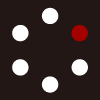
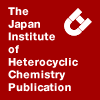
HETEROCYCLES
An International Journal for Reviews and Communications in Heterocyclic ChemistryWeb Edition ISSN: 1881-0942
Published online by The Japan Institute of Heterocyclic Chemistry
e-Journal
Full Text HTML
Received, 10th March, 2010, Accepted, 13th May, 2010, Published online, 13th May, 2010.
DOI: 10.3987/COM-10-11940
■ Synthesis and Structure of 5,7-Dimethylthieno[3,4-d]pyridazine
Nathan C. Tice,* Sarah M. Peak, and Sean Parkin
Department of Chemistry, Eastern Kentucky University, Richmond, KY, 40475-3102, U.S.A.
Abstract
The reaction of 2,5-dimethylthiophene-3,4-dicarboxaldehye (3) with excess hydrazine hydrate afforded the desired 5,6-fused ring thiophene derivative, 5,7-dimethylthieno[3,4-d]pyridazine (4), in high yield (82%). Pyridazine 4 was characterized spectroscopically and its structure was confirmed by X-ray crystallography. Compound 4 was observed to have an inverted, coplanar stacking arrangement in the solid state.INTRODUCTION
Thiophenes and their fused-ring derivatives have long been of interest for use in next generation electronic materials due to their ease of production, synthetic versatility, and low cost compared to traditional inorganic materials (e.g., silicon).1,2 We are primarily interested in facile pathways towards novel thiophene derivatives that incorporate other heteroatoms in fully aromatic systems. Specifically, synthesis of fused-pyridazines has been an alluring target, as formation of their highly stable derivatives has been well documented.3-5 Pyridazines have also been found to have numerous, real world applications in catalysis,6 gas storage,7 polymeric sensors,8 and biological mimetics.9 Herein we report upon the 3-step formation and characterization of 5,7-dimethylthieno[3,4-d]pyridazine (4) employing its diformyl precursor, 2,5-dimethylthiophene-3,4-dicarboxaldehye (3).
RESULTS AND DISCUSSION
The formation of fused-ring pyridazines has typically been accomplished from a 1,4-diketone or keto-aldehyde aromatic precursor, with benzene or cyclopentadiene (Cp) substrates as those most typically employed.10-12 Previously, we have had excellent results in forming stable organic and organometallic pyridazine compounds from Cp percursors.5,13 However, we wished to incorporate the novel synthetic and electronic properties that thiophene substrates possess to form a functional thienopyridazine derivative. This derivative would serve as a synthetic model and precursor for a future heterocylic conducting polymer. Formation of the pyridazine 4 was accomplished via a 3-step pathway employing 2,5-dimethyl thiophene (1) as the initial substrate (Scheme 1).
Although an analogous route towards the formation of 4 was previously reported by El’tsov and Ginesina,14 no spectroscopic or structural analysis was performed. The only evidence presented by the authors for formation of the desired fused pyridazine was its melting point (146-147 °C). Formation of the bischloromethylation product, 3,4-(bischloromethyl)-2,5-dimethyl thiophene (2) was accomplished by reaction of compound 1 with aqueous formaldehyde and concentrated HCl in good yield (85%) using the procedure reported by Wallace and Selegue.15 The chloromethylated product 2 was then converted into the diformyl analog, 2,5-dimethylthiophene-3,4-dicarboxaldehye (3) in good yield (77%). This transformation, previously reported by Brown and coworkers,16 employs a mixture of 2-nitropropane and sodium metal in ethanol for the formylation step. Repeated attempts to substitute the sodium metal for a more convenient base (e.g, sodium ethoxide) led to only trace amounts of the desired dialdehyde 3. Thus, the original procedure was used as reported. The desired target compound, 5,7-dimethylthieno[3,4-d]pyridazine (4), was formed also in good yield (82%) by ring closure of compound 3 with excess hydrazine hydrate. The fused ring pyridazine 4 displays high air and solution stability in a wide variety of solvents. The structure of compound 4 was confirmed by IR, NMR, and GC/MS analysis. The 1H NMR of 4 displays the expected two singlets at 2.56 (6H) and 8.93 (2H) corresponding to the methyl and imine protons respectively, as well as the disappearance of the aldehyde functional group (10.35 ppm). Mass spectrometry also confirms the molecular weight of 4, with the both the base and M+ peaks at 164. IR spectroscopy also showed evidence of formation of the C-N double bond (1552 cm-1), with loss of the carbonyl stretch observed in 3.
The structure of pyridazine 4 was also confirmed by X-Ray crystallographic analysis (Figure 1).
