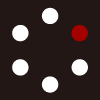
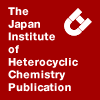
HETEROCYCLES
An International Journal for Reviews and Communications in Heterocyclic ChemistryWeb Edition ISSN: 1881-0942
Published online by The Japan Institute of Heterocyclic Chemistry
e-Journal
Full Text HTML
Received, 15th May, 2010, Accepted, 14th June, 2010, Published online, 16th June, 2010.
DOI: 10.3987/COM-10-S(E)32
■ Efficient Transformation of Flav-3-enes Using Reductive Elimination of Flav-4-triflate
Yoshihito Kohari, Yukio Hoshino, Haruo Matsuyama, and Hiroto Nakano*
Biological function Research Unit, College of Environmental Technology, Muroran Institute of Technology, 27-1 Mizumoto, Muroran, Hokkaido 050-8585, Japan
Abstract
Flav-3-enes were prepared in excellent yields (up to 98%) by reductive elimination of flav-3-ene-4-triflates in the presence of palladium acetate, formic acid and tri-n-buthylamine.Flav-3-enes (3-phenyl-2H-1-benzopyrans) 1 are the useful intermediates of naturally occurring flavonoids such as flavones (2-phenyl-4H-1-benzopyran-4-ones),1 flavans (2-phenyl-3,4-dihydro-2H-1-benzo- pyrans)2 and flavanols (3-hydroxy-2-phenyl-3,4-dihydro-2H-1-benzopyrans),3 which have anti-allergic, anti-inflammatory,4 anti-microbial5 and anti-cancer6 activities (Scheme 1). It is therefore important to establish an effective synthetic methodology for the ring system 1. Some synthetic methodologies have been reported previously for the synthesis of this ring system.7 In particular, the condensation of α, β-unsaturated carbonyl compounds with phenols under different reaction conditions represents a largely used and promising route.
In addition, the methodology using NaBH4 reduction of flavanone 2, tosylation followed by dehydration of 4-tosylate is also efficient synthetic route.1-3 The problem with these syntheses, however, relates to both their selectivity and their general applicability; moreover in some cases the overall yields are poor. We planed the new synthetic methodology using the conversion from 3 to vinyl triflate 2, followed by the reductive elimination of 2 in the presence of palladium acetate, formic acid and tri-n-buthylamine (Scheme 1).8 We describe in this paper the detail of our new and very simple synthetic methodology of 1 based on the reductive elimination of vinyl triflate 2.
We attempted a conversion from ketones 2a-h to vinyl triflates 3a-h (Table 1). The reaction of the simplest ketone 2a with triflic anhydride was carried out in CH2Cl2 in the presence of pyridine as a base at -78°C. The reaction successfully proceeded to afford the desired triflate 3a in excellent yield (97%, entry 1). The reactions of several ketones 2b-h having substituents on the phenyl group at 2-position or benzopyranone ring system, respectively, were also carried out under the same reaction conditions as those used for 2a. As a result, all of the attempted reactions afforded the desired triflates 3b-h in fairly good to excellent yields (88-98%, entries 2-8).
Next, the reductive eliminations of triflates 3a-h were examined (Table 1). The reactions were carried out in the presence of formic acid, tri-n-butylamine, and three kinds of Pd catalysts such as Pd(OAc)2-dppf, Pd(OAc)2-2PPh3, and Pd(PPh3)4 at 60 °C. The reaction of 3a using Pd(OAc)2-dppf catalyst afforded the desired 1a in an excellent isolated yield (95%) (entry 1). Although the use of Pd(OAc)2-2PPh3 or Pd(PPh3)4 as a catalyst, respectively, also afforded satisfactory yields [Pd(OAc)2-2PPh3: 85%, Pd(PPh3)4: 92%], they did not provide the catalytic activity of Pd(OAc)2-dppf. From the above results, it can be seen that Pd(OAc)2-dppf is a better catalyst for the reductive elimination of triflate 3a. The reactions of other substrates 2b-h were also examined under the same reaction conditions as those used for 3a (entries 2-8). The reactions successively proceeded to afford the desired 1b-h in moderate to excellent yields, as shown in Table 1. Both the reactions using 3b with a methoxy substituent or 3c with a methyl substituent on the phenyl groups as an electron-donating group brought about a decease in chemical yield with a complex mixture, although the reason for this decrease remains unclear (entries 2 and 3). It might be due to the steric and the electric factors of methyl or methoxy substituents. In addition, it might be due to the structural instability9 of 1b and 1c. On the other hand, substrates 3d or 3h with a chlorine substituent on the phenyl group as an electron-withdrawing group, respectively, gave chemoselectively 1d (93%) and 1h (92%), respectively without dechlorination that is observed under these reaction conditions (entries 4 and 8).
