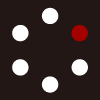
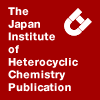
HETEROCYCLES
An International Journal for Reviews and Communications in Heterocyclic ChemistryWeb Edition ISSN: 1881-0942
Published online by The Japan Institute of Heterocyclic Chemistry
e-Journal
Full Text HTML
Received, 11th March, 2010, Accepted, 13th May, 2010, Published online, 13th May, 2010.
DOI: 10.3987/COM-10-11943
■ Synthesis of Some Novel Benzobisthiazoles
Oscene V. Barrett, Nadale K. Downer-Riley, and Yvette A. Jackson*
Department of Chemistry, Mona Campus, University of the West Indies, Mona, Kingston 7, Jamaica
Abstract
The synthesis of angular and linear benzobisthiazoles via reaction of o-methoxythiobenzanilides with AIBN and basic ferricyanide is described.INTRODUCTION
The potent and selective antitumour activity of 2-arylbenzothiazoles has been well established.1 Although benzobisthiazoles have been less researched, a few reports have indicated that these compounds demonstrate potent anti-inflammatory, antibacterial, antifungal and antiprotozoal activity.2-4 In general, linear benzobisthiazoles (type B) have been found to display higher biological activity than their angular counterparts (type A).2, 3 We therefore sought to synthesize benzobisthiazoles in order to evaluate their biological activity.
Benzobisthiazoles have previously been synthesized by oxidative cyclization of phenyldithiourea5 or condensation of p-phenylenediaminedithiols with substituted nitriles, aldehydes or carboxylic acids.6 In addition, we have demonstrated that a common ortho-methoxythiobenzanilide may serve as a precursor to either of two benzothiazoles depending on the conditions used (Scheme 1).7 Subjecting o-methoxythiobenzanilides to radical conditions may yield benzothiazoles with loss of the ortho methoxy group unlike the more traditional methods such as the Jacobson synthesis (potassium ferricyanide and base), which commonly yield benzothiazole from loss of the ortho hydrogen. In this paper, we report the extension of our previous work to effect synthesis of both angular and linear benzobisthiazoles.
RESULTS AND DISCUSSION
Compounds 1a-c were chosen as substrates and were obtained from commercially available 2,5-dimethoxyaniline via the corresponding benzanilides as shown in Scheme 2. 4,7-Dimethoxy-5-nitro-2-phenylbenzothiazole (6a) and 4,7-dimethoxy-6-nitro-2-phenylbenzothiazole (6b) were synthesized from 2,5-dimethoxyaniline (5) in four steps with an overall yield of 50%.8
Palladium catalyzed reduction of nitrophenylbenzothiazoles 6a and 6b in the presence of hydrogen gave the corresponding aminophenylbenzothiazoles in almost quantitative yield. Upon treatment of aminophenylbenzothiazoles with benzoyl chloride in pyridine followed by thionation of the resulting benzanilides, thiobenzanilides 1a and 1b were obtained in 65% and 70% respectively over the 3 steps. 2,5-Dimethoxy-1,4-dithiobenzanilide 1c was also synthesized as shown in Scheme 2. 6-Amino-4,7-dimethoxybenzanilide 8 was obtained from compound 5 in three steps.8 Treatment of compound 8 with benzoyl chloride in pyridine followed by thionation of the resulting dibenzanilide 9 with Lawesson’s reagent furnished dithiobenzanilide 1c.
The Jacobson reaction is known to induce cyclization of thiobenzamides with loss of the ortho hydrogen.9 We have reported, however, that under these conditions (potassium ferricyanide and base), cyclization of highly activated o-methoxythiobenzanilides may proceed with loss of the ortho methoxy group.7 Subjecting compound 1a to Jacobson conditions yielded no linear benzobisthiazole from loss of the ortho hydrogen, and 1b yielded only minor amounts of the corresponding 2b (Table 1). Not surprisingly, benzobisthiazoles 3a and 3b, formed by loss of the ortho methoxy group, were the dominant products. As was expected too,7 the reaction of 2-methoxythiobenzanilides 1a and 1b with 2,2′-azobisisobutyronitrile (AIBN), proceeded with loss of methoxy radical to give benzobisthiazoles 3a and 3b as the only products.
