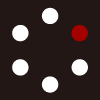
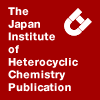
HETEROCYCLES
An International Journal for Reviews and Communications in Heterocyclic ChemistryWeb Edition ISSN: 1881-0942
Published online by The Japan Institute of Heterocyclic Chemistry
e-Journal
Full Text HTML
Received, 17th June, 2010, Accepted, 3rd August, 2010, Published online, 5th August, 2010.
DOI: 10.3987/COM-10-S(E)60
■ 2-Amino-3-(5-phenylfuran-2-yl)propionic Acids and 5-Phenylfuran-2-ylacrylic Acids are Novel Substrates of Phenylalanine Ammonia-Lyase
Csaba Paizs, Monica Ioana Toşa, László Csaba Bencze, Jürgen Brem, Florin Dan Irimie, and János Rétey*
Institute of Organic Chemistry, Karlsruhe Institute of Technology , Richard-Willstätter-Allee, D-76128 Karlsruhe, Germany
Abstract
Both racemic 2-amino-3-(5-phenylfuran-2-yl) propionic acids and 5-phenylfuran-2-yl acrylic acids were synthesized and spectroscopically characterized (UV, EI-MS, 1H-NMR and 13C-NMR). The phenyl group of the 5-phenylfuranyl residue carried no (rac-1a) para-Br (rac-1b) or Cl para or ortho (rac-1c, d) substituents. The novel furanylalanines were used as substrates of recombinant phenylalanine ammonia-lyase (PAL). When 50% of the racemic 5-phenylfuranyl-alanines were converted into the corresponding acrylates, the D-enantiomers of the substrates could be isolated. The L-enantiomers could be prepared from the substituted acrylates when the PAL reaction was reversed in the presence of 6 M ammonia at pH 10.INTRODUCTION
Phenylalanine ammonia-lyase (PAL) is an important plant enzyme, since its product, (E)-cinnamic acid is the precursor of lignines, coumarins and flavonoids. Its mechanism of action has been the subject of great interest (for a recent review see1). PAL and HAL (histidine ammonia-lyase) contain the unique superelectrophylic prosthetic group 5-methylidene-3,5-dihydroimidazol-4-one (MIO) which is necessary for the reaction.2 Previously we prepared MIO-less mutants that were essentially inactive with normal substrates but reacted with nitro-substituted ones. Since the nitro group in toluene makes the benzylic proton by 20 pKa units more acidic,3 we concluded that MIO has the same function, that is, acidification of the β-proton of the substrate. One of us proposed that the MIO group attacks the aromatic ring of the substrates and the resulting positive charge acidifies the β-proton. Although a number of results supported such a mechanism, e.g. a chemical model,4 the faster reaction of racemic 3-hydroxyphenylalanine with PAL than the natural substrate l-phenylalanine,5 recent X-ray structures with inhibitors and substrate analogues revealed that the amino group of the substrate was attached to MIO. However it may be questioned that the enzyme crystallized in the active conformation. Recently evidence for rapid conformational changes during catalysis by enzymes has been shown.6 In another case, it was found that more than 90% of the enzyme was in an inactive conformation and was in a rapid equilibrium with the active form.7 In other words, the X-ray structure shows a static structure which is not necessary the active one. At present it is not firmly established whether the Friedel-Crafts-like mechanism (Figure 1) or the NH2-MIO mechanism is correct. In a recent paper it is hypothesized that both mechanisms can work depending on the conditions.8
Another interesting property of PAL is its ability to react with a large number of arylalanines which makes it useful as enantiospecific biocatalyst. Originally, it was believed that the ammonia eliminations by PAL were irreversible. However, at a surprisingly high concentration of ammonia (6 m) the PAL reaction can be run in the reverse direction,9 i.e. the enantioselective addition of ammonia can be achieved on a preparative scale.
