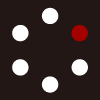
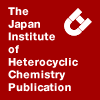
HETEROCYCLES
An International Journal for Reviews and Communications in Heterocyclic ChemistryWeb Edition ISSN: 1881-0942
Published online by The Japan Institute of Heterocyclic Chemistry
e-Journal
Full Text HTML
Received, 27th April, 2010, Accepted, 7th June, 2010, Published online, 8th June, 2010.
DOI: 10.3987/COM-10-S(E)24
■ A Synthetic Approach to Anhydroketopyranoses Having a 6,8-Dioxabicyclo[3.2.1]octane Structure from Ketopyranoses
Takashi Yamanoi,* Kazuhide Matsumura, Sho Matsuda, and Yoshiki Oda
The Noguchi Institute, 1-8-1, Kaga, Itabashi-ku, Tokyo 173-0003, Japan
Abstract
We investigated a synthetic approach to the anhydroketopyranoses having a 6,8-dioxabicyclo[3.2.1]octane structure from D-glucopyranose derivatives. The paper describes the nucleophilic addition of the organometallic reagents RLi or RMgX to the glucono-1,5-lactone derivatives to produce the ketopyranose derivatives having a glucose backbone and their intramolecular cyclization into the desired structural anhydroketopyranoses.INTRODUCTION
The anhydroketopyranoses having a 6,8-dioxabicyclo[3.2.1]octane structure are found in biologically important natural products such as Sedum spectabile,1 Smallanthus sonchifolius,2 and Coriaria japonica.3 Some of them have been prepared by the acid-catalyzed conversions of aldoses4 and by the intramolecular cyclization of C-glycopyranosides via the intramolecular hydrogen abstraction reaction using radical species.5 However, there is a limit to the structure of the anhydroketopyranoses that these methods can synthesize. It is important to develop convenient and useful methods for producing the various types of anhydroketopyranoses.
The anhydroketopyranoses having a 6,8-dioxabicyclo[3.2.1]octane can be structurally regarded as intramolecular O-ketopyranosides. Therefore, we investigated the anydroketopyranosylation based on the intramolecular O-ketosidation to synthesize the anhydroketopyranoses from ketopyranoses. The ketopyranoses used in the reaction were the D-glucopyranose derivatives whose anomeric carbons were bound to functional groups via a carbon–carbon linkage, i.e., ‘1-C-functionalized glucopyranoses’. Our study showed that these ketopyranoses could be converted into anhydroketopyranoses using 5 mol% trifluoromethanesulfonic acid (TfOH).6 We also applied this anydroketopyranosylation to the synthesis of a naturally occurring anhydroketopyranose found in Coriaria japonica.7,8
The anhydroketopyranosylation method requires the appropriately protected ketopyranose derivatives having a C-6 hydroxy group. The addition reaction of the organometallic reagents RLi or RMgX to a protected sugarlactone derivative is a generally convenient technique for synthesizing various ketopyranoses.9 Prior to the anhydroketopyranosylation research, we had investigated the preparation of several appropriate ketopyranose derivatives having a D-glucose backbone from a 6-O-acetyl-D-glucono-1,5-lactone derivative according to the protocol. This investigation led us to the finding that the nucleophilic susceptibility of the two carbonyl carbon atoms, at C-1 and within the C-6 acetyl group of the lactones, remarkably varied based on the RLi or RMgX species.
This full paper comprehensively describes a synthetic approach to the anhydroketopyranoses having a 6,8-dioxabicyclo[3.2.1]octane structure from the ketopyranose derivatives. It consists of (1) the nucleophilic addition of the organometallic reagents, RLi or RMgX, to the 6-O-acetyl-D-glucono-1,5-lactone to produce the ketopyranose derivatives with a D-glucose backbone, and (2) the anhydroketopyranosylation to convert the ketopyranose derivatives into the desired structural anhydroketopyranoses based on the intramolecular O-ketosidation. The former part describes in detail our interesting findings about the nucleophilic reaction behaviors of the organometallic reagents to the lactone derivative, which is useful information for preparing the ketopyranose derivatives.
RESULTS AND DISCUSSION
1. Nucleophilic addition of RLi or RMgX to 6-O-acetyl-D-glycono-1,5-lactone
We investigated the nucleophilic addition to the 6-O-acetyl-2,3,4-tri-O-benzyl-D-glucono-1,5-lactone (1)10 obtained by the oxidation of 6-O-acetyl-2,3,4-tri-O-benzyl-D-glucopyranose using DMSO-Ac2O, as shown in Scheme 1. The main purpose of this study was to chemoselectively introduce the functional groups into the carbonyl carbon atom at the C-1 of 1 to produce the corresponding ketopyranose derivatives having a D-glucose backbone. As the nucleophiles of the organometallic reagents, methyllithium (MeLi), allylmagnesium bromide (AllMgBr), n-butyllithium (n-BuLi), phenylmagnesium bromide (PhMgBr), and benzylmagnesium chloride (PhCH2MgCl) were used. The addition reaction of the nucleophile (2.4 equiv.) to 1 was carried out in dry THF at -78 ºC. These results are shown in Table 1. The reaction of 1 with MeLi produced the two products, 6-O-acetyl-2,3,4-tri-O-benzyl-1-C- methyl-D-glucopyranose (2a) and 2,3,4-tri-O-benzyl-1-C-methyl-D-glucopyranose (3a) in 14% and 64% yields, respectively.