Under these (Jacobson and AIBN) conditions, however, thiobenzanilide 1c did not produce linear benzobisthiazole 3c by double ipso substitution of the methoxy groups. Under both sets of reaction conditions, benzobisthiazole 3b was obtained showing cyclization with loss of ortho methoxy group at one thiobenzanilide moiety and loss of ortho hydrogen at the other. Compound 1d, the product of cyclization with loss of one methoxy group, was also isolated from these reactions (Table 2, entry 3).
Treatment of thiobenzanilide 1d with AIBN or basic K3Fe(CN)6 resulted in cyclization with loss of the ortho hydrogen yielding 3b in 45% and 35% respectively, and indicating that 1d was an intermediate in the conversion of 1c to 3b. Complete conversion of 1c to 3b was not observed even with extended reaction time or addition of excess reagents.
We have thus successfully synthesized the novel benzobisthiazoles 2b, 3a, and 3b.We will continue work in this area to gain a better understanding of the factors which give rise to the selectivity in the cyclization reaction. Initial screening of these compounds against common bacterial pathogens such as B. subtilis, P. aeruginosa, E. coli, and S. aureus indicated no antibacterial activity.
EXPERIMENTAL
1. General
IR spectra were obtained on a Perkin-Elmer 735B FT-IR spectrometer as KBr discs. NMR spectra (Bruker 200 and 500 MHz) were obtained in CDCl3 solution and the resonances are reported in δ units downfield from TMS as an internal standard; J values are given in Hz. The 1H NMR spectroscopic data for all known compounds were identical to that reported in the literature. Column chromatography was carried out using silica gel as the adsorbent. All elemental analyses were done by MEDAC Ltd, Egham, Surrey, UK.
1.1. General procedure for the benzoylation of anilines
To a solution of an aniline (6.0 g) in dry toluene (35 mL) and pyridine (25 mL), benzoyl chloride (1.2 molar equiv.) was added. The solution was heated at reflux for 2 - 6 h, cooled to room temperature and poured into water (200 mL). The two layers were separated and the aqueous layer extracted with EtOAc (3 x 25 mL). The organic fractions were combined, washed with 1M HCl (3 x 25 mL) followed by saturated aqueous sodium hydrogen carbonate (3 x 25 mL) and then dried over magnesium sulfate. The solution was filtered and the solvent removed in vacuo to yield the corresponding benzanilide, which was recrystallized from MeOH or CH2Cl2 –hexanes.
1.1.1. N-(2-Phenyl-4,7-dimethoxybenzothiazol-5-yl)benzamide (7a).
Off-white crystals (70%); mp 185-186 oC (MeOH); Anal. Calcd for C22H18N2O3S: C, 67.67; H, 4.65; N, 7.17%. Found: C, 67.97; H, 4.72; N, 7.23%; IR νmax/cm-1 3437, 2818, 1684, 1525; 1H NMR 3.95 (3H, s, OCH3), 4.36 (3H, s, OCH3), 7.53 (6H, m, H-3′,4′,5′ and H-3″,4″,5″), 7.91 (2H, d, J = 7 Hz, H-2′, 6′), 8.12 (2H, d, J = 3 Hz, H-2″, 6″), 8.36 (1H, s, H-6), 8.87 (1H, s, N-H); 13C NMR δ 56.0, 56.6, 99.1, 99.3, 119.9, 127.5, 128.0, 128.9, 129.0, 131.0, 132.0, 133.6, 135.1, 136.2, 146.3, 149.2, 165.4, 168.1.
1.1.2. N-(2-Phenyl-4,7-dimethoxybenzothiazol-6-yl)benzamide (7b).
Green finely divided crystals (73%); mp 153-155 oC (MeOH); Anal. Calcd for C22H18N2O3S: C, 67.67; H, 4.65; N, 7.17%. Found: C, 67.48; H, 4.63; N, 7.36%; IR νmax/cm-1 3419, 2820, 1671, 1522; 1H NMR 4.07 (3H, s, OCH3), 4.18 (3H, s, OCH3), 7.53 (6H, m, H-3′,4′,5′ and H-3″,4″,5″), 7.91 (2H, dd, J = 8, 2 Hz, H-2′, 6′), 8.12 (2H, m, H-2″, 6″), 8.32 (1H, s, H-6), 8.67 (1H, s, N-H); 13C NMR δ 56.5, 59.9, 100.9, 127.0, 127.3, 127.6, 128.7, 128.9, 129.0, 130.8, 132.1, 133.2, 134.8, 135.9, 141.4, 150.0, 165.4, 165.5.