Thus, the pure l-enantiomers of various halogen, nitro, or cyano-substituted phenylalanines, meta and para tyrosines pyridyl- and pyrimidinyl alanines etc. have been prepared by the reverse PAL reaction.10 Here we describe the synthesis of various 5-phenylfuran-2-yl-acrylic acids as well as the corresponding amino acids both in the racemic and enantiomerically pure form.
RESULTS AND DISCUSSION
1. Synthesis of the 5-phenylfuran-2-ylacrylic acids and the racemic alanines
The 5-phenylfuran-2-carbaldehydes used as starting materials, were prepared by the Meerwein method11 from the corresponding diazonium salts of various anilines and 1-furan-2-carbaldehyde.
On the one hand, these were transformed with triphenyl-λ5-phosphanilidene acetic acid ethyl ester into the corresponding acrylic acid esters which were hydrolysed in the presence of NaOH yielding the desired cinnamates. On the other hand, the aldehydes were transformed into chloromethylene derivatives followed by their coupling with N-protected diethyl amino malonate. By mild basic hydrolysis of the formed diethyl esters, N-protected amino malonic acids were obtained that were decarboxylated in boiling toluene. In a final step the protecting group was removed and the formed phenylalanine analogues were isolated by precipitation at their isoelectric point (Figure 2).
2. Determination of the kinetic constants for the heteroaryl alanines
The kinetic measurements were based on the ultraviolet spectrophotometrical (UV) determination of the concentration of the formed acrylates. For this purpose first their extinction coefficients (ε) were determined in 0.1 M Tris-buffer (pH 8.8) at wavelengths (λ) where the corresponding amino acids do not show absorption. The enzyme assays were carried out in 1 ml cuvettes in the above mentioned buffer at 30 °C in presence of 2.5 µg (2.5×10-3 iU) of PAL varying the substrate concentration between 20-100 µ m. In Table 1 the maximal velocity (Vmax) values relative to that of phenylalanine and the Michaelis constants (Km) values are listed.
3. Biocatalysis
There are some examples for using purified PAL10,12 or Rhodotorula glutinis cells13 in preparative scale production of l-amino acids starting from the corresponding acrylates.
Exploiting the results obtained by the kinetic measurements we performed the synthesis of D-1a-d incubating at 30 °C the corresponding racemates with different amounts of PAL (Table 2, Figure 3) in 0.1 M Tris-buffer at pH 8.8. The progress of the reaction was monitored by HPLC using a Chirobiotic T column. When the L-amino acids were completely transformed into acrylates the reaction was stopped by adjusting the pH to 1.5 and the acrylates were filtered off. The enzyme was precipitated by heating the mixture to 90 °C, followed by filtration of the turbid solution. The D-amino acids were isolated and purified from the filtrate by ion exchange chromatography.
For the production of the l-amino acids, acrylic acids (E)-2a-d were incubated with PAL (Table 2, Figure 4) in 6 m ammonia buffered at pH 10.2 by bubbling CO2, conducting the reaction and the work-up procedure was as described above.
4. Discussion
Recombinant PAL from parsley is a useful biocatalyst. Its broad substrate specificity has been demonstrated by the conversion of various β-arylaminopropionates in the forward and β-arylcinnamates in the reverse reaction. Most of these substrates had a six-membered ring in the β-position and differed only in their substituents (OH, F, Cl, Br, NO2, CN)10b,c and heteroatoms (N) in the ring.10a Recently we demonstrated that also furan-2-yl, thiophen-2-yl, benzofuran-2-yl and benzo[b]thiophen-2-yl acrylates and alanines12 are also substrates for recombinant PAL from parsley. Here we described a new class of substrates (1a-d) and (2a-d) in which the β-substituent is a 5-phenylfuran-2-yl group. Thus in the binding site the hydrophobic pocket, normally harboring the β-aryl group, is large enough to accommodate also the much longer 5-phenylfuranyl residue. This finding suggests further experiments with possible substrates carrying larger substituents in β-position. Thereby the biocatalysis of novel chiral aminoacids would be available. Such amino acids could serve as starting materials for the synthesis of biologically and/or medically active compounds.
