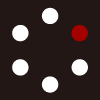
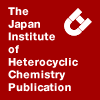
HETEROCYCLES
An International Journal for Reviews and Communications in Heterocyclic ChemistryWeb Edition ISSN: 1881-0942
Published online by The Japan Institute of Heterocyclic Chemistry
e-Journal
Full Text HTML
Received, 28th April, 2010, Accepted, 21st June, 2010, Published online, 21st June, 2010.
DOI: 10.3987/COM-10-11966
■ Copper-Mediated Oxidative Cyclization of Heterocyclically Substituted Aldimines
Daniela Pufky-Heinrich, Michael Ciesielski, Loubna Gharnati, Olaf Walter, and Manfred Döring*
Institute of Catalysis Research and Technology, Karlsruhe Institute of Technology, Hermann-von-Helmholtz Platz 1, 76344 Eggenstein-Leopoldshafen, Germany
Abstract
Copper-mediated cascade reactions were performed with heterocyclically substituted aldimines. These oxidative heterocyclizations include sequences of oxidations and cycloadditions or nucleophilic additions which take place in the coordination sphere of copper ions. When two heterocyclically substituted aldimines were reacted in the presence of copper (II) salts under air, pyridine derivatives were produced. In one case a 1,4-diazatricyclo-[3.2.1.02,7]oct-3-ene was also formed. The basic structure of this new tetracyclic compound contains five new chirale carbon atoms. This cage-like structure has been revealed by X-ray crystallography. The synthesis of 2H-pyrroles by copper-assisted conversions of the aldimines with dimethylacetylene carboxylate or quinones was also described.INTRODUCTION
Until recently, copper-mediated cyclization were rather seldom used for nitrogen-carbon bonding in the chemistry of heterocycles. Now, they are applied more frequently,1 as they allow for the formation of ring structures under mild conditions, in the presence of functional groups, and in a stereoselective manner. A milestone in the development of this type of reaction was the discovery of a highly efficient synthesis method of 1,2,3-triazoles by Sharpless2 and Meldal3 in 2002. This method based on a copper-catalyzed [3+2] cycloaddition has been applied widely.4 Meanwhile, similar ring closure reactions have been reported for the production of substituted pyrroles,5 imidazoles,6 and saturated five-membered heterocycles.7, 8 In addition, some copper-mediated aza-Diels-Alder reactions9, 10 have become known, which allow for the production of nitrogen-containing six-membered rings. The long known copper-catalyzed Ullmann reaction is increasingly applied for intramolecular conversion1 and plays an important role in the synthesis of nitrogen-containing ring structures. However, only a few cases of copper-mediated heterocyclization with ring formation by oxidative nitrogen-carbon bonding have been reported so far. One of the very few examples is the oxidative cyclization of semicarbazones11 and syntheses of nitrogen-bridged heterocycles developed by Junjappa et al.12 and our working group.13-18
The present report will deal with novel reactions which consist of various sequences of oxidation and cycloaddition steps or nucleophilic additions that entirely take place in the coordination sphere of the copper. These reactions show the performance of copper-mediated heterocyclizations and largely extend the application of this reaction principle.
For the reactions mentioned here (scheme 1), heterocyclically substituted aldimines (1) are applied as initial substances. Depending on their structure, their reaction partner, and the conditions chosen, they are converted either into pyridine derivatives (2), the new heterocycle (3), or into the 2H-pyrroles (4), (5), and (6).
RESULTS AND DISCUSSION
In the presence of 4 equivalents of copper(II) chloride per molecule imine and NEt3, the Schiff bases (1a-1e) react according to path A (scheme 1), with the terpyridines (2a), (2c-2e) or the triple substituted pyridine derivative (2b) being formed. First, the copper(I) complexes of the heterocycles are generated. The products are released by the addition of aqueous ammonia solution, with the copper ions being removed as water-soluble tetraamine complexes. The heterocycles (2a-2e) and the corresponding isolated yields are summarized in Table 1.