1.1.3. N,N′-[2,5-Dimethoxylphenyl]-1,4-dibenzamide (9).
Light brown needles (70%); mp 218-220 oC (MeOH); Anal. Calcd for C22H20N2O4: C, 70.20; H, 5.36; N, 7.44%. Found: C, 70.60; H, 5.56; N, 7.63%; IR νmax/cm-1 3326, 2824, 1660, 1540; 1H NMR 3.95 (6H, s, 2 x OCH3), 7.51 (6H, m, H-3′,4′,5′ and H-3″,4″,5″), 7.89 (4H, d, J = 8 Hz, H-2′, 6′ and H-2″, 6″), 8.43 (2H, s, H-3, 6), 8.63 (2H, s, 2 x N-H); 13C NMR δ 56.5, 103.2, 123.4, 127.0, 128.9, 131.8, 135.1, 141.9, 165.1.
1.2. General procedure for the thionation of benzanilides
To a solution of the benzanilide (1.0 g) in dry toluene (40 mL) was added Lawesson’s reagent (0.6 molar equiv.). The mixture was heated at reflux under an atmosphere of nitrogen for 2-6 h, after which it was concentrated and purified by column chromatography or recrystallized from MeOH or EtOAc-hexanes to give finely divided yellow crystals.
1.2.1. N-(2-Phenyl-4,7-dimethoxybenzothiazol-5-yl)thiobenzamide (1a).
(96% from 7a); mp. 160-161 oC (MeOH); Anal. Calcd for C22H18N2O2S2: C, 65.00; H, 4.46; N, 6.89%. Found: C, 65.17; H, 4.43, N, 6.78%; IR νmax/cm-1 3361, 2927, 1607, 1522, 1362; 1H NMR 4.02 (3H, s, OCH3), 4.36 (3H, s, OCH3), 7.45 (6H, m, H-3′,4′,5′ and H-3″,4″,5″), 7.91 (2H, d, J = 8 Hz, H-2′, 6′), 8.15 (2H, m, H-2″, 6″), 8.96 (1H, s, H-6), 9.96 (1H, s, N-H); 13C NMR δ 55.5, 62.6, 100.3, 122.4, 126.8, 127.6, 128.8, 129.1, 129.7, 129.9, 131.2, 133.4, 138.4, 144.0, 146.2, 148.3, 168.2, 196.2.
1.2.2. N-(2-Phenyl-4,7-dimethoxybenzothiazol-6-yl)thiobenzamide (1b).
(96% from 7b); mp. 165-167 oC (MeOH); Anal. Calcd for C22H18N2O2S2: C, 65.00; H, 4.46; N, 6.89%. Found: C, 65.13; H, 4.49; N, 7.13%; IR νmax/cm-1 3369, 3005, 1592, 1341; 1H NMR 4.03 (3H, s, OCH3), 4.16 (3H, s, OCH3), 7.51 (6H, m, H-3′,4′,5′ and H-3″,4″,5″), 7.89 (2H, s, H-2′, 6’), 8.15 (2H, m, H-2″, 6″), 8.86 (1H, s, H-5), 9.71 (1H, s, N-H); 13C NMR δ 55.5, 62.6, 100.3, 122.4, 126.8, 127.6, 128.8, 129.7, 129.9, 131.2, 131.2, 133.4, 138.4, 144.0, 146.2, 148.3, 168.2, 196.2.
1.2.3. N,N′-(2,5-Dimethoxyphenyl)-1,4-dithiobenzamide (1c).
(90% from 9); mp. 223-225 oC (MeOH); Anal. Calcd for C22H20N2O2S2: C, 64.68; H, 4.93; N, 6.86%. Found: C, 64.82; H, 4.97; N, 7.00%; IR νmax/cm-1 3327, 2994, 1538, 1357; 1H NMR 3.95 (6H, s, 2 x OCH3), 7.45 (6H, m, H-3′,4′,5′ and H-3″,4″,5″), 7.86 (4H, d, J = 8 Hz, H-2′, 6′ and H-2″, 6″), 9.43 (2H, s, H-3, 6), 9.87 (2H, s, 2 x N-H); 13C NMR δ 56.8, 103.8, 126.1, 126.7, 128.8, 131.2, 142.6, 144.2, 195.0.