Comparing the kinetic parameters of rac-1b-d (Table 1), the highest rate for the PAL catalysed reaction was found for the para-bromo derivative rac-1b, although its affinity to the enzyme was rather low as shown by its high Km. In the case of the chlorides the enzymatic transformation was faster for para-substituted rac-1c than for the ortho-isomer rac-1d. It seems that the more electron-withdrawing substituent located in the closer position to the furan ring retards the enzyme-catalyzed reaction and this tendency, based on the comparison between rac-1b-d, is consistent with the lower electrophilicity of the furan ring towards the Michael acceptor in MIO. This hypothesis supports the Friedel-Crafts-type mechanism (Figure 5).
Our present results do not allow to decide between the two possible mechanisms of the PAL reaction. Either the Friedel-Crafts type (Figure 5) or the NH2-MIO mechanism can be formulated with the new substrates.
CONCLUSIONS
Here we described the chemical synthesis and spectroscopic characterization of 5-phenylfuran-2-yl- acrylic acids and the corresponding alanines in the racemic form. The new compounds turned out to be good substrates of recombinant PAL and could be resolved into their enantiomers by biocatalysis. The optical rotations of the latter were also determined.
EXPERIMENTAL
1. Materials and methods
1.1. Analytical methods
The 1H- and 13C-NMR spectra were recorded at 25 ºC with a Bruker spectrometer operating at 300 MHz and 75 MHz, respectively. Electron impact mass spectra (EI-MS) were taken on a LCQ advantage by ThermoFisher spectrometer operating at 1950-2050V, the samples were dissolved in MeOH: H2O, (50: 50, v/v), or MeOH: MeCN, (50/50, v/v). High Performance Liquid Chromatography (HPLC) analyses were conducted with a HP 1200 instrument using an Astec Chirobiotic-Tag column (4.6×250 mm) and a mixture of methanol: TEAA buffer (pH 4.1), (80:20, v/v) as eluent for enantiomeric separation of rac-1a-d. For all chiral compounds, high resolution enantiomeric separation was performed. Retention times for l- and d-1a-d are presented in Table 3.
Thin Layer Chromatography (TLC) was carried out using Merck Kieselgel 60F254 sheets. Spots were visualized by treatment with 5% ethanolic phosphomolybdic acid solution and heating. Preparative chromatographic separations were performed using column chromatography on Merck Kieselgel 60 (63-200 µm). Melting points were determined by hot plate method and are uncorrected. Optical rotations were determined on a Perkin-Elmer 201 polarimeter and [α]D values are given in units of 10-1 deg cm2 g-1.
1.2. Reagents and solvents
Furan-2-carbaldehyde, anilines, triphenyl-λ5-phosphanilidene acetic acid ethyl ester, all inorganic reagents and solvents were products of Aldrich or Fluka. All solvents were purified and dried by standard methods as required.
2. Recombinant PAL
The wild-type-phenylalanine ammonia-lyases were overexpressed in E. coli and purified as described, first according to Schuster and Rétey14 and later using the improved method of Baedeker and Schulz.15 Kinetic measurements and enzymatic reactions were performed at 30 oC.
3. Kinetic measurements
3.1. Determination of Vmax and Km values for substrate amino acids
The kinetic constants were determined using a 1 mL cuvette, by recording for 5 min in an interval of 1 min the UV absorption of the produced acrylates at wavelengths where the corresponding amino acids do not show absorption. After incubation of the enzyme (0.25 µg) in 0.1 M Tris-HCl buffer at pH 8.8 and 30 °C for 5 min various amounts of amino acids were added, varying the substrate concentration of the enzymatic assays in range 20-100 μM.
