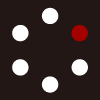
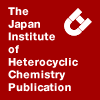
HETEROCYCLES
An International Journal for Reviews and Communications in Heterocyclic ChemistryWeb Edition ISSN: 1881-0942
Published online by The Japan Institute of Heterocyclic Chemistry
e-Journal
Full Text HTML
Received, 26th March, 2010, Accepted, 22nd April, 2010, Published online, 23rd April, 2010.
DOI: 10.3987/COM-10-11954
■ Synthesis of 4-Aryl-1H-pyrazoles by Suzuki-Miyaura Cross Coupling Reaction between 4-Bromo-1H-1-tritylpyrazole and Arylboronic Acids
Hayato Ichikawa, Miho Nishioka, Masao Arimoto, and Yoshihide Usami*
Osaka University of Pharmaceutical Sciences, 4-20-1 Nasahara, Takatsuki, Osaka 569-1094, Japan
Abstract
A general procedure for the synthesis of 4-aryl-1H-pyrazoles by the Suzuki-Miyaura cross coupling reaction between 4-bromo-1H-1-tritylpyrazole and commercially available arylboronic acids was developed. Using this procedure, a direct synthesis of 4-aryl-1H-pyrazoles possessing functional groups, such as hydroxyl, nitro, and amino groups, on the aryl ring was realized. Those molecules could not be prepared by our previous synthesis of 4-aryl-1H-pyrazoles via the Kumada cross coupling reaction.The exploration of new methods for the synthesis of heterocyclic compounds is important work for synthetic organic chemists as most drugs or bioactive compounds possess a heterocyclic part in their molecules. Pyrazoles are one of the most important heterocyclic compounds.1,2 There are a lot of well-known pyrazole-containing drugs, including celecoxib,3,4 rimonobant,5 and 4-methylpyrazole (fomepizole).6,7 Extensive studies of the synthesis of substituted or functionalized pyrazoles have been carried out.8,9 However, most of them deal with the construction of a pyrazole ring by the 2+3 cycloaddition of substituted parts.
The discovery of a novel binuclear platinum (II) complex bearing 4-methylpyrazole as the ligand (4mpz), which exhibits enhanced antitumor activity in cisplatin-resistant L1210/R cell lines,10 has fueled our interest in the direct functionalization of pyrazoles at C-4. Pardo and co-workers reported the synthesis of 4-aryl-1H-pyrazoles via the Stille coupling reaction, as illustrated in Scheme 1 (eq. 1).11 In that procedure, toxic tributyltin chloride was required for the preparation of cross-coupling substrates and the chemical yields were not satisfactory. Another example is the Suzuki coupling (eq. 2) reported by Fu and co-workers.12 In this case, relatively expensive tricyclopentylpalladium was required as an additive for the reaction and 1-benzyl-4-phenyl-1H-pyrazole (1a’) was obtained in 64% yield. This approach is closely related to a series of Harrity’s synthetic studies on functionalized pyrazoles.13-16 Meanwhile, we have developed a method for the synthesis of 4-arylpyrazoles via the Kumada coupling with only 0.2 mol% palladium catalyst, as shown in eq. 3.17 This procedure is quite efficient in that 4-phenyl-1H-1-tritylpyrazole (1a) was synthesized in 99% yield, but has a drawback in that it is difficult to prepare Grignard reagents at the same time. Then, we attempted to perform an alternative procedure where we synthesized 4-aryl-1H-pyrazoles under milder conditions. Herein we describe a simple method for the synthesis of 4-aryl-1H-pyrazoles via the Suzuki-Miyaura coupling reaction18 between 4-bromo-1H-1-tritylpyrazole and commercially available arylboronic acids.