1.3. General procedure for the Jacobson Synthesis
To the thiobenzanilide (0.4 g) in EtOH (0.5 mL) was added 30% aqueous NaOH solution (8 molar equiv.). The mixture was diluted with water to provide a final solution / suspension in 10% aqueous NaOH. Aliquots (0.5 mL) of this mixture were added at 1 minute intervals to a stirred solution of K3Fe(CN)6 (4 molar equiv.) in water at 90 oC. The reaction mixture was then heated at reflux for a further 2 h and allowed to cool to room temperature. The product was collected by filtration, washed with water and recrystallized from MeOH or CH2Cl2-hexanes to give the corresponding benzothiazoles. Column chromatography was done when necessary using CH2Cl2: hexanes – 3:1 as eluent.
1.3.1. 5-Methoxy-2,7-diphenylbenzo[1,2-d; 3,4-d]bisthiazole (3a).
Off-white crystals (73% from 1a); mp. 235-236 oC (MeOH); Anal. Calcd for C21H14N2OS2: C, 67.35; H, 3.77; N, 7.48%. Found: C, 67.54; H, 3.93; N, 7.43%; IR νmax/cm-1 3006, 1556, 1329; 1H NMR 4.03 (3H, s, OCH3), 7.51 (7H, m, H-6, H-3′,4′,5′ and H-3″,4″,5″), 8.15 (4H, m, H-2′, 6′ and H-2″, 6″); 13C NMR δ 56.3, 100.2, 121.2, 122.6, 127.3, 127.5, 129.1, 130.8, 131.2, 133.5, 133.8, 147.8, 153.6, 154.6, 168.3, 170.1.
1.3.2. 4-Methoxy-2,7-diphenylbenzo[1,2-d; 4,3-d]bisthiazole (3b).
Yellow-green needles (64% from 1b and 15% from 1c); mp. 225-227 oC (EtOH); Anal. Calcd for C21H14N2OS2: C, 67.35; H, 3.77; N, 7.48%. Found: C, 67.32; H, 4.05; N, 7.30%; IR νmax/cm-1 3000, 1554, 1346; 1H NMR 4.16 (3H, s, OCH3), 7.48 (m, 6H, H-3′,4′,5′ and H-3″,4″,5″), 7.57 (1H, s, H-5), 8.14 (4H, m, H-2′, 6′ and H-2″, 6″); 13C NMR δ 56.4, 101.8, 119.3, 127.3, 127.6, 129.0, 129.1, 131.0, 133.2, 133.4, 143.6, 153.0, 153.1, 166.4, 167.8.
1.3.3. 4,8-Dimethoxy-2,6-diphenylbenzo[1,2-d; 4,5-d]bisthiazole (2b).
Yellow crystals (10% from 1b) mp. 274-276 oC (MeOH); Anal. Calcd for C22H16N2O2S2·½H2O: C, 63.92; H, 4.12; N, 6.78%. Found: C, 64.24; H, 3.93; N, 6.73%. IR νmax/cm-1 2820, 1481, 1349, 1261; 1H NMR 4.51 (6H, s, OCH3), 7.51 (6H, m, H-3′,4′,5′ and H-3″,4″,5″), 8.14 (4H, dd, J = 7, 3 Hz, H-2′, 6′ and H-2″, 6″); 13C NMR δ 61.7, 126.7, 127.6, 129.0, 130.1, 133.7, 142.4, 142.9, 166.9.
1.3.4. N-(5-Methoxy-2-phenylbenzothiazol-6-yl)thiobenzamide (1d)
Yellow needles (23% from 1c); mp. 137-140 oC (EtOH); Anal. Calcd for C21H16N2OS2: C, 66.99; H, 4.28; N, 7.44%. Found: C, 67.27; H, 4.01; N, 7.41%; IR νmax/cm-1 3350, 2944, 1573, 1510; 1H NMR 4.04 (3H, s, OCH3), 7.50 (7H, m, H-4, H-3′4′,5′ and H-3″,4″,5″), 7.88 (2H, dd, J = 8, 2 Hz, H-2′, 6′), 8.07 (2H, m, H-2″, 6″), 9.93 (1H, s, H-7), 10.06 (1H, s, N-H); 13C NMR δ 56.4, 103.8, 112.6, 115.4, 126.7, 127.3, 127.7, 128.8, 129.1, 130.9, 131.1, 133.6, 144.1, 149.4, 151.8, 169.5, 195.6.