4. Enzymatic synthesis of enantiopure amino acids
4.1. Kinetic resolution of racemic amino acids catalyzed by PAL
Into the solution of one of the amino acids rac-1a-d (0.5 mmol) in Tris-HCl buffer (0.1 M, pH = 8.8, 70 mL), PAL was added and the reaction mixture was stirred under argon at 30 °C. The progress of the reaction was monitored by HPLC. When the L-enantiomer was completely consumed, the pH of the solution was adjusted to 1.5 with 5% HCl, then the solution was heated to 90 °C for 10 min, cooled to room temperature, filtered and applied to a Dowex 50X8 cation exchange resin column. The elution of the pure D-enantiomer of amino acids occurred with 2M ammonia solution. Reaction times, quantity of the enzyme in each chase and the yields of the products are presented in Table 2.
4.2. Synthesis of L-amino acids from cinnamates by PAL mediated enantioselective ammonia addition
Into the solution of one of the cinnamates (E)-2a-d (0.5 mmol) in half-concentrated ammonia solution (50 mL), CO2 was bubbled until the pH of the solution was adjusted to 10.2. After the addition of PAL the reaction mixture was stirred under argon at 30 °C. The progress of the reaction was monitored by HPLC and when the formation of the l-amino acids was stopped, the reaction mixture was degassed under reduced pressure, the pH was adjusted to 1.5 with 5% HCl, filtered, then the solution was heated to 90 °C for 10 min, cooled to room temperature, filtered again, and applied to a Dowex 50X8 cation exchange resin column. Elution of the pure l-enantiomer of the amino acids occurred with 2 m ammonia solution. Reaction times, quantity of the enzyme in each case and the yields of the products are presented in Table 2.
5. Chemical synthesis of the racemic 5-phenylfuran-2-ylalanines
Into a stirred solution of 5-phenylfuran-2-yl aldehydes (4 g) in MeOH (40 mL) NaBH4 was added in small portions at room temperature, until the entire amount of the aldehyde was transformed. The progress of the reactions was monitored by thin layer chromatography, using CH2Cl2 as eluent. There after the reaction mixtures were treated with 1 m HCl (4 mL) and the MeOH was removed in vacuo. The crude products were treated with a mixture of CH2Cl2:H2O, 1:1 (v/v), the organic layers were separated, dried over anhydrous MgSO4, the CH2Cl2 was removed in vacuo. The crude products were purified by column chromatography, using CH2Cl2: acetone, 9:1 (v/v) as eluent. The pure compounds were immediately used in the next step.
A stock solution (1.5 M) was prepared by making up volume of a viscous clear solution of thionyl chloride (5.46 mL, 0.075 mol) and benzotriazole (8.93 g, 0.075 mol) with dry CH2Cl2 up to 50 mL and transferred to 50 mL graduated burette. The reaction was started by adding the stock solution (1.25 mmol equivalent) intermittently from the burette to a stirred solution of the previously obtained 5-phenylfuran-2-ylmethanols (1 mmol) in dry CH2Cl2 (20 mL). Before the addition is complete, benzotriazole hydrochloride started to precipitate. Reaction mixtures were stirred further for 5-10 min. At the end the solid was filtered off and washed with dry CH2Cl2 (2×25 mL). The filtrates were washed with 10% HCl (25 mL), water (25 mL), followed by 2% aqueous NaOH solution (25 mL). The organic layers were separated and dried over anhydrous MgSO4. The crude products were purified by distillation under reduced pressure and the distillates were immediately used in the next step of the synthesis.
55% NaH oil suspension (0.85 g, 19.5 mmol) was added into dry DMF (20 mL) and it was vigorously stirred for 30 min. under argon at room temperature. Subsequently 2-acetylaminomalonic acid Et2O (20 mmol) was added to the suspension and then the reaction mixture was stirred for 30 min, chloromethylene derivatives were added, then the mixtures were stirred for 3 h at room temperature and then for 4 h at 60 ºC. After cooling the reaction products were poured into a water-ice mixture (100 g). The resulting precipitates were filtered off, dried in vacuo at room temperature and used without purification in the next step.