Surprisingly, the reaction of (1d) produced the compound (3) and the pyridine derivative (2d) (scheme 1, path B).
The compound (3) has a cage-like structure (Figure 1). To the best of our knowledge, this is the first report about it. This polycycle contains a distorted tetrahydropyridine unit that is bridged by a C-N bond via C7, C8, and C10 as well as an aciridine, pyrrolidine, and a tetrahydropyrazine ring each. Based on the bonding lengths, the C-N double bond was found at N3 and C6 (1.281 Å). The C-N single bonds have typical values of sp3-hybridized carbon atoms (1.46-1.51 Å).19 The basic structure of (3) contains five chiral carbon atoms (C7, C8, C9, C10 and C11). This corresponds to 16 different stereoisomers, but several of them cannot exist owing to the rigid fused-ring system. According to the NMR studies of the reaction solution, the isolated stereoisomer (3) is the main diastereomer (S,S,S,S,S and R,R,R,R,R). Only traces of another stereoisomer were detected.
Scheme 2 shows a reaction cascade that explains both the formation of the pyridine derivatives (2) and that of the compound (3). Accordingly, the imine is coordinated with copper (II) in the first step of the conversion reaction. Then, it oxidizes to 2-aza-1,3-diene by a two-electron oxidation. Two molecules of the latter react with each other in a [4+2] cycloadditon (aza-Diels-Alder reaction). This part of the reaction sequence has already been described: As reported by Wulff et al.,20 2-aza-1,3 diene is the 4π component reacting with the C-C double bond of a second molecule acting as dienophile.
In the further course of the reaction cascade, the tetrahydropyridine derivative (A) formed is oxidized to dihydropyridine (B) by two copper(II) ions. From the dihydropyridine (B), an imino fragment is eliminated and the pyridine (2) ring formed. However, the dihydropyridine generated by the conversion of (1d) may undergo another reaction instead. By a renewed [4+2] cycloaddition, compound (3) may be formed: This means that the heterocycles (2) and (3) are formed via the same intermediate. Hence, 3 equivalents of copper(II) chloride per molecule (1) are required. Because two molecules of (1) are converted during the reaction cascade a total amount of 6 equivalents copper(II) is needed to form one equivalent of (2) and (3), respectively. According to our findings, the yields are increased significantly, when a slight copper excess is used21. The excess reactant largely prevents the formation of imidazo[1,5-a]-pyridines which would otherwise be formed as by-products.
Copper ions may activate the Schiff bases (1) for further oxidative heterocyclizations. The aldimines (1a), (1b), and (1f) react with dimethyl-acetylenedicarboxylate, 1,4-benzoquinone or 1,4-naphthoquinone to substituted 2H-pyrroles (scheme 1, path C). A plausible mechanism of these heterocyclizations is represented in scheme 3. Accordingly, the aldimines (1) are first deprotonated with NEt3.
The resulting anions attack the carbon atoms of the electron-poor double or triple bonds. Another nucleophilic addition takes place, this time at the carbon atom of the imino group.
The thus formed intermediate cyclization products22 are oxidized to the products (4), (5), and (6) by copper(II) ions in two single-electron oxidation steps. This reaction is accompanied by a rearrangement. Contrary to the synthesis mentioned above, this type of reaction first forms the ring structure, while the oxidation process takes place subsequently. In the absence of oxygen, 2 equivalents of a copper(II) salt per mole of the Schiff base are required. In the presence of oxygen, this cyclization may also take place as a copper-catalyzed process, because the copper(I) ions formed are reoxidized. The isolated yields of (4), (5), and (6) are listed in Table 2.
Copper specificity of all reactions mentioned was demonstrated by experiments performed without metal salt or in the presence of redox-stable zinc(II) chloride with the remaining conditions being identical. No heterocycles were formed. However, the presence of copper ions is no sufficient requirement for the formation of cyclization products. The complexation behavior of the imine (1) also is of significance. Therefore, these reactions have only been observed if both R1 and R2 are heteroaryl substituents. Only those Schiff bases react, which may bind to copper as tridental chelate ligands. The imines (1a), (1b), and (1f) with their heterocyclic substituents R1 and R2 fulfill this requirement and are suitable substrates.