The reaction of 1 with AllMgBr and n-BuLi similarly produced the products 6-O-acetyl-1-C-allyl-2,3,4-tri-O-benzyl-D-glucopyranose (2b) and 1-C-allyl-2,3,4-tri-O-benzyl-D-glucopyranose (3b) in 11% and 68% yields, and 6-O-acetyl-2,3,4-tri-O-benzyl-1-C-n-butyl-D-glucopyranose (2c) and 2,3,4-tri-O-benzyl-1-C-n-butyl-D-glucopyranose (3c) in 64% and 12% yields, respectively. In addition, the addition reaction of MeLi (1.2 equiv.) to 1 gave 2a and 3a in 63% and 10% yields, respectively.
These results showed that the nucleophiles attacked both carbonyl carbon atoms, C-1 and within the C-6 acetyl group, and that the bulky nucleophile tended not to attack the carbonyl carbon atom within the C-6 acetyl group. It is also noteworthy that there was no case when only the C-6 acetyl group was selectively deprotected. Based on these observations, the carbonyl carbon atom C-1 was more reactive to these nucleophiles than the carbonyl carbon atom within the C-6 acetyl group.
The differences in susceptibility to nucleophilic attack between the two carbonyl carbon atoms, C-1 and within the C-6 acetyl group, can be explained by the stereoelectronic effect shown in Figure 1.11 The C-6 acetyl group assumes the Z-conformation and is stabilized by the n-σ*C-O interaction between the lone pair on the OR’ oxygen and the σ* antibonding orbital of the C=O group. This interaction never takes place in lactones having the E-conformation. Thus, the carbonyl carbon atom C-1 will indicate the higher reactivity than the carbonyl carbon atom within the C-6 acetyl group.
Furthermore, the nucleophilic reagents, PhMgBr and PhCH2MgCl, were utilized. The addition of PhMgBr or PhCH2MgCl to 1 gave 6-O-acetyl-2,3,4-tri-O-benzyl-1-C-phenyl-D-glucopyranose (2d) and 6-O-acetyl-1-C-benzyl-2,3,4-tri-O-benzyl-D-glucopyranose (2e) in 89% and 82% yields, respectively, with almost no production of the deacetylated D-glucopyranoses (3d and 3e). When we attempted the deacetylation reaction using methyl 6-O-acetyl-2,3,4-tri-O-benzyl-α-D-glucopyranoside (4) by PhMgBr or PhCH2MgCl, its C-6 acetyl group was not removed under the given reaction conditions using the nucleophile (1.2 equiv.) in dry THF at -78 ºC. This indicates that the PhMgBr and PhCH2MgCl nucleophiles lack the potential nucleophilicity to attack the carbonyl carbon atom within the C-6 acetyl group of 4. It became evident that the PhMgBr and PhCH2MgCl nucleophiles chemoselectively attacked the C-1 carbonyl carbon atom of 1 to produce the ketopyranose derivatives (2d and 2e). As already mentioned, we clarified the nucleophilic susceptibility of the two carbonyl carbon atoms, C-1 and within the C-6 acetyl group, of 1 to the RLi or RMgX species.
The C-6 acetyl group of 2a-e was quantitatively removed using NaOMe in MeOH to produce the desired ketopyranose derivatives 3a-e.
2. Conversion of ketopyranose derivatives into anhydroketopyranoses
We first investigated the conversion of 3a into the corresponding anhydroketopyranose (5a) having a 6,8-dioxabicyclo[3.2.1]octane structure by the anhydroketopyranosylation based on the intramolecular O-ketosidation, as shown in Scheme 2. Brφnsted acids were considered to be the useful activating reagents for this dehydration condensation type reaction as we previously reported for the intermolecular O-ketosidations using the ketopyranose derivatives.12,13 Each reaction using 20 mol% of camphorsulfonic acid, Tf2NH, and TfOH as the Brφnsted acids in MeCN at 0 ºC gave the desired 5a in 59%, 82%, and 84% yields, respectively. Tf2NH and TfOH were very efficient for this reaction. The amount of TfOH was then varied. The reactions using only 1 or 0.5 mol% of TfOH proceeded to give 5a in 86% and 68% yields, respectively. The maximum yield of 93% was attained for the reaction using 5 mol% of TfOH.
In order to investigate the effect of the methyl group at C-1 of 3a on this anhydroketopyranosylation, we attempted the conversion of 2,3,4-tri-O-benzyl-D-glucopyranose (6), which had no methyl group at C-1, into 1,6-anhydro-2,3,4-tri-O-benzyl-D-glucopyranose (7). The production of 7 was scarcely observed by TLC under similar reaction conditions using 20 mol% TfOH for 2 h, and the unreacted 6 was quantitatively recovered from the reaction mixture. This observation indicated that the presence of the methyl group at the anomeric position of 3a drastically increased the reactivity of the anhydroketopyranosylation using 3a. We inferred that the presence of the anomeric methyl group of 3a would promote not only the generation of the oxocarbenium cation intermediate from 3a, but also the conformation flip of the pyranosyl ring from 4C1 into 1C4 because of its equatorial orientation in the anhydroketopyranose form.
We next attempted the anhydroketopyranosylation of 3b-e in order to demonstrate the synthesis of various kinds of anhydroketopyranoses (5b-e). The reactions using 3b and 3c in MeCN at 0 ºC for 2h in the presence of 5 mol% TfOH afforded the desired anhydroketopyranoses (5b and 5c) in the excellent yields of 90% and 95%, respectively. The 1C4 conformational 5d and 5e were similarly obtained from 3d and 3e in 78% and 70% yields, respectively. Interestingly, in these two reactions, the twist-boat conformational isomers 5d-t and 5e-t were also formed in 14% and 26 % yields, respectively.14 Therefore, the total yields of these reactions reached 92% and 96%, respectively. These results showed that the difference in the 1-C-functional groups of 3a-e had almost no influence on the anhydroketopyranosylation yields, although the benzyl and phenyl groups of 3d and 3e influenced the ring conformations of the anhydroketopyranoses.
