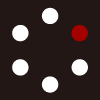
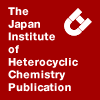
HETEROCYCLES
An International Journal for Reviews and Communications in Heterocyclic ChemistryWeb Edition ISSN: 1881-0942
Published online by The Japan Institute of Heterocyclic Chemistry
e-Journal
Full Text HTML
Received, 26th April, 2010, Accepted, 31st May, 2010, Published online, 31st May, 2010.
DOI: 10.3987/COM-10-11965
■ Synthesis, Structure and Electrochemical Behavior of a Novel Redox-Active Thiacalix[4]arene-Tetrathiafulvalene Assembly
Bang-Tun Zhao,* Wen-Bo Guo, and Pu-Zhou Hu
No. 71, Longmen Road, Department of Chemistry, Luoyang Normal University, Luoyang 471022, Henan Province, China
Abstract
A novel redox-active thiacalix[4]arene-tetrathiafulvalene assembly 5 was carried out through triethyl phosphite-mediated cross-coupling of the corresponding two 1,3-dithiole-2-(thi)ones (3 and 4). The structure of thiacalix[4]arene-tetrathiafulvalene assembly 5 was identified by X-ray diffraction analysis. Meanwhile, the preliminary electrochemical properties of 5 was investigated by cyclic voltammetry (CV) and two one-electron quasi-reversible waves with redox potentials are observed.INTRODUCTION
The study of redox-active molecular receptors capable of sensing charged or neutral substrates and reporting their presence by means of an electrochemical response is an area of intense current interest.1-5 Thanks to remarkable electronic properties, the tetrathiafulvalene moiety (TTF) has appeared as a key building-block for switchable process in various molecular and supramolecular architectures.6-11 More specifically, this system has demonstrated a good ability as a redox-probe in the topic of redox-switchable ligands.12-16 The binding unit of such redox-active receptors can involve various macrocyclic frameworks. By the virtue of their versatility and utility in supramolecular chemistry as host molecules, calixarenes have been considered as relevant structures, which mostly result from an easy access to the basic platform and a straightforward modification at the lower and upper rims.17-22 Thiacalixarenes have attracted considerable interest as an alternative to “classic” calixarenes by providing sites for functionalization not only on the aromatic rings, but also on the bridging sulfur atoms.23-26 Considering the redox properties of TTF and the scaffolding features of the calixarene moiety, several groups have paid special attention in joining the two families to produce calixarene-TTF assemblies and to develop new original electroactive architectures.27-35 We herein describe the synthesis, crystal structure and electrochemical properties of a novel thiacalix[4]arene-TTF electroactive architecture.
RESULTS AND DISCUSSION
Design and synthesis
The synthetic pathway was depicted in Scheme 1. The key thiacalix-thione intermediate 3 was prepared by cyclocondensation step between the bisfunctionalized dibromo-thiacalix[4]arene derivative 1 36 and the so-called “zincate” salt (bis(tetraethylammonium)bis(1,3-dithiole-2-thione-4,5-dithiol) zincate, TEA2[Zn(DMIT)2]) 2 37 in the prsence of Na2S. In this case, the reaction was carried out under high dilution conditions, and afforded the 1:1 cycloadduct in satisfactory 50% yield. No evidence of higher cyclocondensation products nor oligomers was observed. The target thiacalix[4]arene-tetrathiafulvalene assembly 5 10 was achieved in 20% yield by the direct self-coupling of thiacalix-thione 3 and 4,5-propylenedithio-1,3-dithiole-2-one 4 38-39 mediated by triethylphosphite. The 1H NMR spectra of compounds 1, 3 and 5 show two singlets for both the But and Ar-H protons, however, the distinction between the cone and 1,3-alternate conformers is not easy, because 1H NMR spectra of both conformers appear the same resonance pattern for the But and Ar-H protons. According to reported research,40 the tetraalkylation of thiacalix[4]arene with bulky alkyl groups at lower rims may immobilize a main 1,3-alternate conformer. As for compounds 1, 3 and 5, their two phenyl rings with methoxy groups freely rotate along the axis of Ar-S bond. Therefore, their conformation should be in equilibrium between cone and 1,3-alternate in solution.
X-Ray structure
In order to gain more information about conformational properties, single crystals of compound 5 have been obtained by the slow evaporation of chloroform-methanol solution at rt and studied by X-ray diffraction methods. As seen from Figure 1, there are two isomers which both adopt the 1,3-alternate conformation of thiacalix[4]arene framework. Two TTF units are nearly planar.