Commercially available pyrazole was converted into 4-bromo-1H-1-tritylpyrazole (3) via 2 using a known method.11,17 Compound 3 was reacted with several commercially available boronic acids in the presence of 5 mol% palladium acetate, triphenylphosphine, and potassium carbonate under reflux in THF. The results of the Suzuki-Miyaura cross coupling reaction are summarized in Table 1. The reaction with phenylboronic acid resulted in 92% yield of 1a (entry 1), which is slightly lower than that obtained with
our previous method10 but better than that obtained with the reverse type of the Suzuki-Miyaura coupling reaction.9 Using this procedure, molecules that could not be obtained by the previous method were prepared. The reaction with arylboronic acids bearing a hydroxyl or an amino group proceeded, as seen in entries 2, 3, 6, and 7. Increasing the amount of the palladium catalyst was not effective, as shown by the results of entries 6 and 7. Although 4-(3-nitrophenyl)-1H-1tritylpyrazole (1e) could not be synthesized in our previous work,17 it was synthesized in this study albeit the low yield (entry 5). Other heteroarylboronic acids coupled with pyrazoles, as shown in entries 8-11. The Suzuki-Miyaura coupling reaction between B-(1H-1-tritylpyrazol-4-yl)boronic acid (4), which was prepared along Scheme 3, and 2-bromothiophene gave 4-(thiophen-2-yl)-1H-1-tritylpyrazole (1g) as shown in Scheme 4 in lower yield compared to entries 8, 9 in Table 1 under similar reaction conditions. Protection with trityl group at N-1 is necessary in this study since the desired coupled product could not been obtained under the same reaction condition when 2 was used as a coupling partner to phenylboronic acid. This result agreed to Fu’s work12 that the Suzuki-Miyaura coupling reaction between B-(1H-pyrazol-4-yl)boronic acid and chlorobenzene gave the desired product in lower yield (8%) than the corresponding reaction (64%) shown in eq. 2 (Scheme 1).10
In summary, the Suzuki-Miyaura coupling reaction between 4-bromo-1H-1-tritylpyrazole and arylboronic acids was found to afford various kinds of 4-aryl-1H-pyrazoles. A direct preparation of 4-aryl-1H-pyrazoles with hydroxyl, nitro, or amino groups on the aryl ring resulted in relatively low yields. Nevertheless, this procedure is quite simple and practical as commercially available arylboronic acids can be used.
EXPERIMENTAL
IR spectra were obtained with a JEOL FT/IR-680 Plus spectrometer. HRMS was determined with a JEOL JMS-700 (2) mass spectrometer. NMR spectra were recorded at 27 ℃ on Varian UNITY INOVA-500 and Mercury-300 spectrometers in CDCl3 with tetramethylsilane (TMS) as internal standard. Melting points were determined on a Yanagimoto micromelting point apparatus and are uncorrected. Liquid column chromatography was conducted over silica gel (Nacalai, silica gel 60, 70−230 mesh or 230−400 mesh). Analytical TLC was performed on precoated Merck glass plates (silica gel 60 F254) and compounds were detected by dipping the plates in an ethanol solution of phosphomolybdic acid, followed by heating. Dry THF was distilled over sodium benzophenone ketyl under nitrogen atmosphere.
4-Bromo-1H-pyrazole (2)
To a solution of pyrazole (6.8 g, 100 mmol) in CH2Cl2 (120 mL) was added bromine (5.1 mL, 100 mmol) at 0 ℃ with stirring. After stirring overnight, the reaction mixture was treated with NaHSO4 aq. and extracted with CH2Cl2. The organic layer was washed with brine, dried over MgSO4, and filtered. The solvent as removed under reduced pressure to give a crude residue, which was purified by recrystallization to afford 2 (14.7 g, 100%). 2: Colorless crystals; 1H-NMR (300 MHz, CDCl3): δ 6.97 (1H, s, pyrazole-H), 7.60 (1H, s, pyrazole-H).
4-Bromo-1H-1-tritylpyrazole (3)
To a solution of 2 (5.1 g, 35 mmol) in dry Et2O (350 mL) with Et3N (5.9 mL, 42 mmol) was added trityl chloride (12g, 42 mmol) under nitrogen atmosphere. After stirring for 1 hr at rt, the reaction mixture was quenched by the addition of water. The organic layer was washed with brine, dried over MgSO4, and filtered. The solvent was removed under reduced pressure to give a crude residue, which was purified by recrystallization to afford 3 (12 g, 85%). 3: Colorless crystals; 1H-NMR (300 MHz, CDCl3): δ 7.08−7.36 (15 H, m, Ph-H), 7.37 (1H, s, pyrazole-H), 7.61 (1H, s, pyrazole-H).
Synthesis of 4-aryl-1H-1-tritylpyrazoles (Table 1, Scheme 4)
General procedure: To a solution of 3 (389 mg, 1.0 mmol) in THF (5.0 mL) were added phenylboronic acid (293 mg, 2.4 mmol), Pd(OAc)2 (225 mg, 0.05 mmol), triphenylphosphine (20 mg, 0.22 mmol), and K2CO3 (415 mg, 3.0 mmol). The reaction mixture was stirred under reflux overnight. The reaction mixture was quenched by the addition of dil. HCl aq. and extracted with EtOAc. The organic layer was washed with brine, dried over MgSO4, and filtered. The solvent was removed under reduced pressure to give a crude residue, which was purified by column chromatography (hexane:EtOAc = 20:1) to afford 1a (360 mg, 92%).