Compound 4 crystallizes in a monoclinic P 21/c space group, with four molecules in the unit cell. The imine carbon bond lengths, N1-C3 and N2-C4, are nearly identical at 1.308(2) and 1.305(2) Å respectively. The C-C bonds within the thiophene portion of 4 are significantly shorter compared to those in the pyridazine ring. The bond lengths for C1-C2 and C5-C6 are 1.380(2) and 1.377(2) Å respectively compared to those of C2-C3 and C4-C5 at 1.427(2) and 1.428(2) Å respectively (Figure 1). This observation most likely reflects the dominance of the aromatic thiophene resonance form versus the “non-classical” thiophene resonance form that is commonly believed to be a factor in the stability of 5,6-fused ring thiophenes.17 The central thienopyridazine portion of 4 is highly planar, with the root-mean-square deviation from the planes created by C1-C6, N1, N2, and S1 in each of the four unique molecules averaging to 0.0154 Å. The methyl substituents C7 and C8 are also nearly planar with respect to the central fused ring, with deviations from least-squares plane of 0.0660 and 0.0190 Å respectively.
Compound 4 exhibited an inverted, coplanar arrangement or “face-to-face” stacking, with the thiophene portion of one molecule aligning itself with the pyridazine portion of the next (Figures 2 and 3). This face-to-face stacking is desirable, as it leads to more efficient charge transfer and thus better conductivity in molecular electronic materials.18 However, no short contacts (atoms positioned at less than the sum of the van der Waals radii from each other) are observed between these layers of molecules. For example, the distances between S1 on the (x,y,z) molecule and N1/N2 on the "upper" or (1-x,1-y,1-z) molecule are 3.360 Å and 3.367 Å respectively (Figure 3). The corresponding distances to the "lower" or (-x,1-y,1-z) molecule are 3.794 Å and 3.636 Å respectively (the sum of the van der Waals radii for S and N is 3.35 Å). However, the interplanar distance for the “upper pair” of molecules of 4, (x,y,z) and (1-x,1-y,1-z), is 3.4449 Å (Figure 3). Similarly, this distance for the “lower pair,” (x,y,z) and (-x,1-y,1-z) is 3.4799 Å, which does fall into the commonly accepted range of π-interaction or “π-stacking” (3.4-3.6 Å).19 Thus, while there may be some evidence of molecular orbital interaction between molecules in this stacked arrangement, their actual π-interaction is likely weak due to the lack of short contacts throughout.
Tables of crystallographic details, atomic coordinates and displacement parameters, bond distances and angles, intermolecular contact distances, structure factors and a crystallographic information file (CIF) for the structure of 4 have been deposited with the Cambridge Crystallographic Data Centre.20
EXPERIMENTAL
General Procedures. All reactions were carried out using standard Schlenk techniques under a nitrogen atmosphere unless otherwise noted. NMR solvent CDCl3 (Aldrich) was used without further purification. Hydrazine hydrate, MeOH, magnesium sulfate, Et2O, CH2Cl2, pentane, silica and 2,5-dimethylthiophene (1) (Aldrich) were also used without further purification. Thiophene precursors 3,4-(bischloromethyl)-2,5-dimethyl thiophene (2) and 3 were prepared according to literature methods15,16 and their structural identities were confirmed by NMR and GC/MS.
1H and 13C NMR spectra were recorded on a JOEL-300 MHz NMR spectrometer at ca. 22 oC and were referenced to residual solvent peaks. All 13C NMR spectra were listed as decoupled. Infrared spectra were recorded on Spectrum One FT-IR Spectrometer. Electron ionization (EI) mass spectra were recorded at 70 eV on a Perkin Elmer GC/MS. Melting points were taken on a standard Mel-Temp apparatus. X-Ray diffraction data were collected at 90 K on a Bruker-Nonius X8 Proteum diffractometer at the University of Kentucky X-Ray Crystallographic Laboratories. Elemental analysis was performed at Atlantic Microlabs, Inc. in Norcross, GA.
Synthesis of 5,7-dimethylthieno[3,4-d]pyridazine (4) In a 50 mL round bottom flask, 3 (260 mg, 1.55 mmol) was added to a solution of 20 mL of MeOH and 2 mL of 64% hydrazine hydrate (excess). This was allowed to stir overnight at room temperature. The reaction mixture was quenched with water and then extracted with CH2Cl2. The organic layer was collected, dried over magnesium sulfate, and filtered. The solvent was removed under reduced pressure to give 4, as a yellow solid (208 mg, 82%). An analytically pure sample was obtained by chromatographing 4 with Et2O over a thick pad of silica followed by trituration with cold pentane. Mp 117.3-120.5 °C. 1H NMR (300 MHz, CDCl3, ppm): δ 2.79 (s, 6H, Me), 9.11 (s, 2H, CH). 13C NMR (75 MHz, CDCl3, ppm): δ 12.09 (Me), 127.57 (C), 135.12 (C), 145.70 (CH). IR (KBr, cm-1): 1552 (CN stretch). GC/MS (EI, pos): m/z 164 (M+ and base peak). Analysis for C8H8N2S: Calc. C, 58.50; H, 4.90; N, 17.10. Found C, 57.95; H, 4.89; N, 16.70.