In conclusion, we have developed an efficient synthetic methodology for obtaining the flav-3-enes, which are useful intermediates for the synthesis of flavonids. Thus the conversion from flavanones 2 to flav-3-enes 1 via triflates 3 was accomplished in total yields of 61-95% under very mild conditions. This new methodology can be extended to the preparation of still more flav-3-enes having various substituens. Further studies to examine the scope and limitations of our new synthetic methodology for the synthesis of flavonoids are now in progress.
EXPERIMENTAL
All reactions were carried out in anhydrous solvents and under nitrogen atmosphere. Flavanones were prepared from commercially available acetophenones and benzaldehydes according to well-known method. 1H and 13C NMR spectra were recorded at 270 MHz and 67.8 MHz on a JEOL JNM-EX 270 FT NMR SYSTEM in CDCl3 using tetramethylsilane as an internal standard. TLC analyses were performed on commercial aluminum plates bearing a 0.25-mm layer of Merck Silica gel 60F254. Silica gel (Wakogel 200 mesh) was used for column chromatography.
General procedure for synthesis of flav-3-ene-4-triflates 3a-h. Flavanone 2a (2.0 mmol) was dissolved in dry CH2Cl2 (5.0 mL) along with dry pyridine (2.4 mmol) under nitrogen. The solution was cooled at -78 °C. 2.4 mmol of trifluoromethanesulfonic anhydride was slowly added. The resulting mixture was allowed to warm to 0 °C, stirred at 0 °C for 1 h, then warmed to room temperature, and stirred until flavanone disappeared by monitoring using TLC. The reaction mixture was extracted with Et2O and the extract was washed with H2O, 1M aq. HCl and brine, and dried over MgSO4. The solvent was removed and the residue was isolated by silica gel column chromatography (hexane/EtOAc 4:1) to afford 3a.
2-Phenyl-4-trifluoromethanesulfonyloxy-2H-1-benzopyrane (3a). Light yellow oil, Rf 0.64 (4:1 hexane/EtOAc), 1H NMR (CDCl3): δ 5.81 (d, 1H, J = 3.8 Hz), 6.07 (d, 1H, J = 3.8 Hz), 6.82 (d, 1H, J = 8.0 Hz), 6.95 (t, 1H, J = 7.6 Hz), 7.21 (td, 1H, J = 8.0, 1.5 Hz), 7.29 (dd, 1H, J = 7.6, 1.5 Hz), 7.34-7.46 (m, 5H), 13C NMR (CDCl3): δ 76.3, 113.1, 116.4, 116.6, 121.6, 121.6, 127.1, 128.3, 128.9, 129.1, 131.8, 138.8, 143.1, 153.8.
3’-Methoxy-2-phenyl-4-trifluoromethanesulfonyloxy-2H-1-benzopyran (3b). A Light yellow oil, Rf 0.59 (4:1 hexane/EtOAc), 1H NMR (CDCl3): δ 3.78 (s, 3H), 5.83 (d, 1H, J = 3.8 Hz), 6.08 (d, 1H, J = 3.6 Hz), 6.85 (d, 1H, J = 8.3 Hz), 6.89-7.04 (m, 3H), 7.22-7.34 (m, 3H), 13C NMR (CDCl3): δ 55.2, 77.1, 112.5, 113.0, 114.8, 116.4, 116.6, 119.3, 121.6, 121.7, 130.0, 131.8, 140.3, 143.2, 153.8, 160.0.