Electrochemical behavior
The electrochemical properties of thiacalix[4]arene-tetrathiafulvalene assembly 5 was carried out on a CHI650 electrochemistry workstation in a dichloromethane-acetonitrile (1/1, v/v) mixture. As expected from the electrochemical inertness of the thiacalix[4]arene platform,41 only the TTF redox signature is observed for compound 5, which undergoes the expected two quasi-reversible redox waves at Eox1 = 0.603 V and Eox2 = 0.896 (vs Ag/AgCl), corresponding to the successive formation of the stable TTF/TTF·+ and TTF·+/TTF2+ , respectively ( Figure 2).42
EXPERIMENTAL
All reactions were carried out under nitrogen atmosphere. All dry solvents were prepared according to standard procedures. Melting points are uncorrected and were determined using a Boetius Block apparatus (China). 1H(13C) NMR spectra were recorded at Bruker DPX 400 MHz. IR spectra were measured on an FT-IR spectrometer Nicolet Avata 350 in KBr. Mass spectra were measured using ESI technique on Bruker Esquire 3000 spectrometer. Elemental analyses were measured on Carlo-Erba 1106 instruments. X-Ray crystallographic data for 5 was obtained using a Bruker SMART APEX II CCD diffractometer equipped with graphite monochromated Mo Κα radiation (λ = 0.71073 Å) at 291 K. The structure was solved by direct methods using SHELXS-97 and refined using SHELXL-97 by full-matrix least-squares methods on F2.43-44 Hydrogen atoms were located from expected geometry and were isotropically refined by riding model. All non-hydrogen atoms were refined anisotropically except those of the disordered fragments. The φ-ω scan was used for absorption correction. Cyclic voltammetry (CV) experiments were performed on a CHI620C electrochemistry workstation in a three-electrode system served as Pt working electrode (2 mm, diameter), with Ag/AgCl and platinum wire acting as reference and counter electrodes, respectively.
Synthesis of thiacalix-thione intermediates 3
A mixture of dibromo-thiacalix[4]arene 1 (0.26 g, 0.26 mmol), TEA2[Zn(DMIT)2] 2 (0.37 g, 0.52 mmol), Na2S (0.061 g, 0.78 mmol) in dry THF(100 mL) was refluxed under N2 for 72 h. After the solution was cooled to room temperature, the solvent was evaporated under reduced pressure and the residue was resolved in CH2Cl2(30 mL).The crude mixture was washed with water (2×100 mL) and the organic layer was dried with Na2SO4.and evaporated to dryness under reduce pressure. Upon purification by a silicagel column chromatography (V(CH2Cl2) / V(petroleum ether) = 1 / 20), pure compound 3 was obtained as yellow poder in 50% yield. Mp>270 ºC. 1H NMR(400 MHz, CDCl3): δ: 7.51 (s, 4 H, ArH), 7.23 (s, 4 H, ArH), 3.88 (t, J= 5.87 Hz, 4 H, -OCH2CH2-), 3.22 (s, 6 H, -OCH3), 2.60 (t, J= 7.48 Hz, 4 H,-CH2CH2S-), 1.78~1.74 (m, 4 H, -CH2CH2CH2-), 1.29 (s, 18 H ,C(CH3)3 ), 1.27 (s, 18 H ,C(CH3)3 ). 13C NMR(125 MHz, CDCl3): δ: 212.1, 158.0, 156.2, 146.7, 146.0, 139.5, 131.3, 129.7, 127.3, 125.8, 70.0, 56.7, 34.4, 34.2, 31.3, 31.2, 30.7. IR(KBr, cm-1): υ: 2961, 2867, 1634, 1459, 1413, 1379, 1263, 1067, 1009, 877, 800, 761; MS-ESI: m/z calculated: 1027.6; found: 1049.5(M+Na-1)+. Anal. Calcd for C51H62O4S9, C, 59.64; H, 6.09; S, 28.04. Found: C, 59.72; H, 6.15; S, 28.08.