Furthermore, the reaction using 2,3,4-tri-O-benzyl-6-O-t-butyldimethylsilyl-D-glucopyranose (8) also afforded 5a in 80% yield. In this reaction, TfOH not only promoted the anhydroketopyranosylation, but also removed the TBS group of 8, which is a great advantage of this anhydroketopyranosylation. In none of the above reactions did we observe the production of oligosaccharides by intermolecular O-ketosidation. These results are summarized in Table 2.
CONCLUSIONS
This paper described the synthetic approach to several anhydroketopyranoses having a 6,8-dioxabicyclo[3.2.1]octane structure from the ketopyranose derivatives. We clarified the nucleophilic reaction behaviors of RLi or RMgX to the D-glucono-1,5-lactone to produce the ketopyranose derivatives and the anhydroketopyranosylation characteristics to convert them into the desired structural anhydroketopyranoses.
EXPERIMENTAL
1H NMR (600 MHz) and 13C NMR (150 MHz) spectra were recorded on a JEOL ECA-600 spectrometer in CDCl3 using TMS as an internal standard. Optical rotations were recorded on a JASCO DIP-360 digital polarimeter. HRMS were obtained on a Mariner spectrometer (PerSeptive Biosystems Inc.). Preparative TLC was performed using Merck silica gel 60GF254. Column chromatography was conducted using silica gel 60 N (40~50 µm, Kanto Chemical Co., INC.). All anhydrous solvents were purified according to standard methods.
6-O-Acetyl-2,3,4,-tri-O-benzyl-D-glucono-1,5-lactone (1)10: To a solution of 6-O-acetyl-2,3,4,-tri-O-benzyl-D-glucopyranose (3.5 g, 7.2 mmol) in DMSO (20 mL) was added Ac2O (13 mL). After stirring overnight, the cool water was added to the reaction mixture. The reaction mixture was extracted with AcOEt (200 mL), and the organic layer was washed with water and brine. After the organic layer was dried over anhydrous Na2SO4, the solvent was filtered and evaporated under reduced pressure. The crude product was purified by preparative flash silica-gel column chromatography (1:15 AcOEt-hexane) to give 1 (3.2 g, 92%) as an amorphous solid. Rf 0.48 (1:2 AcOEt-hexane); [α]D25 +102◦ (c 3.9, CHCl3); 1H NMR (CDCl3): δ 2.00 (3H, s, CH3), 3.75 (1H, dd, J = 5.5 Hz, J = 9.6 Hz, H-4), 3.95 (1H, t, J = 5.5 Hz, H-3), 4.13 (1H, d, J = 5.5 Hz, H-2), 4.24 (1H, dd, J = 4.1 Hz, J = 12.4 Hz, H-6a), 4.33 (1H, dd, J = 2.7 Hz, J = 12.4 Hz, H-6b), 4.43 (1H, d, J = 11.7 Hz, CH2Ph), 4.44 (1H, d, J = 11.7 Hz, CH2Ph), 4.63-4.66 (4H, m, H-5, CH2Ph), 4.85 (1H, d, J = 11.7 Hz, CH2Ph); 13C NMR (CDCl3): δ 20.7 (CH3), 62.3 (C-6), 73.0 (CH2Ph), 73.28 (CH2Ph), 73.29 (CH2Ph), 75.5 (C-4 or C-5), 75.6 (C-4 or C-5), 76.7 (C-2), 80.8 (C-3), 168.3 (C-1), 170.4 (COCH3); HRMS(ESI): m/z calcd for 513.1884 (C29H30O7·Na+); Found: 513.1902.
6-O-Acetyl-2,3,4,-tri-O-benzyl-1-C-methyl-D-glucopyranose (2a) and 2,3,4,-tri-O-benzyl-1-C- methyl-D-glucopyranose (3a): To a solution of 1 (1 g, 2 mmol) in THF (5 mL) was added dropwise a 1.14 M Et2O solution of MeLi (4.3 mL, 4.9 mmol) at -78 oC under an Ar atmosphere. After the resulting mixture was stirred at -78 oC for 1 h, the reaction was then quenched by the addition of water (5 mL). The reaction mixture was extracted with AcOEt (30 mL), and the organic layer was washed with water and brine. After the organic layer was dried over anhydrous Na2SO4, the solvent was filtered and evaporated under reduced pressure. The crude product was purified by preparative flash silica-gel column chromatography (1:10 → 1:4 → 1:2 AcOEt-hexane) to give 2a (0.14 g, 14%) as an amorphous solid and 3a (0.61 g, 64%) as an amorphous solid. 2a: Rf 0.23 (1:2 AcOEt-hexane); 1H NMR (CDCl3): δ 1.39 (3H, s, H-1’), 2.03 (3H, s, COCH3), 3.35 (1H, d, J = 9.6 Hz, H-2), 3.54 (1H, t, J = 9.6 Hz, H-4), 4.00 (1H, t, J = 9.6 Hz, H-3), 4.05-4.07 (1H, m, H-5), 4.23-4.28 (2H, m, H-6), 4.58 (1H, d, J = 11.0 Hz, CH2Ph), 4.71 (1H, d, J = 11.0 Hz, CH2Ph), 4.87 (1H, d, J = 11.0 Hz, CH2Ph), 4.91 (2H, s, CH2Ph), 4.94 (1H, d, J = 11.0 Hz, CH2Ph); 13C NMR (CDCl3): δ 20.9 (COCH3), 26.4 (C-1’), 63.3 (C-6), 69.7 (C-5), 74.9 (CH2Ph), 75.6 (CH2Ph), 75.8 (CH2Ph), 78.0 (C-4), 83.2 (C-2), 83.5 (C-3), 97.3 (C-1), 170.9 (C=O); HRMS(ESI): m/z calcd for 529.2197 (C30H34O7·Na+); Found: 529.2242. 3a: Rf 0.09 (1:2 AcOEt-hexane); 1H NMR (CDCl3): δ 1.30 (3H, s, H-1’), 3.26 (1H, d, J = 9.3 Hz, H-2), 3.52 (1H, t, J = 9.5 Hz, H-4), 3.64 (1H, dd, J = 4.2 Hz, J = 12.0 Hz, H-6a), 3.74 (1H, dd, J = 2.7 Hz, J =12.0 Hz, H-6b), 3.81-3.85 (1H, m, H-5), 3.91 (1H, t, J = 9.3 Hz, H-3), 4.61 (1H, d, J = 11.0 Hz, CH2Ph), 4.64 (1H, d, J = 11.2 Hz, CH2Ph), 4.80-4.88 (4H, m, CH2Ph); 13C NMR (CDCl3): δ 26.7 (C-1’), 61.9 (C-6), 72.0 (C-5), 74.9 (CH2Ph), 75.6 (CH2Ph), 75.7 (CH2Ph), 78.0 (C-4), 83.2 (C-2), 83.4 (C-3), 97.3 (C-1); HRMS (ESI): m/z calcd for 487.2091 (C28H32O6·Na+); Found: 487.2138. The ordinary treatment of 2a using NaOMe in MeOH quantitatively afforded 3a.
