S. Viana, J. P. Correia, H. Siegenthaler, and L. M. Abrantes, Electrochim. Acta, 2004, 49, 2249. CrossRef
26. E. Song, I.-H. Yeo, and W. Paik, Bull. Korean Chem. Soc., 1999, 20, 1303.
27. Handbook of conducting Polymers, ed. by T. A. Skotheim, Marcel Dekker, New York, 1986, vol. 1 and 2.
28. N. P. Rodrigues, J. Obirai, T. Nyokong, and F. Bedioui, Electroanalysis, 2005, 17, 186. CrossRef
29. R. Fraga, J. P. Correia, R. Keese, and L. M. Abrantes, Electrochim. Acta, 2005, 50, 1653. CrossRef
30. N. Diab and W. Schuhmann, Electrochim. Acta, 2001, 47, 265. CrossRef
31. F. Bedioui, S. Griveau, T. Nyokong, A. J. Appleby, C. A. Caro, M. Gulppi, G. Ochoa, and J. H. Zagal, Phys. Chem. Chem. Phys., 2007, 9, 3383. CrossRef
32. H. Shimakoshi, A. Nakazato, M. Tokunaga, K. Katagiri, K. Ariga, J. Kikuchi, and Y. Hisaeda, Dalton Trans, 2003, 2308. CrossRef
33. T. Otten, T. Darbre, S. Cosnier, L. M. Abrantes, J. P. Correia, and R. Keese, Helv. Chim. Acta, 1998, 81, 1117. CrossRef
34. a) Stepanek, R. PH.D. Thesis, ETH Zürich, 1987, No. 8069; b) Caderas, C. PH.D. Thesis, ETH Zürich, Switzerland 1987, No. 7822; c) Wolleb-Gygi, A., PH.D. Thesis, University of Bern, Switzerland, 1993; d) M. J. Pfammatter, T. Darbre, and R. Keese, Helv. Chim. Acta, 1998, 81, 1105. CrossRef
35. Y. Murakami, Y. Hisaeda, T. Ozaki, and Y. Matsuda, Chem. Lett., 1988, 469. CrossRef
36. L. M. Abrantes, J. P. Correia, R. Fraga, T. Darbre, and R. Keese, Portugaliae Electrochim. Acta, 1998, 16, 93.
37. A. M. Tenreiro, C. Nabais, J. P. Correia, F. S. S. Fernandes, J. R. Romero, and L. M. Abrantes, J. Solid State Electrochem., 2007, 11, 1059. CrossRef
38. C. H. Yu and Y. O. Su, J. Electroanal. Chem., 1994, 368, 323. CrossRef
39. S. L. Vilakazi and T. Nyokong, Electrochim. Acta, 2000, 46, 453. CrossRef
40. R. Keese, H. Siegenthaler, R. Luginbühl, M. A. Rashid, and M. Schnippering, ECS Transactions, 2007, 2, 27.