In summary, we have developed a novel heterocyclization route for the formation of nitrogen-containing heterocycles. Thus, the oxidation and cyclization steps may be repeat in a cascade-like manner and take place in the coordination sphere of copper ions. They are suited for the formation of nitrogen-containing, five-membered and six-membered rings as well as for the setup of cage-like structures. Furthermore, these substances might serve as potential ligands for metal complexes.
EXPERIMENTAL
NMR spectra were recorded with Bruker 250 MHz (250 MHz for 1H and 62 MHz for 13C). The ESI-MS data were obtained by an LD/MSD-1100-spectrometer from Hewlett Packard Company. IR spectra were recorded by a 2000-FT-IR-Spektrometer (KBr pressling).
Unless stated otherwise, all synthesis steps were performed under argon atmosphere.
The starting compounds were synthesized according literature known procedures:
1-pyridine-2-ylethaneamine (a),23 1-pyridine-1-ylpropaneamine (d),24 C-phenyl-C-pyridine-2-ylmethaneamine (f),25 The other amines were commercially available.
The crystal data for C26H26N8 (3) were recorded by a Nonius-Kappa-CCD 1000 diffractometer. M = 450.55, orthorhombic, Pna(2), a = 20.2315(15) Å, b = 10.0265(7) Å, c = 11.2033(8) Å, α = 90°, β = 90°, γ = 90°, V = 2272.6(3) Å3, Z = 4, Dc= 1.317 g cm-3, X-ray source Mo Kα (radiation), λ = 0.71073 Å, F (000) = 952, T = 183 K, R1 = 0.0673, wR2 = 0.1190. For searches relating to single-crystal X-ray diffraction data, the Cambridge Structural Database was used.26 Data were corrected for polarization and Lorentz effects, and an experimental absorption correction was performed with SADABS.27
Synthesis of 1-(1-Methyl-1H-imidazol-2-yl)ethaneamine (b)
Part I: A solution of NaOAc (15.67 g, 191 mmol) and NH4Cl (13.24 g, 191 mmol) in 80 mL H2O was heated up to 60 °C and 1-(1-methyl-1H-imidazole-2-yl)ethanone28 (15.77 g, 127 mmol) was added in a dropwise manner. The mixture was stirred for 2h at 60 °C, followed by neutralization with NaOH. The formed precipitate was filtered and recrystallized using EtOH. 1-(1-methyl-1H-imidazol-2-yl)ethanone oxime was a colorless solid: yield: 15.06 g (85%); mp 176 °C: ESI-MS m/z 139 (100, M+); 1H NMR (CDCl3): δ 11.32 (s, 1H), 7.18 (d, J = 0.8 Hz, 1H,), 6.92 (d, J = 0.8 Hz, 1H,), 3.78 (s, 3H), 2.19 (s, 3H); 13C NMR (CDCl3): δ 149.37, 143.04, 127.67, 125.11, 36.27, 12.58.
Part II: A mixture of the respective oxime (7.16 g, 5.15 mmol) and NaOAc (7.94 g, 103 mmol) in 40 mL water and 15 mL EtOH was heated up to 60 °C. Zinc dust (13.47 g, 206 mmol) was added in small portions to the solution within 30 min, heated afterwards to reflux for 1h, and filtered through a glass frit under hot conditions. The remaining solid was boiled three times in 30 mL EtOH/H2O (1:1). After another filtration, the combined filtrates were set to pH = 5 with conc. HCl, reduced to a small volume and cooled on ice. The amine was generated by the addition of KOH until the organic layer formed. Extraction with 40 mL THF (5x), drying of the combined organic layers over KOH and Na2SO4, and distillation in vacuo gave the final product as a colorless liquid: yield: 5.68 g (88%); Bp 58-68 °C (0.3 mbar); 1H NMR (CDCl3): δ 6.85 (d, J = 1.1 Hz, 1H,), 6.72 (d, J = 1.1 Hz, 1H), 4.05 (q, J = 6.7 Hz, 1H,), 3.59 (s, 3H), 3.59 (s, 3H), 1.69 (s, 1H), 1.42 (d, J = 6.7 Hz, 3H); 13C NMR (CDCl3): δ 152.15, 127.13, 121.26, 44.29, 32.87, 23.86.