6-O-Acetyl-1-C-allyl-2,3,4,-tri-O-benzyl-D-glucopyranose (2b) and 1-C-allyl-2,3,4,-tri-O-benzyl-D- glucopyranose (3b): The above same procedure using a 1.0 M diethyl ether solution of AllMgBr (0.75 mL, 0.750 mmol) and 1 (153 mg, 0.31 mmol) in THF (3 mL) gave 2b (PTLC; 1:2 AcOEt-hexane, 19 mg, 11%) as an amorphous solid and 3b (115 mg, 68%) as an amorphous solid. 2b: Rf 0.41 (1:2 AcOEt-hexane); 1H NMR (CDCl3): δ 1.97 (3H, s, CH3), 2.30 (1H, dd, J = 6.8 Hz, J = 13.7 Hz, H-1’a), 2.38 (1H, dd, J = 8.0 Hz, J = 13.7 Hz, H-1’b), 3.35 (1H, d, J = 9.5 Hz, H-2), 3.46 (1H, t, J = 9.5 Hz, H-4), 3.91-3.97 (1H, m, H-5), 3.98 (1H, t, J = 9.3 Hz, H-3), 4.16 (1H, dd, J = 4.4 Hz, J = 12.0 Hz, H-6a), 4.21 (1H, dd, J = 1.9 Hz, J = 11.7 Hz, H-6b), 4.52 (1H, d, J = 11.0 Hz, CH2Ph), 4.63 (1H, d, J = 11.0 Hz, CH2Ph), 4.88-4.92 (4H, m, CH2Ph), 5.04-5.15 (2H, m, H-3’), 5.66-5.80 (1H, m, H-2’); 13C NMR (CDCl3): δ 20.9 (CH3), 42.6 (C-1’), 63.1 (C-6), 69.6 (C-5), 74.9 (CH2Ph), 75.4 (CH2Ph), 75.6 (CH2Ph), 78.1 (C-4), 81.4 (C-2), 83.6 (C-3), 97.5 (C-1), 120.3 (C-3’), 131.9 (C-2’), 170.8 (C=O); HRMS (ESI): m/z calcd for 555.2353 (C32H36O7·Na+); Found: 555.2371. 3b: Rf 0.16 (1:2 AcOEt-hexane); 1H NMR (CDCl3): δ 2.38 (1H, dd, J = 6.9 Hz, J = 13.7 Hz, H-1’a), 2.46 (1H, dd, J = 7.6 Hz, J = 13.7 Hz, H-1’b), 3.41 (1H, d, J = 8.9 Hz, H-2), 3.58 (1H, t, J = 9.6 Hz, H-4), 3.71-3.75 (1H, m, H-6a), 3.80 (1H, dd, J = 2.1 Hz, J = 11.7 Hz, H-6b), 3.86-3.89 (1H, m, H-5), 4.04 (1H, t, J = 8.9 Hz, H-3), 4.68-4.70 (2H, m, CH2Ph), 4.87-4.90 (2H, m, CH2Ph), 4.92-4.95 (2H, m, CH2Ph), 5.11-5.21 (2H, m, H-3’), 5.81 (1H, m, H-2’); 13C NMR (CDCl3): δ 42.7 (C-1’), 61.8 (C-6), 71.8 (C-5), 75.0 (CH2Ph), 75.4 (CH2Ph), 75.6 (CH2Ph), 78.0 (C-4), 81.4 (C-2), 83.5 (C-3), 97.6 (C-1), 120.4 (C-3’), 131.8 (C-2’); HRMS (ESI): m/z calcd for 513.2248 (C30H34O6·Na+); Found: 513.2295. The ordinary treatment of 2b using NaOMe in MeOH quantitatively afforded 3b.