The N-substituted-malonic acid ester (0.75 g) dissolved in MeOH (2.5 mL) were added in one portion into 10% aqueous NaOH solution (2.5 mL), then the reaction mixture was stirred 20 h at room temperature. Subsequently, the MeOH was removed in vacuo, the reaction mixture was extracted with CH2Cl2 (2×25 mL) and the pH of the solution was adjusted to 1 with conc. HCl at -10 ºC, resulting a white precipitate, which was filtered and dried in vacuo at room temperature. The formed dicarboxylic acids were suspended in toluene (10 mL) and were refluxed for 2 h. The solvent was removed in vacuo, affording the pure products.
Into the solution of N-acetyl amino acid derivatives (1 g) in dioxane (15 mL) concentrated HCl (1 mL) was added and the mixtures were refluxed for 4 h, cooled to room temperature, obtaining the products as a white precipitate, which were filtered, dried and finally washed with Et2O. The precipitate was resuspended in water and the pH was adjusted to 5.6, stirred for 30 min. filtered and dried at room temperature affording the pure racemic 5-phenylfuran-2-yl-alanines (rac-1a-d).
rac-2-Amino-3-(5-phenylfuran-2-yl)propanoic acid (rac-1a).
Yield 61%, mp 170 °C. 1H-NMR: (300 MHz, DMSO-d6): δ = 2.85(2H, s), 3.24 (2H, d); 4.14 (1H, t); 6.46 (1H, d); 6.89 (1H, d); 7.52 (2H, dd); 7.65 (1H, dd); 7.96 (2H, d); 13C-NMR (75 MHz, DMSO-d6): δ = 36.03; 47.55; 106.61; 111.05; 127.92; 128.78; 133.31; 136.35; 170.14; 198.39; 205.65; ESI+-MS: m/z: 250.1087 [M+H2O+H+].
rac-2-Amino-3-(5-(4-bromophenyl)furan-2-yl)propanoic acid (rac-1b). Yield 68%, mp 175 °C. 1H-NMR: (300 MHz, DMSO-d6): δ = 2.83 (2H, s), 3.22 (2H, d); 4.14 (1H, t); 6.46 (1H, d); 6.87 (1H, d); 7.75 (2H, d); 7.89 (2H,d);13C-NMR (75 MHz, DMSO-d6): δ = 35.95; 47.55; 107.55; 111.29; 125.35; 127.38; 131.71; 135.85; 170.19; 197.68; 205.63; ESI+-MS: m/z (abundance): 328.0183 (100%), 330.0176 (99.12%) [M+H2O+H+].
rac-2-Amino-3-(5-(4-chlorophenyl)furan-2-yl)propanoic acid (rac-1c) Yield 60%, mp 180 °C. 1H-NMR: (300 MHz, DMSO-d6): δ = 2,87 (2H, s), 3.23 (2H, d); 4.13 (1H, t); 6.65 (1H, d); 6.95 (1H, d); 7.61 (2H, d); 7.79 (2H, d); 13C-NMR (75 MHz, DMSO-d6): δ = 35.99; 47.53; 111.23; 125.08; 128.88; 129.88; 135,03; 138.16; 170.13; 197.46; 205.56; ESI+-MS: m/z (abundance): 284.0683 (100%), 286.0652 (33.17%) [M+H2O+H+]; ESI--MS: m/z (abundance): 282.0521 (100%), 284.508 (35.66%) [M+OH-].
rac-2-Amino-3-(5-(2-chlorophenyl)furanyl)propanoic acid (rac-1d). Yield 65%, mp 210 °C. 1H-NMR: (300 MHz, DMSO-d6): δ = 2.85(2H, s), 3.35 (2H, d); 4.20 (1H, d); 6.46 (1H, d); 7.04 (1H, d); 7.29 (1H, t); 7.40 (1H, t); 7.49 (1H, d); 7.81 (1H, d); 13C-NMR (75 MHz, DMSO-d6): δ = 28.60; 51.01; 111.23; 112.07; 127.55; 127.94; 128.38; 128.65; 128.73; 130.72; 148.89; 149.07; 170.01; ESI--MS: m/z (abundance): 266.0579 (100%), 268.0549 (33.02%) [M+H+].