General Procedure for the Synthesis of Aldimines (1)
A solution of the amines desired (10 mmol in 5 mL absolute EtOH) was cooled to 0 °C and the corresponding aldehydes (10 mmol in 15 mL absolute EtOH) were added. After 30 min of stirring, the ice bath was removed and the solution was continued to be stirred at room temperature. Volatile components of the mixture were removed under reduced pressure and the crude product was dried in vacuo. The imines (1a-f) were used without any further purification. Characterization was done exclusively with 1H NMR spectroscopy.
N-((1-Methyl-1H-imidazol-2-yl)methylene)-1-(pyridin-2-yl)ethane-amine (1a)
Colorless solid: yield: 2.10 g (98%); 1H NMR (CD3OD): δ 8.48 (m, 1H), 8.41 (s, 1H), 7.83 (m, 1H), 7.62 (m, 1H), 7.30 (m, 1H), 7.21 (d, J = 1.1 Hz, 1H), 7.21 (d, J = 1.1 Hz, 1H), 4.63 (q, J = 6.7 Hz, 1H), 4.06 (s, 3H), 1.59 (d, J = 6.7 Hz, 3H).
N-[1-(1-Methyl-1H-imidazol-2-yl)ethyl]-N-[(1-methyl-1H-imidazol-2-yl)methylen]amine (1b)
Colorless solid: yield: 2.13 g (98%); 1H NMR (CD3OD):δ 8.26 (s, 1H), 7.04 (d, J = 1.1 Hz, 1H), 6.91 (d, J = 1.2 Hz, 1H), 6.87 (d, J =1.1 Hz, 1H), 6.76 (d, J = 1.2 Hz, 1H), 4.72 (q, J = 6.7 Hz, 1H), 3.90 (s, 3H), 3.58 (s, 3H), 1.64 (d, J =6.7 Hz, 3H).
1-(Pyridin-2-yl)-N-((1-trityl-1H-imidazol-4-yl)methylene)ethane-amine (1c)
Colorless solid: yield: 4.38 g (99%); 1H NMR (CDCl3) δ 8.45 (m, 1H), 8.29 (s, 1H), 7.55 (m, 1H), 7.40 (m, 2H), 7.26 (m, 9 Hz), 7.05 (m, 8H), 4.52 (q, J = 6.7 Hz, 1H), 1.53 (d, J = 6.7 Hz, 3H).
N-((1-Methyl-1H-imidazol-2-yl)-methylene)-1-(pyridin-2-yl)propane-1-amine (1d)
Colorless solid: yield: 2.25 g (98%); 1H NMR (CD3OD); δ 8.47 (m, 1H), 8.34 (s, 1H), 7.58 (m, 1H), 7.38 (m, 1H), 7.09 (m, 1H), 7.04 (d, J =1.1 Hz, 1H) 6.87 (d, J = 1.1 Hz, 1H), 4.25 (t, J = 6.5 Hz,1H), 3.99 (s, 3H), 1.91 (m, 2H), 1.91 (t, J = 7.4 Hz, 3H).
1-(Pyridin-2-yl)-N-(quinolin-2-yl-methylene)ethane-amine (1e)
Yellow solid: yield: 2.59 g (99%);1H NMR (CDCl3): δ 8.70 (s, 1H), 8.57 (m, 1H), 8.19 (m, 3H), 7.82 (d, 1H), 7.71 (m, 2H), 7.55 (m, 2H), 7.16 (m, 1H), 4.84 (q, J =6.7 Hz, 1H), 1.68 (d, J = 6.7, 3H).