6-O-Acetyl-2,3,4,-tri-O-benzyl-1-C-butyl-D-glucopyranose (2c) and 2,3,4,-tri-O-benzyl-1-C-butyl-D- glucopyranose (3c): The above same procedure using a 1.59 M n-hexane solution of n-BuLi (0.39 mL, 0.61 mmol) and 1 (126 mg, 0.26 mmol) in THF (3 mL) gave 2c (PTLC; 1:19 AcOEt-toluene, 90 mg, 64%) as a colorless oil and 3c (16 mg, 12%) as an amorphous solid. 2c: Rf 0.36 (1:2 AcOEt-hexane); 1H NMR (CDCl3): δ 0.85 (3H, t, J = 6.9 Hz, H-4’), 1.19-1.35 (4H, m, H-2’, H-3’), 1.61-1.64 (2H, m, H-1’), 2.03 (3H, s, COCH3), 3.42 (1H, d, J = 9.6 Hz, H-2), 3.51 (1H, t, J = 9.6 Hz, H-4), 4.02 (1H, t, J = 9.6 Hz, H-3), 4.02-4.05 (1H, m, H-5), 4.22 (1H, dd, J = 4.8 Hz, J = 11.7 Hz, H-6a), 4.30 (1H, dd, J = 2.1 Hz, J = 11.7 Hz, H-6b), 4.59 (1H, d, J = 10.3 Hz, CH2Ph), 4.69 (1H, d, J = 11.0 Hz, CH2Ph), 4.86-4.94 (4H, m, CH2Ph); 13C NMR (CDCl3): δ 14.0 (C-4’), 20.9 (COCH3), 22.7 (C-3’), 24.6 (C-2’), 38.2 (C-1’), 63.2 (C-6), 69.6 (C-5), 75.0 (CH2Ph), 75.5 (CH2Ph), 75.6 (CH2Ph), 78.2 (C-4), 81.3 (C-2), 83.8 (C-3), 98.4 (C-1), 170.8 (C=O); HRMS (ESI): m/z calcd for 571.2666 (C33H40O7·Na+); Found: 571.2687. 3c: Rf 0.17 (1:2 AcOEt-hexane); 1H NMR (CDCl3): δ 0.85 (3H, t, J = 6.9 Hz, H-4’), 1.16-1.36 (4H, m, H-2’, H-3’), 1.60-1.65 (2H, m, H-1’), 3.40 (1H, d, J = 8.9 Hz, H-2), 3.56 (1H, t, J = 9.6 Hz, H-4), 3.71-3.74 (1H, m, H-6a), 3.81 (1H, bd, J = 11.7 Hz, H-6b), 3.87-3.91 (1H, m, H-5), 4.02 (1H, t, J = 9.6 Hz, H-3), 4.67-4.94 (6H, m, CH2Ph); 13C NMR (CDCl3): δ 14.0 (C-4’), 22.8 (C-3’), 24.6 (C-2’), 38.3 (C-1’), 61.9 (C-6), 71.7 (C-5), 74.9 (CH2Ph), 75.4 (CH2Ph), 75.6 (CH2Ph), 78.1 (C-4), 81.2 (C-3), 83.6 (C-2), 98.4 (C-1); HRMS (ESI): m/z calcd for 529.2561 (C31H38O6·Na+); Found: 529.2610. The ordinary treatment of 2c using NaOMe in MeOH quantitatively afforded 3c.
6-O-Acetyl-2,3,4,-tri-O-benzyl-1-C-phenyl-D-glucopyranose (2d) and 2,3,4,-tri-O-benzyl-1-C-phenyl- D-glucopyranose (3d): The above same procedure using a 1.08 M THF solution of PhMgBr (0.95 mL, 1 mmol) and 1 (209 mg, 0.43 mmol) in THF (3 mL) gave 2d (PTLC; 1:2 AcOEt-hexane, 215 mg, 89%) as a colorless oil. 2d: Rf 0.43 (1:2 AcOEt-hexane); 1H NMR (CDCl3): δ 2.03 (3H, s, CH3), 3.54 (1H, d, J = 8.9 Hz, H-2), 3.71 (1H, t, J = 8.9 Hz, H-4), 3.80 (1H, d, J = 10.3 Hz, CH2Ph), 4.11 (1H, t, J = 8.9 Hz, H-3), 4.22-4.25 (1H, m, H-5), 4.31 (1H, dd, J = 4.2 Hz, J = 11.7 Hz, H-6a), 4.35 (1H, dd, J = 2.1 Hz, J = 11.7 Hz, H-6b), 4.39 (1H, d, J = 10.3 Hz, CH2Ph), 4.64 (1H, d, J = 11.0 Hz, CH2Ph), 4.88-4.92 (2H, m, CH2Ph), 4.96 (1H, d, J = 11.0 Hz, CH2Ph); 13C NMR (CDCl3): δ 20.9 (CH3), 63.3 (C-6), 70.1 (C-5), 75.0 (CH2Ph), 75.5 (CH2Ph), 75.7 (CH2Ph), 77.8 (C-4), 83.4 (C-3), 85.0 (C-2), 97.8 (C-1), 170.8 (C=O); HRMS (ESI): m/z calcd for 591.2353 (C35H36O7·Na+); Found 591.2398. The ordinary treatment of 2d using NaOMe in MeOH quantitatively afforded 3d as an amorphous solid. 3d: Rf 0.18 (1:2 AcOEt-hexane); 1H NMR (CDCl3): δ 3.56 (1H, d, J = 9.0 Hz, H-2), 3.71 (1H, t, J = 9.6 Hz, H-4), 3.75 (1H, dd, J = 4.1 Hz, J = 11.7 Hz, H-6a), 3.80 (1H, d, J = 10.3 Hz, CH2Ph), 3.85 (1H, m, H-6b), 3.89 (1H, m, H-5), 4.08 (1H, t, J = 8.9 Hz, H-3), 4.38 (1H, d, J = 10.3 Hz, CH2Ph), 4.71 (1H, d, J = 11.0 Hz, CH2Ph), 4.89-4.94 (3H, m, CH2Ph); 13C NMR (CDCl3): δ 62.0 (C-6), 72.3 (C-5), 75.0 (CH2Ph), 75.4 (CH2Ph), 75.7 (CH2Ph), 78.1 (C-4), 83.3 (C-3), 85.1 (C-2), 97.9 (C-1); HRMS (ESI): m/z calcd for 549.2248 (C33H34O6·Na+); Found: 549.2250.