6. Chemical synthesis of the (E)-5-phenylfuran-2-ylacrylates
To a stirred solution of 5-phenyl-furan-2-ylcarbaldehydes (10 mmol) in dry toluene (20 mL) triphenyl-λ5-phosphanilidene acetic acid ethyl ester (4.35 g, 12.5 mmol) was added. The reaction mixtures were refluxed for 2 h, and then the toluene was removed in vacuo. The precipitate was taken up in a minimal amount of CH2Cl2 and the crude product was purified with column chromatography on silica gel using CH2Cl2 as eluent. In each case 3-5% (Z)-isomer was also formed.
The isolated (E)-acrylic acid esters (0.5 g) were suspended in 10% KOH (10 mL) and the mixtures were refluxed for 4 h. After that the reaction mixtures were cooled to 0 °C and the pH was adjusted to 1 using conc. HCl. The formed precipitate was filtered, washed with water (5 mL), dried and purified by recrystallization from EtOH.
(E)-3-(5-Phenylfuran-2-yl)acrylic acid ((E)-2a) Yield 91%, mp 173-174 °C. 1H-NMR: (300 MHz, DMSO-d6): δ = 6.32 (d, J = 15.5 Hz, 1H); 6.46 (d, J = 3.2 Hz, 1H); 6.89 (d, J = 3.2 Hz, 1H); 7.42-81 (m, 4H); 7.96 (d, J = 7.8 Hz, 2H); 13C-NMR: (75 MHz, DMSO-d6): δ = 105.1; 110.3; 123.5; 123.6; 127.8; 128.5; 130.3; 131.0; 150.1; 152.4; 170.14; ESI+-MS: m/z: 233.07534 [M+H2O+H+].
(E)-3-(5-(4-Bromophenyl)furan-2-yl)acrylic acid ((E)-2b) Yield 90%, mp 181-181.5 °C. 1H-NMR: (300 MHz, DMSO-d6): δ = 6.34 (d, J = 15.5 Hz, 1H); 6.40 (d, J = 3.8 Hz, 1H); 6.93 (d, J = 3.8 Hz, 1H); 7.39 (d, J = 15.5 Hz, 1H); 7.60 (d, J = 8.6 Hz, 2H); 7.80 (d, J = 8.6 Hz, 2H); 13C-NMR: (75 MHz, DMSO-d6): δ = 106.3; 109.8; 120.0; 123.7; 126.1; 130.4; 131.7; 132.1; 149.0; 150.8; 170.3; ESI+-MS: m/z (abundance): 310.9834 (100%), 312.9863 (98.7%) [M+H2O+H+].
(E)-3-(5-(4-Chlorophenyl)furan-2-yl)acrylic acid ((E)-2c) Yield 91%, mp 168-169 °C. 1H-NMR: (300 MHz, DMSO-d6): δ = 6.32 (d, J = 15.6 Hz, 1H); 6.42 (d, J = 3.3 Hz, 1H); 6.89 (d, J = 3.3 Hz, 1H); 7.40 (d, J = 15.6 Hz, 1H); 7.72 (d, J = 7.8 Hz, 2H); 7.86 (d, J = 7.8 Hz, 2H); 13C-NMR (75 MHz, DMSO-d6): δ = 108.2; 111.1; 122.6; 125.8; 128.8; 129.8; 130.9; 134,6; 150.1; 152.7; 169.9; ESI+-MS: m/z (abundance): 249.0278 (100%), 251.0169 (32.31%) [M+H+].
(E)-3-(5-(2-Chlorophenyl)furan-2-yl)acrylic acid ((E)-2d) Yield 92%, mp 169-170 °C. 1H-NMR: (300 MHz, DMSO- d6): δ = 6.30 (d, J = 15.5 Hz, 1H); 6.47 (d, J = 2.8 Hz, 1H); 7.07 (d, J = 3.2 Hz, 1H); 7.31 (t, J = 7.7 Hz, 1H); 7.34 (d, J = 15.5 Hz, 1H); 7.42 (t, J = 7.7 Hz, 1H); 7.53 (d, J = 7.7 Hz, 1H); 7.84 (d, J = 7.9 Hz, 1H); 13C-NMR: (75 MHz, DMSO-d6): δ = 110.7; 111.3; 124.5; 128.3; 128.7; 129.2; 129.7; 130.1; 131.7; 132.3; 148.3; 149.2; 170.5; ESI+-MS: m/z (abundance): 267.0462 (100%), 269.0349 (33.45%) [M+H2O+H+].