4-Phenyl-1H-1-tritylpyrazole9 (1a): 1H-NMR (300 MHz, CDCl3): δ 7.94 (1H, s, pyrazole-H), 7.61 (1H, s, pyrazole-H), 7.14–7.45 (20H, m, Tr-H, Ph-H).
4-(4-Hydroxyphenyl)-1H-1-tritylpyrazole8 (1b): 1H-NMR (300 MHz, CDCl3): δ 7.84 (1H, d, J = 0.8 Hz, pyrazole-H), 7.52 (1H, d, J = 0.8 Hz, pyrazole-H), 7.32–7.17 (15H, m, Tr-H), 7.25 (2H, d, J = 8.7 Hz, Ar-H), 6.74 (2H, d, J = 8.7 Hz, Ar-H), 5.04 (1H, brs, OH); 13C-NMR (75 MHz, CDCl3): δ 153.7, 142.6, 136.5, 129.8, 128.1, 127.4, 126.5, 124.9, 121.0, 115.4, 78.7 (lack of a carbon signal corresponding to the trityl-quaternary carbon, probably because of overlap with one of the chloroform signals); HRMS m/z calcd for C28H22N2O (M+) 402.1732, found 402.1731.
4-(3-Hydroxyphenyl)-1H-1-tritylpyrazole (1c): Colorless crystals; mp 220−222 ℃; IR (KBr) νmax 3272 (OH), 1610 (C=C), 1598 (C=C) cm-1; 1H-NMR (300 MHz, CDCl3): δ 7.89 (1H, d, J = 0.7 Hz, pyrazole-H), 7.59 (1H, d, J = 0.7 Hz, pyrazole-H), 7.33–7.28 (9H, m, Tr-H), 7.21–7.16 (6H, m, Tr-H), 7.15 (1H, t, J = 8.8 Hz, Ar-H), 6.98 (1H, dt, J = 7.7, 1.5 Hz, Ar-H), 6.84 (1H, t, J = 2.4 Hz, Ar-H), 6.60 (1H, ddd, J = 8.1, 2.6, 0.9 Hz, Ar-H), 5.08 (1H, brs, OH); 13C-NMR (75 MHz, CDCl3): δ 155.4, 142.5, 136.8, 129.7, 129.6, 128.9, 127.4, 120.9, 117.6, 113.1, 112.1, 78.8 (lack of a carbon signal probably because of overlap with one of the chloroform signals); HRMS m/z calcd for C28H22N2O (M+) 402.1732, found 402.1729.
4-(2-Tolyl)-1H-1-tritylpyrazole (1d): Colorless crystals; mp 100−103 ℃; IR (KBr) νmax 1602 (C=C), 1563 (C=C) cm−1; 1H-NMR (300 MHz, CDCl3): δ 7.81 (1H, d, J = 0.7 Hz, pyrazole-H), 7.46 (1H, d, J = 0.7 Hz, pyrazole-H), 7.34–7.28 (10H, m, Tr-H, Tol-H), 7.25–7.1 (9H, m, Tr-H, Tol-H), 2.38 (3H, brs, CH3); 13C-NMR (75 MHz, CDCl3): δ 143.2, 139.2, 135.2, 132.2, 131.3, 130.6, 130.2, 129.0, 127.9, 127.7, 126.7, 125.9, 120.7, 78.6, 21.2; MS (EI) m/z 400 (M+), 243 (100%); HRMS m/z calcd for C29H24N2 (M+) 400.1940, found 400.1934.
4-(3-Nitrophenyl)-1H-1-tritylpyrazole (1e): Colorless crystals; mp 202−204 ℃; IR (KBr) νmax 1570 (C=C), 1528 (ArNO2) cm−1; 1H-NMR (300 MHz, CDCl3): δ 8.24 (1H, t, J = 2.2 Hz, 2’-H), 8.04 (1H, ddd, J = 8.0, 2.4, 1.1 Hz, 3’- or 6’-H), 8.00 (1H, d, J = 0.6 Hz, pyrazole-H), 7.75 (1H, br d, J = 8.0 Hz, 3’- or 6’-H), 7.74 (1H, d, J = 0.6 Hz, pyrazole-H), 7.49 (1H, t, J = 8.0 Hz, 2’-H), 7.38–7.32 (10H, m, Tr-H), 7.24–7.14 (5H, m, Tr-H); 13C-NMR (75 MHz, CDCl3): δ 148.7, 142.7, 137.3, 134.5, 131.3, 130.1, 129.7, 129.6, 128.0, 127.9, 120.9, 120.1, 119.6, 79.2; MS (EI) m/z 431 (M+), 243 (100%); HRMS m/z calcd for C28H21N3O2 (M+) 431.1634, found 431.1638.