6-O-Acetyl-1-C-benzyl-2,3,4,-tri-O-benzyl-D-glucopyranose (2e) and 1-C-benzyl-2,3,4,-tri-O-benzyl- D-glucopyranose (3e): The above same procedure using a 1.0 M Et2O solution of PhCH2MgCl (1.05 mL, 1 mmol) and 1 (214 mg, 0.44 mmol) in THF (3 mL) gave 2e (PTLC; 1:2 AcOEt-hexane, 172 mg, 82%) as a colorless oil. 2e: Rf 0.41 (1:2 AcOEt-hexane); 1H NMR (CDCl3): δ 2.02 (3H, s, CH3) , 2.82 (1H, d, J = 13.7 Hz, H-1’a), 3.00 (1H, d, J = 13.7 Hz, H-1’b), 3.40 (1H, d, J = 8.9 Hz, H-2), 3.50 (1H, t, J = 9.6 Hz, H-4), 3.93-3.95 (1H, m, H-5), 4.09 (1H, t, J = 8.9 Hz, H-3), 4.24 (1H, dd, J = 4.9 Hz, J = 11.7 Hz, H-6a), 4.28 (1H, dd, J = 1.4 Hz, J = 11.7 Hz, H-6b), 4.59 (1H, d, J = 11.0 Hz, CH2Ph), 4.72 (1H, d, J = 11.0 Hz, CH2Ph), 4.87 (1H, d, J = 11.0 Hz, CH2Ph), 4.90-4.94 (2H, m, CH2Ph), 5.00 (1H, d, J = 11.0 Hz, CH2Ph); 13C NMR (CDCl3): δ 20.9 (CH3), 43.5 (C-1’), 63.0 (C-6), 69.5 (C-5), 75.0 (CH2Ph), 75.4 (CH2Ph), 75.7 (CH2Ph), 78.3 (C-4), 81.3 (C-3), 83.8 (C-2), 97.7 (C-1), 170.7 (C=O); HRMS (ESI): m/z calcd for 605.2510 (C36H38O7·Na+); Found: 605.2532. The ordinary treatment of 2e using NaOMe in MeOH quantitatively afforded 3e as an amorphous solid. 3e: Rf 0.25 (1:2 AcOEt-hexane); 1H NMR (CDCl3): δ 2.80 (1H, d, J = 13.0 Hz, H-1’a), 3.02 (1H, d, J = 13.8 Hz, H-1’b), 3.41 (1H, d, J = 9.0 Hz, H-2), 3.54 (1H, t, J = 9.6 Hz, H-4), 3.68-3.84 (3H, m, H-5, H-6), 4.08 (1H, t, J = 9.0 Hz, H-3), 4.65-5.03 (6H, m, CH2Ph); 13C NMR (CDCl3): δ 43.7 (C-1’), 62.0 (C-6), 71.6 (C-5), 75.0 (CH2Ph), 75.4 (CH2Ph), 75.7 (CH2Ph), 78.2 (C-4), 81.4 (C-2), 83.7 (C-3), 97.5 (C-1); HRMS (ESI): m/z calcd for 563.2404 (C34H36O6·Na+); Found: 563.2443.
(1R,2R,3S,4R,5R)-2,3,4-Tris(benzyloxy)-5-methyl-6,8-dioxabicyclo[3.2.1]octane (5a): To a stirred solution of TfOH (0.48 µL, 0.0054 mmol) was added 3a (50 mg, 0.11 mmol) in MeCN (2 mL) at 0 oC in the presence of dry CaSO4 (ca. 100 mg) in an Ar atmosphere. The resulting mixture was stirred for 2 h. The reaction was then quenched by the addition of a sat. aq. NaHCO3 solution (5 mL). The reaction mixture was extracted with AcOEt, and the organic layer was washed with water and a sat. aq. NaCl solution. After the organic layer was dried over Na2SO4, the solvent was evaporated under reduced pressure. The crude product was purified by preparative silica gel TLC (1:2 AcOEt-hexane) to give 5a (45 mg, 93%) as a colorless oil. 5a: Rf 0.54 (1:2 AcOEt-hexane); [α]D23 -47.7◦ (c 1.54, CHCl3); 1H NMR (CDCl3): δ 1.49 (3H, s, H-1’), 3.23 (1H, s, H-4), 3.31 (1H, s, H-2), 3.59 (1H, s, H-3), 3.75 (1H, t, J = 6.9 Hz, H-7a), 3.96 (1H, d, J = 6.9 Hz, H-7b), 4.34-4.39 (3H, m, CH2Ph), 4.53 (1H, m, CH2Ph), 4.53 (1H, d, J = 6.2 Hz, H-1), 4.58-4.61 (2H, m, CH2Ph); 13C NMR (CDCl3): δ 21.0 (C-1’), 65.5 (C-7), 71.0 (CH2Ph), 71.2 (CH2Ph), 72.3 (CH2Ph), 74.5 (C-2), 75.2 (C-3), 75.7 (C-1), 77.3 (C-4), 107.0 (C-5); HRMS (ESI): m/z calcd for 469.1991 (C28H30O5·Na+); Found: 469.2032.