N-[(1-Methyl-1H-imidazol-2-yl)methylene]-N-[phenyl(pyridin-2-yl)methyl]amine (1f)
Colorless solid: yield: 2.73 g (99%); 1H NMR (CD3OD): δ 8.35 (s, 2H), 7.72 (m, 1H), 7.56 (m, 1H), 7.22 (m, 6H), 7.13 (d, J = 1.1 Hz, 1H), 6.98 (d, J =1.1 Hz, 1H), 5.61 (s, 1H), 4.02 (s, 3H).
General Procedure for the Synthesis of (2a), (2c), and (2e)
10.0 mmol of the imines 1a, 1c, and 1e were dissolved in 20 mL absolute EtOH. After adding a solution of 40.0 mmol CuCl2 in 20 mL absolute EtOH, the mixture was heated to reflux and 40.0 mmol of NEt3 were added. The reaction solution was stirred for 60 min at the same temperature. After cooling to room temperature, the organic layers were removed under reduced pressure and 30 mL of 10% NH4OH were added to the residue. The mixture was stirred for 30 min under air, followed by extraction with EtOAc (3x). The combined organic layers were dried over sodium sulfate and the solvent was removed under reduced pressure. The residues were purified by column chromatography (EtOAc and neutral Al2O3) to afford pyridine derivatives (2a), (2c), and (2e) in yields of 53%, 46%, and 40% as colorless crystals respectively.
6’-(1-Methyl-1H-imidazol-2-yl)-2,2’:4’,2’-terpyridine (2a)
mp 173 °C; 1H NMR (CDCl3): δ 9.08 (d, J = 1.7 Hz, 1H), 8.83 (d, J = 1.7 Hz, 1H), 8.75 (m, 2H), 8.40 (m, 1H), 8.03 (m, 1H), 7.82 (m, 2H), 7.33 (m, 2H), 7.19 (d, J = 1.0 Hz, 1H), 7.04 (d, J = 1.0 Hz, 1H), 4.29 ppm (s, 3H); 13C NMR (62 MHz, CDCl3): δ 156.14, 155.74, 154.72, 150.70, 150.03, 149.33, 148.47, 145.07, 136.79, 128.26, 124.49, 123.77, 121.16, 120.96, 120.27, 117.51, 36.78; ESI-MS m/z 313 (100%, M+). Anal. Calcd for C19H15N5: C, 72.83; H, 4.82; N, 22.35. Found: C, 72.44; H, 7.98; N, 22.46.
6’-(1-Trityl-1H-imidazol-4-yl)-2,2’:4’,2’’-terpyridine (2c)
mp 206 °C; 1H NMR (CDCl3): δ 8.88 (d, J = Hz, 1H), 8.69 (m, 1H), 8.62 (m, 1H), 8.58 (d, J = Hz, 1H), 8.40 (m, 1H), 7.99 (m, 1H), 7.66 (m, 3H), 7.57 (s, 1H), 7.31 (m, 10H), 7.17 (m, 7H); 13C NMR (CDCl3): δ 156.74, 156.48, 155.48, 150.25, 149.38, 148.65, 142.41, 139.52, 137.22, 137.12, 130.21, 128.63, 123.99, 121.81, 121.67, 120.83, 117.43; ESI-MS m/z 542 (100%, M+). Anal. Calcd for C37H27N5: C, 82.05; H, 5.02; N, 12.93. Found: C, 82.04; H, 5.22; N, 12.75.
6’-(Quinolin-2-yl)-2,2’:4’,2’’-terpyridine (2e)
mp 171 °C; 1H NMR (CDCl3): δ 9.34 (d, 1H, J = 1.6 Hz, 1H), 9.15 (d, J = 1.6 Hz, 1H), 8.83 (m, 2H), 8.73 (m, 2H), 8.29 (m, 2H), 8.11 (m, 2H), 7.87 (m, 3H), 7.75 (m, 1H), 7.56 (m, 1H), 7.36, (m, 1H); 13C NMR (CDCl3): δ 156.36, 156.11, 155.15, 150.02, 149.14, 148.63, 147.97, 136.86, 136.57, 129.96, 128.36, 127.52, 126.70, 123.81, 123.63, 121.37, 119.32, 119.16, 118.93; ESI-MS m/z 360 (100%, M+). Anal. Calcd for C24H16N4: C, 79.98; H, 4.47; N 15.54. Found: C, 78.82; H, 4.67; N, 15.14.