Synthesis of thiacalix[4]arene-tetrathiafulvalene assembly 5
A suspension of thiacalix[4]arene-thione 3 (0.10 mmol) and 4,5-propylenedithio-1,3-dithiole-2-one 4 (0.12 mmol) in freshly distilled triethylphosphite (2 mL) and dry toluene(2 mL ) was heated at 120°C for 6 h, under an N2 atmosphere. After cooling to rt, cold methanol (20 mL) was added and afforded a crude material which was purified by silicagel column chromatography ((V(CH2Cl2) / V(petroleum ether = 1 / 30). The pure compound 5 was obtained as orange powder in 20% yield. Mp>270 ºC. 1H NMR (400 MHz, CDCl3): δ: 7.49 (s, 4 H, ArH); 7.24 (s, 4 H, ArH); 3.81 (t, J= 6.28 Hz, 4 H, -OCH2CH2-), 3.14(s, 6 H, -OCH3), 2.71 (s, br, 4 H,-SCH2), 2.53 (s, 4 H,-SCH2), 2.40 (s, br, 2 H, -SCH2CH2CH2S-), 1.69 (s, br, 4 H, -OCH2CH2CH2S-), 1.27 (s, 18 H ,C(CH3)3 ), 1.24 (s, 18 H ,C(CH3)3 ). 13C NMR(125 MHz, CDCl3): δ: 157.9, 146.6, 145.9, 130.9, 129.4, 127.7, 126.7, 70.6, 34.3, 34.2, 33.0, 31.4, 31.2, 30.2, 29.7. IR(KBr, cm-1): υ: 2960, 2901, 2871, 1630, 1452, 1403, 1371, 1260, 1094, 1020, 801, 706. MS-ESI: m/z calculated: 1200.2; found: 1223.2(M+Na)+. Anal. Calcd for C57H68O4S12, C, 56.99; H, 5.71; S, 31.97. Found: C, 56.72; H, 5.83; S, 31.58.
Crystallographic study
X-Ray data for 5: C57H68O4S12, M = 1201.33 g/mol, Triclinic, space group P-1, a = 16.308(8) Ǻ, b = 17.039(8) Ǻ , c = 25.134(12) Ǻ, α = 107.395(6)º, β = 90.393(6)º , γ = 111.215(6)º, V = 6161(5) Ǻ; Z= 2, Dc =1.295 g/cm3, μ = 0.468 mm-1, F(000) = 2534, crystal dimensions of 0.23 x 0.19 x 0.18 mm. The final refinement gave R = 0.0959 and Rw = 0.2851 using 22725 independent reflections (θmax = 25.50, 1368 parameters). The max. and min. difference peaks and holes are 1.485 and -0.522 e·Å-3, respectively. Crystallographic data were deposited in CSD under CCDC registration number CCDC No. 769036.
ACKNOWLEDGEMENTS
We thank the Natural Science Foundation of China (Grant No. 20872058) for financial support.
References
1. C. Caltagirone and P. A. Gale, Chem. Soc. Rev., 2009, 38, 520. CrossRef
2. P. A. Gale, S. E. García-Garrido, and J. Garric, Chem. Soc. Rev., 2008, 37, 151. CrossRef
3. C. A. Nijhuis, B. J. Ravoo, J. Huskens, and D. N. Reinhoudt, Coord. Chem. Rev., 2007, 251, 1761. CrossRef
4. W. Wang and A. E. Kaifer, Angew. Chem. Int. Ed., 2006, 45, 7042. CrossRef
5. A. E. Kaifer and M. Gómez-Kaifer (Eds.), Supramolecular Electrochemistry, Wiely-VCH Weinheim, New York, 2004.
6. D. Canevet, M. Sallé, G. X. Zhang, D. Q. Zhang, and D. B. Zhu, Chem. Commun., 2009, 2245. CrossRef
7. J. L. Segura and N. Martin, Angew. Chem. Int. Ed., 2001, 40, 1372. CrossRef
8. M. R. Bryce, J. Mater. Chem., 2000, 10, 589. CrossRef
9. M. B. Nielsen, C. Lomholt, and J. Becher, Chem. Soc. Rev., 2000, 29, 153. CrossRef
10. P. Batail, Chem. Rev., 2004, 104, 4887. CrossRef
11. J. I. Yamada and T. Sugimoto (Eds.), TTF Chemistry: Fundamentals & Applications of Tetrathiafulvalene, Springer, Tokyo, Berlin, Heidelberg, New York, 2004.
12. Y. P. Zhao, X. J Wang, J. J. Wang, G. Si, Y. Liu, C. H. Tung, and L. Z. Wu, New J. Chem., 2009, 33, 813. CrossRef
13. H. Wu, D. Q. Zhang, G. X. Zhang, and D. B. Zhu, J. Org. Chem., 2008, 73, 4271. CrossRef
14. G. Trippé, F. Le Derf, P. Frère, and M. Sallé, Tetrahedron Lett., 2008, 49, 5452. CrossRef
15. C. Benhaoua, M. Mazari, N. Mercier, F. Le Derf, and M. Sallé, New J. Chem., 2008, 32, 913. CrossRef
16. J. Y. Balandier, A. Belyasmine, and M. Sallé, Eur. J. Org. Chem., 2008, 269. CrossRef
17. C. D. Gutsche, Monographs in Supramolecular Chemistry, Calixarenes, ed. by J. F. Stoddart, The Royal Society of Chemistry, Cambridge (1989).