(1R,2R,3S,4R,5R)-5-Allyl-2,3,4-tris(benzyloxy)-6,8-dioxabicyclo[3.2.1]octane (5b): The above same procedure using TfOH (0.95 µL, 0.011 mmol) and 3b (104.9 mg, 0.21 mmol) in MeCN (2 mL) gave 5b (PTLC; 1:2 AcOEt-hexane, 91.4 mg, 90%) as a colorless oil. 5b: Rf 0.64 (1:2 AcOEt-hexane); [α]D23 +7.6◦ (c 4.57, CHCl3); 1H NMR (CDCl3): δ 2.56 (1H, dd, J = 7.6 Hz, J = 14.4 Hz, H-1’a), 2.64 (1H, dd, J = 7.6 Hz, J = 14.4 Hz, H-1’b), 3.24 (1H, s, H-4), 3.26 (1H, s, H-2), 3.54 (1H, s, H-3), 3.66 (1H, dd, J = 6.2 Hz, J = 6.9 Hz, H-7a), 3.94 (1H, d, J = 6.9 Hz, H-7b), 4.29-4.34 (3H, m, CH2Ph), 4.41-4.52 (3H, m, CH2Ph), 4.56 (1H, d, J = 5.5 Hz, H-1), 5.03-5.05 (2H, m, H-3’), 5.74-5.79 (1H, m, H-2’); 13C NMR (CDCl3) δ 38.1 (C-1’), 65.7 (C-7), 70.9 (CH2Ph), 71.5 (CH2Ph), 72.2 (CH2Ph), 74.6 (C-2), 74.9 (C-3), 75.5 (C-1), 76.2 (C-4), 107.2 (C-5), 118.4 (C-3’), 132.0 (C-2’); HRMS (ESI): m/z calcd for 495.2147 (C30H32O5·Na+); Found: 495.2195.
(1R,2R,3S,4R,5R)-2,3,4-Tris(benzyloxy)-5-butyl-6,8-dioxabicyclo[3.2.1]octane (5c): The above same procedure using TfOH (0.53 μL, 0.006 mmol) and 3c (53.6 mg, 0.11 mmol) in MeCN (2 mL) gave 5c (PTLC; 1:2 AcOEt-hexane, 49.1 mg, 95%) as a colorless oil. 5c: Rf 0.7 (1:2 AcOEt-hexane); [α]D23 -36.7◦ (c 1.08, CHCl3); 1H NMR (CDCl3) δ 0.84 (3H, t, J = 6.9 Hz, H-4’), 1.13-1.19 (1H, m, H-2’a), 1.20-1.31 (2H, m, H-3’), 1.33-1.40 (1H, m, H-2’b), 1.75 (1H, dt, J = 4.1 Hz, J = 13.7 Hz, H-1’a), 1.90 (1H, dt, J = 3.4 Hz, J = 13.7 Hz, H-1’b), 3.25 (1H, s, H-4), 3.31 (1H, s, H-2), 3.60 (1H, s, H-3), 3.70 (1H, t, J = 6.9 Hz, H-7a), 3.98 (1H, d, J = 6.9 Hz, H-7b), 4.32-4.40 (3H, m, CH2Ph), 4.49-4.57 (3H, m, CH2Ph), 4.59 (1H, d, J = 6.2 Hz, H-1); 13C NMR (CDCl3) δ 14.0 (C-4’), 22.9 (C-3’), 24.1 (C-2’), 32.9 (C-1’), 65.6 (C-7), 70.9 (CH2Ph), 71.4 (CH2Ph), 72.0 (CH2Ph), 74.7 (C-2), 75.0 (C-3), 75.4 (C-1), 76.2 (C-4), 107.9 (C-5). HRMS (ESI): m/z calcd for 511.2460 (C31H36O5·Na+); Found: 511.2510.
(1R,2R,3S,4R,5R)-2,3,4-Tris(benzyloxy)-5-phenyl-6,8-dioxabicyclo[3.2.1]octane (5d): The above same procedure using TfOH (0.58 μL, 0.0065 mmol) and 3d (67.9 mg, 0.13 mmol) in MeCN (2 mL) gave 5d (PTLC; 1:2 AcOEt-hexane, 51.2 mg, 78%) as a colorless oil and 5d-t (9 mg, 14%) as a colorless oil. 5d: Rf 0.66 (1:2 AcOEt-hexane); [α]D23 -3.5◦ (c 2.71, CHCl3); 1H NMR (CDCl3): δ 3.39 (1H, s, H-4), 3.50 (1H, s, H-2), 3.68 (1H, s, H-3), 3.81 (1H, t, J = 6.2 Hz, H-7a), 4.01-4.06 (2H, m, CH2Ph), 4.07 (1H, d, J = 6.9 Hz, H-7b), 4.31-4.36 (2H, m, CH2Ph), 4.56 (1H, d, J = 13.1 Hz, CH2Ph), 4.67 (1H, d, J = 13.1 Hz, CH2Ph), 4.79 (1H, d, J = 6.2 Hz, H-1); 13C NMR (CDCl3): δ 65.6 (C-7), 71.0 (CH2Ph), 71.6 (CH2Ph), 72.2 (CH2Ph), 74.6 (C-2), 76.2 (C-3), 76.3 (C-1), 77.6 (C-4), 106.9 (C-5); HRMS (ESI): m/z calcd for 531.2147 (C33H32O5·Na+); Found: 531.2196. Its twist boat isomer (5d-t): Rf 0.59 (1:2 AcOEt-hexane); [α]D23 -74.5◦ (c 0.47, CHCl3); 1H NMR (CDCl3): δ 3.55 (1H, s, H-2), 3.77 (1H, d, J = 5.5 Hz, H-4), 3.86 (1H, d, J = 5.5 Hz, H-3), 3.94 (1H, dd, J = 8.2 Hz, J = 5.5 Hz, H-7a), 3.95 (1H, d, J = 11.7 Hz, CH2Ph), 4.40 (1H, d, J = 11.7 Hz, CH2Ph), 4.42 (1H, d, J = 8.2 Hz, H-7b), 4.44 (1H, d, J = 12.4 Hz, CH2Ph), 4.45 (1H, d, J = 12.4 Hz, CH2Ph), 4.53 (1H, d, J = 12.4 Hz, CH2Ph), 4.63 (1H, d, J = 6.2 Hz, H-1), 4.66 (1H, d, J = 12.4 Hz, CH2Ph); 13C NMR (CDCl3): δ 66.1 (C-7), 71.2 (CH2Ph), 72.4 (CH2Ph), 73.3 (CH2Ph), 75.0 (C-3), 75.4 (C-1), 76.9 (C-2), 77.7 (C-4), 107.6 (C-5); HRMS (ESI): m/z calcd for 531.2147 (C33H32O5·Na+); Found: 531.2178.