2’,4’-Dimethyl-2-phenyl-4-trifluoromethanesulfonyloxy-2H-1-benzopyran (3c). A light yellow oil, Rf 0.73 (4:1 hexane/EtOAc), 1H NMR (CDCl3): δ 2.30 (s, 3H), 2.44 (s, 3H), 5.76 (d, 1H, J = 3.6 Hz), 6.29 (d, 1H, J = 3.6 Hz), 6.79(dd, 1H, J = 8.3, 1.0 Hz), 6.80 (td, 1H, J = 7.6, 1.0 Hz), 7.00-7.05 (m, 2H), 7.21 (td, 1H, J = 7.6, 1.0 Hz), 7.27-7.33 (m, 2H), 13C NMR (CDCl3): δ 19.1, 21.0, 74.9, 113.1, 116.5, 121.5, 121.6, 127.0, 128.0, 131.7, 132.0, 133.3, 136.3, 139.1, 143.4, 154.1.
3’,4’-Dichloro-2-phenyl-4-trifluoromethanesulfonyloxy-2H-1-benzopyran (3d). A light yellow oil, Rf 0.66 (4:1 hexane/EtOAc), 1H NMR (CDCl3): δ 5.80 (d, 1H, J = 4.0 Hz), 6.07 (d, 1H, J = 4.0 Hz), 6.86 (d, 1H, J = 8.3 Hz), 7.00 (td, 1H, J = 7.6, 1.0 Hz), 7.29 (dd, 1H, J = 8.3, 2.2 Hz), 7.47 (d, 1H, J = 8.3 Hz), 7.55 (d, 1H, J = 2.2 Hz), 13C NMR (CDCl3): δ 75.0, 116.2, 116.7, 121.9, 122.1, 126.3, 129.2, 131.0, 132.1, 133.1, 133.3, 138.9, 143.7, 153.4.
4’-Isopropyl-2-phenyl-4-trifluoromethanesulfonyloxy-2H-1-benzopyran (3e). A light yellow oil, Rf 0.73 (4:1 hexane/EtOAc), 1H NMR (CDCl3): δ 1.23 (d, 6H, J = 6.9 Hz), 2.90 (q, 1H, J = 6.9 Hz), 5.81 (d, 1H, J = 3.8 Hz), 6.60 (d, 1H, J = 3.8 Hz), 6.82 (dd, 1H, J = 8.1, 1.0 Hz), 6.94 (td, 1H, J = 7.6, 1.0 Hz), 7.21 (td, 1H, J = 7.8, 1.5 Hz), 7.24 (d, 2H, J = 8.3 Hz), 7.29 (dd, 1H, J = 7.8, 1.5 Hz), 7.37 (d, 2H, J = 8.3 Hz), 13C NMR (CDCl3): δ 23.8, 33.9, 77.3, 113.2, 116.4, 116.6, 121.5, 121.6, 127.0, 127.3, 131.7, 136.2, 143.1, 150.0, 153.9.
7-Methyl-2-phenyl-4-trifluoromethanesulfonyloxy-2H-1-benzopyran (3f). A light yellow oil, Rf 0.65 (4:1 hexane/EtOAc), 1H NMR (CDCl3): δ 2.23 (s, 3H), 5.75 (d, 1H, J = 3.8 Hz), 6.04 (d, 1H, J = 3.8 Hz), 6.65 (s, 1H), 6.76 (d, 1H, J = 7.8 Hz), 7.16 (d, 1H, J = 7.8 Hz), 7.33-7.45 (m, 5H), 13C NMR (CDCl3): δ 21.5, 77.3, 111.8, 113.8, 121.4, 127.1, 128.9, 129.0, 139.0, 142.7, 143.4, 153.8.
7-Methoxy-2-phenyl-4-trifluoromethanesulfonyloxy-2H-1-benzopyran (3g). A light yellow oil, Rf 0.63 (4:1 hexane/EtOAc), 1H NMR (CDCl3): δ 3.76 (s, 3H), 5.86 (d, 1H, J = 4.0 Hz), 6.03 (d, 1H, J = 3.8 Hz), 6.78-6.83 (m, 3H), 7.35-7.43 (m, 5H), 13C NMR (CDCl3): δ 55.7, 77.1, 106.6, 113.9, 117.1, 117.4, 117.5, 127.1, 128.9, 129.1, 138.7, 143.2, 147.7, 154.3.