18. J. Vicens and V. Böhmer (Eds.), Calixarene: A Versatile Class of Macrocyclic Compounds, Kluwer Academic Publishers, Dordrecht (1991).
19. A. Ikeda and S. Shinkai, Chem. Rev., 1997, 97, 1713. CrossRef
20. C. D. Gutsche, Calixarenes Revisited, The Royal Society of Chemistry, Cambridge (1998).
21. L. Mandolini and R.Ungaro, Calixarenes in Action, Imperial College Press, London (2000). CrossRef
22. Z. Asfari, V. Böhmer, J. M. Harrowfield, and J. Vicens, Calixarenes 2001, Kluwer Academic Publishers, Dordrecht (2001).
23. H. Kumagai, M. Hasegawa, S. Miyanari, Y. Sugawa, Y. Sato, T. Hori, S. Ueda, H. Kaniyama, and S. Miyano, Tetrahedron Lett., 1997, 38, 3971. CrossRef
24. N. Iki and S. Miyano, J. Inclusion Phenom. Macrocycl. Chem., 2001, 41, 99. CrossRef
25. P. Lhoták, Eur. J. Org. Chem., 2004, 1675. CrossRef
26. N. Morohashi, F. Narumi, N. Iki, T. Hattori, and S. Miyano, Chem. Rev., 2006, 106, 5291. CrossRef
27. S. Mendoza, L. A. Godínez, and A. E. Kaifer, Supramol. Chem., 2004, 16, 165. CrossRef
28. J. B. Regnouf de Vains, M. Sallé, and R. Lamartine, J. Chem. Soc., Perkin Trans. 2, 1997, 2461. CrossRef
29. B. T. Zhao, M. J. Blesa, N. Mercier, F. Le Derf, and M. Sallé, J. Org. Chem., 2005, 70, 6254. CrossRef
30. B. T. Zhao, M. J. Blesa, N. Mercier, F. Le Derf, and M. Sallé, Supramol. Chem., 2005, 17, 465. CrossRef
31. B. T. Zhao, M. J. Blesa, N. Mercier, F. Le Derf, and M. Sallé, New. J. Chem., 2005, 29, 1164. CrossRef
32. M. J. Blesa, B. T. Zhao, M. Allain, F. Le Derf, and M. Sallé, Chem. Eur. J., 2006, 12, 1906. CrossRef
33. J. Lyskawa, M. Sallé, J. Y. Balandier, F. Le Derf, E. Levillain, M. Allain, P. Viel, and S. Palacin, Chem. Commun., 2006, 2233. CrossRef
34. M. Frei, F. Diederich, R. Tremont, T. Rodriguez, and L. Echegoyen, Helv. Chim. Acta, 2006, 89, 2040. CrossRef
35. B. T. Zhao, M. J. Blesa, F. Le Derf, D. Canevet, C. Benhaoua, M. Mazari, M. Allain, and M. Salle, Tetrahedron, 2007, 63, 10768. CrossRef
36. B. T. Zhao, L. Wang, and B. X. Ye, Acta Chim. Sinica, 2007, 65, 1663.
37. C. Wang, A. S. Batsanov, M. R. Bryce, and J. A. K. Howard, Synthesis, 1998, 1615. CrossRef
38. G. Xue, Q. Fang, and W. T. Yu, Acta Cryst., 2003, C59, o653.
39. M. Kumasaki, H. Tanaka, and A. Kobayashi, J. Mater. Chem., 1998, 8, 301. CrossRef
40. P. Lhoták, M. Himl, I. Stibor, and H. Petříčková, Tetrahedron Lett., 2002, 43, 9621. CrossRef
41. N. Iki, S. Ogawa, T. Matsue, and S. Miyano, J. Electroanal. Chem., 2007, 610, 90. CrossRef
42. G. Y. Wen, D. Q. Zhang, Y. Y. Huang, R. Zhao, L. Y. Zhu, Z. G. Shuai, and D. B. Zhu, J. Org. Chem., 2007, 72, 6247. CrossRef
43. G. M. Sheldrick, SHELXS-97, Program for the Solution of Crystal Structures, University of Göttingen, Germany, 1997.
44. G. M. Sheldrick, SHELXL-97, Program for the Refinement of Crystal Structures, University of Göttingen, Germany, 1997.