1.3.5. N-(5-Methoxy-2-phenylbenzothiazol-6-yl)benzamide (4d)
Finely divided yellow crystals (23% from 1c); mp. 182-184 oC (MeOH); Anal. Calcd for C21H16N2O2S: C, 69.98; H, 4.47; N, 7.77%. Found: C, 69.77; H, 4.38; N, 7.69; IR νmax/cm-1 3452, 2949, 2848, 1668, 1571; 1H NMR 4.04 (3H, s, OCH3), 7.50 (7H, m, H-4, H-3′,4′,5′ and H-3″,4″,5″), 7.88 (2H, dd, J = 8, 2 Hz, H-2′, 6′), 8.07 (2H, m, H-2″, 6″), 8.76 (1H, s, H-7), 9.22 (1H, s, N-H); 13C NMR δ 56.3, 103.6, 111.3, 126.7, 127.0, 127.2, 127.7, 128.8, 129.0, 130.7, 131.9, 133.7, 135.0, 148.3, 150.3, 165.3, 168.2.
1.4. General procedure for the AIBN-induced Cyclizaton of thiobenzanilides
To a solution of the thiobenzanilide (0.1 g) in nitrobenzene (5 mL) was added AIBN (1.2 molar equiv.) and the mixture stirred at reflux for 30 min. The crude product was eluted through a silica column, first with hexanes to remove the nitrobenzene followed by EtOAc. The EtOAc fraction was concentrated and recrystallized from EtOAc-hexanes or EtOH.
1.4.1. 8-Methoxy-2,7-diphenylbenzo[2,3-d: 4,5-d]bisthiazole (3a).
Off-white crystals (86% from 1a).
1.4.2. 4-Methoxy-2,7-diphenylbenzo[2,3-d: 4,5-d]bisthiazole (3b).
Yellow-green needles (74 % from 1b, 25% from 1c; and 45% from 1d).
1.4.3. 5-Methoxy-6-thiobenzamidobenzothiazole (1d)
Yellow needles (23% from 1c).
ACKNOWLEDGEMENTS
Financial support of ND-R by a University of the West Indies (UWI) Research Fellowship is gratefully acknowledged.
References
1. E. Kashiyama, I. Hutchinson, M. Chua, S. F. Stinson, L. R. Phillips, G. Kaur, E. A. Sausville, T. D. Bradshaw, A. D. Westwell, and M. F. G. Stevens, J. Med. Chem., 1999, 42, 4172; CrossRef I. Hutchinson, M. Chua, H. L. Browne, V. Trapani, T. D. Bradshaw, A. D. Westwell, and M. F. G. Stevens, J. Med. Chem., 2001, 44, 1446; CrossRef I. Hutchinson, S. A. Jennings, B. R. Vishnuvajjala, A. D. Westwell, and M. F. G. Stevens, J. Med. Chem., 2002, 45, 744; CrossRef C. A. Mathis, Y. Wang, D. P. Holt, G. Huang, M. L. Debnath, and W. E. Klunk, J. Med. Chem., 2003, 46, 2740. CrossRef
2. F. Stephens and D. Wibberley, J. Chem. Soc., 1950, 3336. CrossRef
3. E. Cullen, R. Becker, K. Freter, T. LeClerq, G. Possanza, and H. Wong, J. Med. Chem., 1992, 35, 350. CrossRef
4. D. Meurer, R. Hutzler, J. Fitch, and P. Cassidy, Macromolecules, 1994, 27, 1303. CrossRef
5. H. Hugerscoft, Chem. Ber., 1901, 34, 3130; CrossRef H. Hugerscoft, Chem. Ber., 1903, 36, 3121; CrossRef A. F. Jordan, C. Luo, and A. B. Reitz, J. Org. Chem., 2003, 68, 8693. CrossRef
6. S. Mukai, J. Polymer Science and Technology, 1997, 10, 83; A. G. Green and A. G. Perkin, J. Chem. Soc., 1903, 83, 1201; CrossRef S. L. Solar, R. J. Cox, and N. Clecak, J. Org. Chem., 1968, 33, 2132. CrossRef
7. N. K. Downer and Y. A. Jackson, Org. Biomol. Chem., 2004, 2, 3039. CrossRef
8. Y. A. Jackson, M. A. Lyon, N. Townsend, K. Bellabe, and F. Soltanik, J. Chem. Soc., Perkin Trans. 1, 2000, 205. CrossRef
9. P. Jacobson, Chem. Ber., 1886, 19, 1067. CrossRef