2,4,6-Tris(1-methyl-1H-imidazol-2-yl)pyridine (2b)
The imine (1b) (2.17 g, 10 mmol) was dissolved in 20 mL absolute EtOH and a solution of CuCl2 (5.38 g, 40.00 mmol) in 20 mL absolute EtOH was added. While heating to reflux, 4.05 g NEt3 (40 mmol) were added and the reaction mixture was stirred for another 15 min under reflux. Subsequently, the solution was cooled to ambient temperature, followed by a removal of the solvent under reduced pressure. 50 mL NH4OH (10%) were added and the resulting mixture was stirred at room temperature for 15 min in the presence of oxygen. The solution was extracted with EtOAc (3x), washed with a solution of ammonium acetate and the combined organic layers were dried over Na2SO4. After filtration the solvent was removed under reduced pressure. Compound (2b) was purified by column chromatography (Al2O3, acetone): yield: 32%; mp 198 °C; 1H NMR (CDCl3): δ 8.33 (s, 2H), 7.09 (m, 3H), 6.95 (m, 3H), 4.03 (s, 6H), 3.83 (s, 3H); 13C NMR (CDCl3): δ 149.88, 145.11, 139.72, 129.46, 128.70, 124.26, 123.85, 121.17, 35.85, 35.01; ESI-MS m/z 318 (100%, M+). Anal. Calcd for C17H17N7: C, 63.93; H, 5.37; N, 30.70. Found: C, 62.95; H, 5.37; N, 31.37.
Synthesis of (2d) and (3)
2.25 g (9.85 mmol) of compound (1d) were dissolved in 20 mL absolute EtOH and a solution of CuCl2 (5.30 g, 39.40 mmol) in 20 mL absolute EtOH was added. While heating to reflux, 3.99 g of NEt3 (39.40 mmol) were added. The reaction mixture was stirred for 30 min under reflux, cooled to ambient temperature and volatile components were removed under reduced pressure. Subsequently, 40 mL of 10% NH4OH were added and the resulting solution was stirred in air for 30 min, followed by extraction with CHCl3 (3x). The combined organic layers were washed with a solution of NH4OAc, dried over Na2SO4, filtered and the solvent was removed under reduced pressure. The crude product was purified by column chromatography (Al2O3, acetone) and recrystallized from EtOAc/n-hexane to give 2d (12%) and 3 (10%) as colorless crystals.
3’,5’-Dimethyl-6’-(1-methyl-1H-imidazol-2-yl)-2,2’:4’,2’’-terpyridin (2d)
mp 152 °C, 1H NMR (CDCl3): δ 8.69 (m, 1H), 8.60 (m, 1H), 7.72 (m, 3H), 7.25 (m, 1H), 7.05 (d, J = 1.1 Hz, 1H), 6.89 (d, J = 1.1 Hz, 1H), 3.73 (s, 6H), 2.14 (s, 3H), 2.08 (s. 3H); 13C NMR (CDCl3): δ 157.74, 156.57, 152.51, 149.77, 148.72, 147.02, 144.82, 135.52, 135.19, 130.29, 128.35, 126.23, 123.07, 122.54, 121.17, 121.10, 120.52, 33.25, 15.65; ESI-MS m/z 341 (100%, M+). Anal. Calcd for C21H19N5: C, 73.88; H, 5.61; N, 20.51. Found: C, 73.51; H, 5.86; N, 21.01.