(1R,2R,3S,4R,5R)-5-Benzyl-2,3,4-tris(benzyloxy)-6,8-dioxabicyclo[3.2.1]octane (5e): The above same procedure using TfOH (0.53 μL, 0.006 mmol) and 3e (64.4 mg, 0.12 mmol) in MeCN (2 mL) gave 5e (PTLC; 1:2 AcOEt-hexane, 45.3 mg, 70%) as a colorless oil and 5e-t (16.3 mg, 26%) as a colorless oil. 5e: Rf 0.66 (1:2 AcOEt-hexane); [α]D23 -35.1◦ (c 2.32, CHCl3); 1H NMR (CDCl3): δ 2.97 (1H, d, J = 13.4 Hz, H-1’a), 3.23 (1H, s, H-4), 3.29 (1H, s, H-2), 3.34 (1H, d, J = 13.7 Hz, H-1’b), 3.45 (1H, t, J = 6.9 Hz, H-7a), 3.60 (1H, s, H-3), 3.91 (1H, d, J = 6.9 Hz, H-7b), 4.33 (3H, m, CH2Ph), 4.43 (1H, d, J = 12.4 Hz, CH2Ph), 4.50 (1H, d, J = 12.4 Hz, CH2Ph), 4.53 (1H, d, J = 5.5 Hz, H-1), 4.56 (1H, d, J = 13.0 Hz, CH2Ph); 13C NMR (CDCl3): δ 39.5 (C-1’), 65.7 (C-7), 70.9 (CH2Ph), 71.5 (CH2Ph), 72.0 (CH2Ph), 74.5 (C-2), 74.9 (C-3), 75.3 (C-1), 76.8 (C-4), 107.2 (C-5); HRMS (ESI): m/z calcd for 545.2304 (C34H34O5·Na+); Found: 545.2354. Its twist boat isomer (5e-t): Rf 0.52 (1:2 AcOEt-hexane); [α]D23 -19.3◦ (c 1.97, CHCl3); 1H NMR (CDCl3): δ 2.95 (1H, d, J = 14.4 Hz, H-1’a), 3.43 (1H, d, J = 14.4 Hz, H-1’b), 3.48 (1H, d, J = 1.4 Hz, H-2), 3.52 (1H, t, J = 5.5 Hz, H-7a), 3.56 (1H, d, J = 4.8 Hz, H-4), 3.81 (1H, d, J = 5.5 Hz, H-3), 4.11 (1H, d, J = 6.9 Hz, H-7b), 4.25 (1H, d, J = 11.6 Hz, CH2Ph), 4.40 (1H, d, J = 5.5 Hz, H-1), 4.43-4.51 (5H, m, CH2Ph); 13C NMR (CDCl3): δ 39.1 (C-1’), 65.9 (C-7), 71.2 (CH2Ph), 71.3 (CH2Ph), 73.0 (CH2Ph), 74.2 (C-3), 75.1 (C-1), 76.2 (C-2), 76.5 (C-4), 107.8 (C-5); HRMS (ESI): m/z calcd for 545.2304 (C34H34O5·Na+); Found: 545.2352.
References
1. F. B. La Forge and C. S. Hudson, J. Biol. Chem., 1917, 30, 61.
2. M. Takenaka and H. Ono, Tetrahedron Lett., 2003, 44, 999. CrossRef
3. T. Hatano, R. Yoshihara, S. Hattori, M. Yoshizaki, T. Shingu, and T. Okuda, Chem. Pharm. Bull., 1992, 40, 1703.
4. Z. Hricoviniova-Bilikova and L. Petrus, Carbohydr. Res., 1999, 320, 31. CrossRef
5. C. G. Francisco, A. J. Herrera, and E. Suarez, J. Org. Chem., 2002, 67, 7439. CrossRef
6. T. Yamanoi, K. Matsumura, S. Matsuda, and Y. Oda, Synlett, 2005, 2973. CrossRef
7. S. Matsuda, K. Matsumura, M. Watanabe, and T. Yamanoi, Tetrahedron Lett., 2007, 48, 5807. CrossRef
8. S. Matsuda, T. Yamanoi, and M. Watanabe, Tetrahedron, 2008, 64, 8082. CrossRef
9. T. Yamanoi and S. Matsuda, Heterocycles, 2009, 79, 163 and references cited herein. CrossRef
10. S. Horito, K. Asano, K. Umemura, H. Hashimoto, and J. Yoshiumra, Carbohydr. Res., 1983, 121, 175. CrossRef
11. ‘Stereoelectronic Effect’, ed. A. J. Kirby, Oxford University Press Inc., New York, 1996.
12. T. Yamanoi, S. Matsuda, I. Yamazaki, R. Inoue, K. Hamasaki, and M. Watanabe, Heterocycles, 2006, 68, 673. CrossRef
13. T. Yamanoi, Y. Oda, S. Matsuda, I. Yamazaki, K. Matsumura, K. Katsuraya, M. Watanabe, and T. Inazu, Tetrahedron, 2006, 62, 10383. CrossRef
14. T. B. Grindley, Glycoscience: Chemistry and Chemical Biology I; Ed. by B. Fraser-Reid, K. Tatsuta, and J. Thieme, Springer: Berlin, 2001, pp. 4-51.