6-Chlro-7-methyl-2-phenyl-4-trifluoromethanesulfonyloxy-2H-1-benzopyran (3h). A light yellow oil, Rf 0.65 (7:1 hexane/EtOAc), 1H NMR (CDCl3): δ 2.29 (s, 3H), 5.83 (d, 1H, J = 4.0 Hz), 6.07 (d, 1H, J = 4.0 Hz), 6.72 (s, 1H), 7.23 (s, 1H), 7.37-7.41 (m, 5H); 13C NMR (CDCl3): δ 20.3, 77.5, 113.0, 115.5, 119.0, 121.8, 127.0, 127.1, 129.0, 129.3, 138.5, 140.1, 142.2, 152.2.
General procedure for the synthesis of flav-3-enes (2-phenyl-2H-1-benzopyrans) 1a-h. To a mixture of 3a (1.0 mmol) and tri-n-butylamine (3.0 mmol) in dry DMF (2.0 mL), formic acid (2.0 mmol) was added in the presence of palladium acetate (0.02 mmol) and 1,1’-bis(diphenylphosphino)ferrocene (0.04 mmol) under nitrogen. The resulting mixture was stirred at 60 °C for 1 h. The reactiom mixture was exracted with Et2O and the extract was washed with H2O and brine, and dried over MgSO4. The solvent was removed and the residue was isolated by silica gel column chromatography (hexane/EtOAc 10:1) to afford 1a.
2-Phenyl-2H-1-benzopyran (1a).10 A light yellow oil, Rf 0.55 (4:1 hexane/EtOAc), 1H NMR (CDCl3): δ 5.79 (dd, 1H, J = 9.9, 3.5 Hz), 5.91 (dd, 1H, J = 3.3, 2.0 Hz), 6.53 (dd, 1H, J = 9.8, 1.4 Hz), 6.78 (d, 1H, J = 8.8 Hz), 6.86 (td, 1H, J = 8.0, 1.5 Hz), 7.29 (dd, 1H, J = 7.6, 1.5 Hz), 7.34-7.46 (m, 5H), 13C NMR (CDCl3): δ77.1, 116.0, 121.2, 121.3, 123.9, 124.8, 126.6, 127.0, 128.3, 128.6, 129.4, 140.8, 153.1, HRMS (EI): calcd for C15H12O: 208.0880; found: 208.0892.
3’-Methoxy-2-phenyl-2H-1-benzopyran (1b). A light yellow oil, Rf 0.42 (10:1 hexane/EtOAc); 1H NMR (CDCl3): δ 3.74 (s, 3H), 5.75 (dd, 1H, J = 9.7, 3.3 Hz), 5.85 (dd, 1H, J = 3.3, 2.0 Hz), 6.48 (dd, 1H, J = 9.7, 2.0 Hz), 6.77-7.28 (m, 8H), 13C NMR (CDCl3): δ 55.1, 76.9, 112.5, 113.7, 115.9, 119.2, 121.1, 121.2, 123.9, 124.7, 126.5, 129.4, 129.6, 142.4, 153.1, 159.8, HRMS (EI): calcd for C16H14O2: 238.0994; found: 238.0994.
2’,4’-Dimethyl-2-phenyl-2H-1-benzopyran (1c). A light yellow oil, Rf 0.55 (10:1 hexane/EtOAc), 1H NMR (CDCl3): δ 1.22 (s, 3H), 1.25 (s, 3H), 5.79 (dd, 1H J = 9.9, 3.5 Hz), 5.88 (dd, 1H, J = 3.5, 1.7 Hz), 6.52 (dd, 1H, J = 9.7, 1.6 Hz), 6.77 (d, 1H, J = 7.9 Hz), 6.85 (td, 1H, J = 7.4, 1.2 Hz), 7.00 (dd, 1H, J = 7.4, 1.8 Hz), 7.09 (td, 1H, J = 7.9, 1.8 Hz), 7.21 (m, 3H), 13C NMR (CDCl3): δ 23.9, 33.9, 77.1, 116.0, 116.6, 121.1, 121.3, 123.9, 125.0, 127.0, 127.1, 129.1, 131.7, 138.2, 149.1, 153.2, HRMS (EI): calcd for C17H15O: 235.1124; found: 235.1127.