4-(3-Aminophenyl)-1H-1-tritylpyrazole (1f): Colorless crystals; mp 155−157 ℃; IR (KBr) νmax 3380 (N-H), 1617 (C=C), 1588 (C=C) cm−1; 1H-NMR (300 MHz, CDCl3): δ 7.89 (1H, d, J = 0.7 Hz, pyrazole-H), 7.57 (1H, d, J = 0.7 Hz, pyrazole-H), 7.38–7.31 (9H, m, Tr-H), 7.27–7.19 (6H, m, Tr-H), 7.09 (1H, t, J = 7.7 Hz, Ar-5H), 6.82 (1H,br d, J = 7.7, Hz, Ar-4H), 6.74 (1H,br s, Ar-2H), 6.52 (1H,dd, J = 7.7, 2.4 Hz, Ar-6H), 3.64 (2H, brs, -NH2); 13C-NMR (75 MHz, CDCl3): δ 146.8, 143.1, 137.3, 133.5, 130.2, 129.7, 129.2, 127.8, 121.7, 116.0, 113.3, 112.1; MS (EI) m/z 401 (M+), 243 (100%); HRMS m/z calcd for C28H23N3 (M+) 401.1892, found 401.1885.
4-(Thiophen-2-yl)-1H-1-tritylpyrazole10 (1g): 1H-NMR (200 MHz, CDCl3): δ 7.84 (1H, d, J = 0.8 Hz, pyrazole-H), 7.55 (1H, d, J = 0.8 Hz, pyrazole-H), 7.33–7.28 (9H, m, Tr-H), 7.23–7.15 (6H, m, Tr-H), 7.08 (1H, dd, J = 5.0, 1.3 Hz, thienyl-5H), 6.99 (1H, dd, J = 3.6, 1.3 Hz, thienyl-3H), 6.94 (1H, dd, J = 5.0, 3.6 Hz, thienyl-4H).
4-(Thiophen-3-yl)-1H-1-tritylpyrazole (1h): Colorless crystals; mp 203−205 ℃; IR (KBr) νmax 1595 (C=C) cm−1; 1H-NMR (300 MHz, CDCl3): δ 7.88 (1H, d, J = 0.7 Hz, pyrazole-H), 7.56 (1H, d, J = 0.7 Hz, pyrazole-H), 7.36–7.12 (9H, m, Ar-H); 13C-NMR (75 MHz, CDCl3): δ 143.1, 137.5, 130.1, 129.1, 127.9, 127.8, 127.8, 127.2, 126.1, 126.0, 118.2, 78.7; MS (EI) m/z 392 (M+), 243 (100%); HRMS m/z calcd for C26H20N2S (M+) 392.1347, found 392.1345.
4-(Fur-2-yl)-1H-1-tritylpyrazole (1i): Colorless crystals; mp 114−118 ℃; IR (KBr) νmax 1610 (C=C), 1598 (C=C) cm−1; 1H-NMR (200 MHz, CDCl3): δ 7.87 (1H, s, pyrazole-H), 7.61 (1H, s, pyrazole-H), 7.38–7.13 (16H, m, Tr-H, furyl-H), 6.40 (1H, dd, J = 3.6, 1.9 Hz, furyl-H), 6.31 (1H, d, J = 3.6 Hz, furyl-H); 13C-NMR (75 MHz, CDCl3): δ 146.8, 142.9, 140.6, 136.5, 130.1, 127.9, 127.8, 127.7, 127.2, 111.2, 103.7 (lack of a carbon signal probably because of overlap with one of the chloroform signals); MS (EI) m/z 376 (M+), 243 (100%); HRMS m/z calcd for C26H20N2O (M+) 376.1576, found 376.1579.