2,6-Dimethyl-5,8-bis[1-methyl-1H-imidazole-2yl]-3,7-di-pyridine-2-yl-1,4-diazatricyclo[3.2.1.02,7]oct-3ene (3)
mp 216 °C; 1H NMR (CDCl3): δ 8.75 (m, 1H), 8.69 (m, 1H) , 8.58 (m, 1H), 7.79 (m, 3H), 7.37 (m, 1H), 7.21 (m, 1H), 7.07 (d, J = 1.0 Hz, 1H), 6.81 (d, J = 1.1 Hz, 1H), 6.73 (d, J =1.1 Hz, 1H), 6.69 (d, J =1.0 Hz, 1H), 4.12 (q, J = 0.7 Hz, 1H), 3.91 (s, 3H), 3.62 (s, 1H), 3.17 (s, 3H), 1.44 (s, 3H), 0.94 (d, J = 0.7 Hz, 3H); 13C NMR (CDCl3): δ 157.74, 156.57, 152.51, 148.77, 148.72, 147.02, 144.82, 135.52, 135.19, 130.29, 128.35, 126.23, 123.07, 122.54, 121.17, 121.10, 120.52, 33.25, 15.65; ESI-MS m/z 450 (100%, M+). Anal. Calcd for C26H26N8: C, 69.31; H, 5.82; N, 24.87. Found: C, 68.97; H, 5.79; N, 24.14.
Dimethyl 2-methyl-2,5-bis(1-methyl-1H-imida-zol-2-yl)-2H-pyrrole-3,4-dicarboxylate (4a)
To an ice-cooled solution of 1a (0.82 g, 3.77 mmol) in 10 mL MeOH, CuCl2 (0.051 g, 0.38 mmol in 5 mL MeOH), dimethyl acetylenedicarboxylate (0.54 g, 3.77 mmol), and NEt3 (0.038 g, 0.38 mmol) were added. While stirring vigorously, dry air was bubbled through the solution for 3h at room temperature. The mixture was concentrated under reduced pressure and the crude product was purified by column chromatography (Al2O3, EtOAc). The product was colorless crystals: yield: 0.26 g (19%) mp 167 °C; 1H NMR (CDCl3): δ 7.08 (d, J = 1.1 Hz, 1H), 6.96 (d, J = 1.1 Hz, 1H), 6.90 (d, J = 1.2 Hz, 1H), 6.72 (d, J = 1.2 Hz, 1H), 3.97 (s, 3H), 3.92 (s, 3H), 3.72 (s, 3H), 3.36 (s, 3H), 1.99 (s, 3H); 13C-NMR (CDCl3): δ 165.13, 161.56, 159.97, 155.91, 142.47, 140.32, 129.36, 130.39, 127.52, 125.99, 123.79, 83.32, 53.23, 52.94, 36.27,34.00, 23.68 ; IR (cm-1): 3118, 2949, 2843, 1743, 1714, 1576, 1434, 1331, 1280, 1235; ESI-MS m/z 357 (47%, M+). Anal. Calcd for C17H19N5O4: C, 57.12; H, 5.36; N, 19.60. Found: C, 56.76; H, 5.51; N, 19.58.