3’,4’-Dichloro-2-phenyl-2H-1-benzopyran (1d). A light yellow oil, Rf 0.49 (10:1 hexane/EtOAc), 1H NMR (CDCl3): δ 5.69 (dd, 1H J = 9.7, 3.5 Hz), 5.80 (dd, 1H, J = 3.5, 1.5 Hz), 6.39 (dd, 1H, J = 9.7, 1.5 Hz), 6.77 (d, 1H, J = 7.9 Hz), 6.85 (td, 1H, J = 7.3, 1.2 Hz), 6.97 (dd, 1H, J = 7.4, 1.7 Hz), 7.09 (td, 1H, J = 7.8, 1.8 Hz), 7.23 (dd, 1H, J = 8.3, 1.9 Hz), 7.36 (d, 1H, J = 8.3 Hz), 7.50 (d, 1H, J = 1.2 Hz), 13C NMR (CDCl3): δ 75.5, 116.0, 120.9, 121.5, 123.4, 124.7, 126.2, 126.7, 128.9, 129.7, 130.5, 132.2, 132.6, 140.9, 152.6, HRMS (EI): calcd for C15H10OCl2: 276.0109; found: 276.0110.
4’-Isopropyl-2-phenyl-2H-1-benzopyran (1e). A light yellow oil, Rf 0.58 (10:1 hexane/EtOAc), 1H NMR (CDCl3): δ 1.20 (d, 6H, J = 6.9 Hz), 2.85 (q, 1H, J = 6.9 Hz), 5.71 (dd, 1H, J = 9.9, 3.3 Hz), 5.83 (d, 1H, J = 3.3 Hz), 6.45 (d, 1H, J = 8.1 Hz), 6.78 (td, 1H, J = 7.4, 1.2 Hz), 6.94 (dd, 1H, J = 7.3, 1.7 Hz), 7.04 (td, 1H, J = 7.6, 1.8 Hz), 7.18 (d, 2H, J = 8.1 Hz), 7.34 (d, 2H, J = 8.1 Hz), 13C NMR (CDCl3): δ 23.9, 33.8, 77.0, 115.9, 121.0, 121.3, 123.8, 124.9, 126.5, 126.6, 127.1, 129.3, 138.2, 149.0, 153.2, HRMS (EI): calcd for C18H18O: 250.1358; found: 250.1360.
7-Methyl-2-phenyl-2H-1-benzopyran (1f). A light yellow oil, Rf 0.58 (10:1 hexane/EtOAc), 1H NMR (CDCl3): δ 2.24 (s, 3H), 5.70 (dd, 1H, J = 9.9, 3.4 Hz), 5.86 (d, 1H, J = 3.4 Hz), 6.47 (d, 1H, J = 9.9 Hz), 6.61 (s, 1H), 6.65 (d, 1H, J = 7.5 Hz), 6.87 (d, 1H, J = 7.5 Hz), 7.28-7.44 (m, 5H), 13C NMR (CDCl3): δ 21.4, 77.1, 116.6, 118.6, 121.8, 123.7, 123.8, 126.3, 127.0, 128.2, 128.6, 139.7, 141.0, 153.0, HRMS (EI): calcd for C16H14O: 222.1045; found: 222.1045.
7-Methoxy-2-phenyl-2H-1-benzopyran (1g). A light yellow oil, Rf 0.51 (10:1 hexane/EtOAc), 1H NMR (CDCl3): δ 3.72 (s, 3H), 5.79-5.84 (m, 2H), 6.48 (dd, 1H, J = 10.7, 2.8 Hz), 6.57 (d, 1H, J = 2.8 Hz), 6.65 (dd, 1H, J = 8.7, 2.8 Hz), 6.72 (d, 1H, J = 8.7 Hz), 7.28-7.44 (m, 5H), 13C NMR (CDCl3): δ 55.6, 76.9, 111.7, 114.5, 116.5, 122.0, 124.1, 125.8, 127.0, 128.2, 128.6, 140.7, 147.0, 154.0, HRMS (EI): calcd for C16H14O2: 238.0994; found: 238.1002.