The X-Ray crystallographic structure of 4 was determined by X-Ray crystallographic methods. The crystals for which data were collected were typical of others in the batch, which had been grown by slow evaporation from methylene chloride at room temperature. These crystals were mounted on glass fibers with Paratone N oil. Data were collected at 90 K on a Bruker-Nonius X8 Proteum diffractometer. The main programs used were Bruker APEX2 to obtain cell parameters and for data reduction, SADABS for absorption correction,21 SHELXS-97 for structure solution, and SHELXL-97 for refinement.22 Hydrogen atoms were placed in geometrically calculated positions. Crystal data and a summary of experimental details are given in Table 1.
ACKNOWLEDGEMENTS
We wish to thank our sources of support for this research, including the Department of Chemistry, the Junior Faculty Summer Research Award from the College of Arts and Sciences and the Chemistry Diversity Initiative at Eastern Kentucky University. We also wish to thank Dr. Jay Baltisberger at Berea College in Berea, KY for his support on the NMR spectroscopy.
References
1. A. R. Katritzky and C. W. Rees, 'Comprehensive heterocyclic chemistry: structure, reactions, synthesis and uses of heterocyclic compounds,' Pergamon Press: Oxford, 1984.
2. J. Roncali, Chem. Rev., 1997, 97, 173. CrossRef
3. C. E. Wallace, J. P. Selegue, and A.Carrillo, Organometallics, 1998, 17, 3390. CrossRef
4. M. T. Blankenbuehler, 'Approaches to Organometallic-Fused Heterocyles,' Ph.D. Thesis, Department of Chemistry, University of Kentucky, Lexington, KY, 1999.
5. N. C. Tice, 'The Synthesis, Structure, and Reactivity of Some Organometallic-Fused Heterocycles,' Ph.D. Thesis, Department of Chemistry, University of Kentucky, Lexington, KY, 2006.
6. Y. Xu, T. Akermark, V. Gyollai, D. Zou, L. Eriksson, L. Duan, R. Zhang, B. Akermark, and L. A. Sun, Inorg. Chem., 2009, 48, 2717. CrossRef
7. B. S. Ghanem, K. J. Msayib, N. B. McKeown, K. D. M. Harris, Z. Pan, P. M. Budd, A. Butler, J. Selbie, D. Book, and A. Walton, Chem. Commun., 2007, 67. CrossRef
8. J. S. Yang and T. M. Swager, J. Am. Chem. Soc., 1998, 120, 5321. CrossRef
9. A. Volonterio, L. Moisan, and J. Rebek, Org. Lett., 2007, 9, 3733. CrossRef
10. A. G. J. Anderson and D. M. Forkey, J. Am. Chem. Soc., 1969, 91, 924. CrossRef
11. K. Hafner, H. E. A. Kramer, H. Musso, G. Ploss, and G. Schultz, Chem. Ber., 1964, 97, 2066. CrossRef
12. W. J. Linn and W. H. Sharkey, J. Am. Chem. Soc., 1957, 79, 4970. CrossRef
13. C. A. Snyder, N. C. Tice, P. G. Sriramula, J. L. Neathery, J. K. Mobley, C. L. Phillips, A. Z. Preston, J. M. Strain, E. S. Vanover, M. P. Starling, N. V. Sahi, and K. R. Bunnell, Synth. Commun., 2010, accepted manuscript.
14. A. V. El’tsov and A. A. Ginesina, Zh. Org. Khim., 1967, 3, 191.
15. C. E. Wallace, 'The Synthesis, Characterization, and Reactivity of Some Ruthenocene-fused Heterocycles, ' Ph.D. Thesis, Department of Chemistry, University of Kentucky, Lexington, KY, 1998.
16. T. M. Brown, W. Carruthers, and M. G. Pellatt, J. Chem. Soc., Perkin Trans. 1, 1982, 2, 483. CrossRef
17. F. Wuld, M. Kobayashi, and A. J. Heeger, J. Org. Chem., 1984, 49, 3382. CrossRef
18. J. E. Anthony, Chem. Rev., 2006, 106, 5028. CrossRef
19. M. D. Curtis, J. Cao, and J. W. Kampf, J. Am. Chem. Soc., 2004, 126, 4318. CrossRef
20. CCDC 767317 contains the supplementary crystallographic data for this paper. These data can be obtained free of charge from The Cambridge Crystallographic Data Centre via www.ccdc.cam.ac.uk/data_request/cif.
21. Bruker, 2009. APEX2 and SADABS. Bruker AXS Inc., Madison, Wisconsin, USA.
22. G. M. Sheldrick, Acta Cryst., 2008, A64, 112.