ACKNOWLEDGEMENTS
We thank Professor Georg E. Schulz and Dr. Mathias Baedeker for a plasmid of the modified PAL gene. Financial support by the Deutsche Forschungsgemeinschaft, the European Commission and the Fonds der Chemischen Industrie is gratefully acknowledged. The financial support to C.P. from the Romanian Ministry of Education and Research (CNCSIS No. 527/2461) is gratefully acknowledged.
References
1. L. Poppe and J. Rétey, Angew. Chem., 2005, 117, 3734; CrossRef L. Poppe and J. Rétey, Angew. Chem. Int. Ed., 2005, 44, 3668. CrossRef
2. T. F. Schwede, J. Rétey, and G. E. Schulz, Biochemistry, 1999, 38, 5355. CrossRef
3. F. G. Bordwell and Y. Zhao, J. Org. Chem., 1995, 60, 6348. CrossRef
4. M. Rettig, A. Sigrist, and J. Rétey, Helv. Chim. Acta, 2000, 83, 2246. CrossRef
5. B. Schuster and J. Rétey, Proc. Natl. Acad. Sci. USA, 1995, 92, 8433. CrossRef
6. S. J. Benkovic, G. G. Hammes, and S. Hammes-Schiffer, Biochemistry, 2008, 47, 3317. CrossRef
7. H. Engelkamp, N. S. Hatzakis, J. Hofkens, F. C. De Schryver, R. J. M. Nolte, and A. E. Rowan, Chem. Commun., 2006, 935. CrossRef
8. S. Bartsch and U. T. Bornscheuer, Angew. Chem., 2009, 121, 3412; CrossRef S. Bartsch and U. T. Bornscheuer, Angew. Chem. Int. Ed., 2009, 48, 3362. CrossRef
9. a) S. Yamada, K. Nabe, N. Izuo, K. Nakamichi, and I. Chibata, Appl. Environment. Microb., 1981, 42, 773; b) C. T. Evans, K. Hanna, C. Payne, D. Conrad, and M. Misawa, Enzyme Microb. Technol., 1987, 9, 417; CrossRef c) M. Yanaka, D. Ura, A. Takahashi, and N. Fukuhara (Mitsui Toatsu Chemicals), JP 06,113,870 (1994) [Chem. Abstr., 1994, 121, 155941 y].
10. a) A. Gloge, B. Langer, L. Poppe, and J. Rétey, Arch. Biochem. Biophys., 1998, 359, 1; CrossRef b) A. Gloge, J. Zoń, Á. Kővári, L. Poppe, and J. Rétey, Chem. Eur. J., 2000, 6, 3386; CrossRef c) W. Liu, (Great Lakes Chemical Co.), US P 5,981,239, 1999.
11. H. Meerwein, E. Buchner, and K. J. Emster, J. Prakt. Chem., 1939, 152, 237. CrossRef
12. a) C. Paizs, A. Katona, and J. Rétey, Chem. Eur. J., 2006, 12, 2739; CrossRef b) C. Paizs, A. Katona, and J. Rétey, Eur. J. Org. Chem., 2006, 1113. CrossRef
13. G. Renard, J. C. Guilleux, C. Bore, V. Malta-Valette, and D. A. Lerner, Biotechnol. Lett., 1992, 14, 673. CrossRef
14. B. Schuster and J. Rétey, FEBS Lett. 1994, 349, 252. CrossRef
15. M. Baedeker and G. E. Schulz, FEBS Lett., 1999, 457, 57. CrossRef