6-Chlro-7-methyl-2-phenyl-2H-1-benzopyran (1h). A light yellow oil, Rf 0.64 (10:1 hexane/EtOAc), 1H NMR (CDCl3): δ 2.27 (s, 3H), 5.80 (dd, 1H, J = 9.73, 3.46 Hz), 5.87 (dd, 1H, J = 3.5, 1.7 Hz), 6.46 (dd, 1H, J =9.7, 1.7 Hz), 6.66 (s, 1H), 6.98 (s, 1H), 7.71-7.44 (m, 5H), 13C NMR (CDCl3): δ 20.1, 77.1, 118.3, 120.4, 123.0, 125.0, 126.0, 126.4, 127.0, 128.5, 128.7, 137.0, 140.4, 151.4, HRMS (EI): calcd for C16H13OCl: 256.0655; found: 256.0655.
References
1. Y. Ashihara, Y. Nagata, and K. Kurosawa, Bull. Chem. Soc. Jpn., 1977, 50, 3298. CrossRef
2. J. L. Asherson, O. Bilgic, and D. W. Young, J. Chem. Soc., Perkin. Trans. 1, 1980, 522. CrossRef
3. (a) M. D. Agli, S. Bellosta, L. Rizzi, G. V. Galli, M. Canavesi, F. Rota, R. Parente, E. Bosisio, and S. Romeo, Cell. Mol. Life. Sci., 2005, 65, 2896; CrossRef (b) V. Arnaudinaud, B. Nay, S. Vergé, A. Nuhrich, G. Deffieux, J. M. Mérillon, J. P. Monti, and J. Vercauteren, Tetrahedron Lett., 2001, 42, 1279; CrossRef (c) V. Arnaudinaud, B. Nay, S. Vergé, A. Nuhrich, G. Deffieux, J. M. Mérillon, J. P. Monti, and J. Vercauteren, Tetrahedron Lett., 2001, 42, 5669. CrossRef
4. Y. Yamamoto and R. B. Gaynor, J. Clin. Invest., 2001, 107, 135. CrossRef
5. T. P. T. Cushnie and A. J. Lamb, International Journal of Antimicrobial Agents, 2005, 26, 343. CrossRef
6. R. R. de Sousa, K. C. Queiroz, A. C. Souza, S. A. Gurgueira, A. C. Augusto, and M. A. Miranda, M. P. Peppelenbosch , C. V. Ferreira and H. Aoyama, J Enzyme Inhib. Med. Chem., 2007, 22, 439. CrossRef
7. E. Grotewold, ‘The Science of Flavonoids, Springer, Science & Business Media, Inc., New York, 2006. CrossRef
8. (a) J. Tsuji, ‘Transition Metal Reagents and Catalysts: Innovations in Organic Synthesis.’ Wiley: Chichester, U.K., 2000; (b) S. Cacchi, P. G. Ciattini, E. Morera, and G. Ortar, Tetrahedron Lett., 1984, 25, 4821; CrossRef (c) R. E. Dolle, S. J. Schmidt, and L. I. Kruse, Tetrahedron Lett., 1988, 29, 1581. CrossRef
9. A reversible mechanism has been reported from a compound similar to 1 to a ring-opened isomer; (a) M. Sakuragi, Y. Kawanishi, Y. Suzuki, and H. Sakuragi, Bull. Chem. Soc. Jpn., 2008, 81, 641; CrossRef (b) A. Padwa, A. Au, G. A. Lee and W. Owens, J. Org. Chem., 1975, 40, 1142; CrossRef (c) Y. Kodama, T. Nakabayashi, K. Segawa, E. Hattori, M. Sakuragi, N. Nishi, and H. Sakuragi, J. Phys. Chem., A. 2000, 104, 11478. CrossRef
10. J.-R. Labrosse, P. Lhoste, and D. Sinou, Synth. Commun., 2002, 32, 3667 CrossRef