B-(1H-1-tritylpyrazol-4-yl)boronic acid (4) (Scheme 2)
To a suspension of 3 (19.6 g, 50 mmol) in Et2O (250 mL) was added 1.6 M n-BuLi in hexane (35 mL, 55 mmol) at −78 ℃ under nitrogen atmosphere. After stirring for 30min, B(OMe)3 (6.2 mL, 56mmol) was added. The reaction mixture was treated with 1N HCl aq., then extracted with EtOAc. The organic layer was separated, dried over MgSO4, filtered and evaporated to give a crude residue, which was purified by column chromatography (eluent; MeOH:CH2Cl2= 100:1) to afford 4 (10.5g, 59%). 4: Colorless crystals; mp 85−87 ℃; IR (KBr) νmax 3308 (OH), 1597 (C=C), 1536 (C=C) cm−1; 1H-NMR (200 MHz, CDCl3): δ 7.92 (1H, s, pyrazole-H), 7.77 (1H, s, pyrazole-H), 7.34–7.28 (9H, m, Tr-H), 7.18–7.12 (6H, m, Tr-H); 13C-NMR (75 MHz, CDCl3): δ 145.1, 142.9, 138.7, 130.1, 130.0, 127.8, 127.6, 78.8; MS (FAB) m/z 310 ([M−BO2H]+), 309 ([M−B(OH)2]+), 243 (100%); HRFABMS m/z calcd for C22H18N2 ([M−BO2H]+) 310. 1470, found 310.1475, m/z calcd for C22H17N2 ([M−B(OH)2]+) 309.1392. 1470, found 309.1389.
ACKNOWLEDGEMENTS
We are grateful to Ms. M. Fujitake of this University for MS measurements. This work was supported in part by a Grant-in-Aid for “High-Tech Research Center” Project for Private Universities: matching fund subsidy from MEXT (Ministry of Education, Culture, Sports, Science, and Technology), 2006−2009, Japan.
References
1. J. Elguero, P. Goya, N. Jagerovic, and A. M. S. Silva, Targets in Heterocyclic Systems, 2002, 6, 52.
2. C. Lamberth, Heterocycles, 2007, 71, 1467. CrossRef
3. J.-M. Dogne, J. Hanson, C. Supuran, and D. Pratico, Curr. Pharm. Des., 2006, 12, 971. CrossRef
4. J. P. O'Connor and T. Lysz, Drugs of Today, 2008, 44, 693.
5. J. H. M. Lange and C. G. Kruse, Chemical Record, 2008, 8, 156. CrossRef
6. J. Likforman, A. Brouard, C. Philippe, C. Bismuth, and E. J. Postaire, Toxicol. Clinique et Experimentale, 1987, 7, 373.
7. N. De Brabander, M. Wojciechowski, K. De Decker, A. De Weerdt, and P. G. Jorens, Euro. J. Pediatrics, 2005, 164, 158. CrossRef
8. B. Stanovnik and J. Svete, Science of Synthesis, 2002, 12, 15.
9. S. Fustero, A. Simon-Fuentes, and J. F. Sanz-Cervera, Organic Preparations and Procedures International, 2009, 41, 253. CrossRef
10. T. Kanai, R. Komaki, T. Sato, S. Komeda, Y. Saito, and M. Chikuma, Yakugaku Zasshi, 2007, 127, Suppl. 2, 51.
11. J. Elguero, C. Jaramillo, and C. Pardo, Synthesis, 1997, 563. CrossRef
12. N. Kudo, M. Perseghini, and G. C. Fu, Angew. Chem. Int. Ed., 2006, 45, 1282. CrossRef
13. D. N. Browne, M. D. Helm, A. Plant, and J. P. A. Harrity, Angew. Chem. Int. Ed., 2007, 46, 8656. CrossRef
14. D. N. Browne, J. B. Taylor, A. Plant, and J. P. A. Harrity, J. Org. Chem., 2009, 74, 396. CrossRef
15. D. N. Browne, J. F. Vivat, A. Plant, E. Gomez.-Bengoa, and J. P. A. Harrity, J. Am. Chem. Soc., 2009, 131, 7762. CrossRef
16. R. S. Foster, J. Huang, J. F. Vivat, D. L. Browne, and J. P. A. Harrity, Org. Biomol. Chem., 2009, 7, 4052. CrossRef
17. H. Ichikawa, Y. Ohno, Y. Usami, and M. Arimoto, Heterocycles, 2006, 68, 2247. CrossRef
18. A. Suzuki, Handbook of Organopalladium Chemistry for Organic Synthesis (Ed. by E. Negishi), Wiley Interscience, New York, 2002, chap. III.2.2.