Dimethyl 2-methyl-5-(1-methyl-1H-imidazol-2-yl)-2-(pyridin-2-yl)-2H-pyrrole-3,4-dicarboxylate (4b)
CuCl2 (1.35 g, 10.00 mmol in 10 mL absolute MeOH) and dimethyl acetylenedicarboxylate (0.71 g, 5.00 mmol) were added to an ice-cooled solution of 1b (1.07 g, 5.00 mmol) in 15 mL absolute MeOH. The mixture was stirred for 10 min at 0 °C and NEt3 (0.51 g, 5.00 mmol) was added in drops while stirring for 30 min on ice, followed by 2h at room temperature. After concentrating the solution, 50 mL NH4OH (10%) were added in the presence of air and the solution was extracted five times with 30 mL CHCl3. The combined organic phases were washed with 20 mL of 5.0 M NH4OAc, dried over Na2SO4 and the solvent was removed in vacuo. The crude product was purified by column chromatography (silica gel, 1. EtOAc/n-hexane, 2. EtOAc/acetone) and the solvent was removed to afford (4b) as colorless crystals: yield: 0.30 g (17%); mp 171 °C; 1H NMR (CDCl3): δ 8.46 (m, 1H), 7.53 (m, 1H), 7.20 (m, 1H), 7.11 (m, 1H), 7.07 (d, J = 1.1 Hz, 1H), 6.92 (d, J = 1.1 Hz, 1H), 3.94 (s, 3H), 3.92 (s, 3H), 3.69 (s, 3H), 1.97 (s, 3H); 13C NMR (CDCl3): δ 165.42, 161.98, 157.67, 157.34, 149.41, 140.14, 138.77, 136.95, 130.04, 125.60, 123.09, 121.34, 87.68, 53.16, 52.64, 36.25, 22.30; IR (cm-1) 2949, 2843, 1736, 1713, 1579, 1435, 1426, 1328, 1275, 1253; ESI-MS m/z 355 (37%, (M+H)+). Anal. Calcd for C18H18N5O4: C, 61.01; H, 5.12; N, 15.81. Found: C, 61.08; H, 5.10; N, 15.52.
Dimethyl 5-(1-methyl-1H-imidazol-2-yl)-2-phenyl-2-(pyridin-2-yl)-2H-pyrrole-3,4-dicarboxylate (4f)
A solution of 1f (0.80 g, 2.90 mmol) in 10 mL absolute MeOH was cooled to – 15 °C and CuCl2 (0.78 g, 5.80 mmol in 10 mL absolute MeOH) was added in drops, followed by the addition of dimethyl-acetylenedicarboxylate (0.41 g, 2.90 mmol) and NEt3 (0.29 g, 2.90 mmol). The mixture was stirred for 30 min in an ice bath and for 2h at room temperature. Organic layers were removed under reduced pressure and 50 mL NH4OH (10%) were added. The solution was extracted with 30 mL EtOAc (6x), the combined organic layers were washed with 20 mL NH4OAc (5.0 M) and dried over Na2SO4. The solution was concentrated to a few milliliters and cooled to – 30 °C. The precipitate formed was recrystallized on EtOAc and purified by column chromatography (silica gel, EtOAc/n-hexane 2:1). (4f) colorless crystals: yield: 0.30 g (25%); mp 185 °C; 1H NMR (CDCl3): δ 8.42 (m, 1H), 7.60 (m, 1H), 7.45 (m, 1H), 7.20 (m, 3H), 7.09 (d, J = 0.9 Hz, 1H), 6.93 (d, J = 0.9 Hz, 1H), 3.97 (s, 3H), 3.91 (s, 3H), 3.67 (s, 3H); 13C NMR (CDCl3): δ 165.16, 162.44, 160.30, 159.46, 157.51, 149.10, 140.16, 139.55, 137.83, 136.55, 130.26, 128.43, 128.38, 128.27, 125.82, 124.94, 123.08, 93.58, 53.16, 52.57, 36.31; IR (cm-1): 2952 (s), 2842 (w), 1748, 1711, 1571 (s), 1428 (s), 1327 (s), 1276 (s), 1251 (s), 1158 (s); ESI-MS m/z 417 (22%, (M+H)+). Anal. Calcd for C23H20N4O4: C, 66.34; H, 4.84; N, 13.45. Found: C, 65.83; H, 4.65; N, 13.42.
1-Methyl-3-(1-methyl-1H-imidazol-2-yl)-1-(pyridin-2-yl)-1H-isoindole-4,7-diol (5a)
1a (0.80 g, 3.75 mmol in 10 mL absolute MeOH) and CuCl2 (2.02 g, 15.0 mmol in 10 mL absolute MeOH) were mixed at -15 °C. 1,4-benzoquinone (0.41 g, 3.75 mmol in 15 mL absolute MeOH) and NEt3 (1.52 g, 15.0 mmol) were added and the mixture was stirred for 30 min on ice, followed by 1h at room temperature. Volatile components were removed under reduced pressure and the remaining crude product was dissolved in 50 mL of 10% NH4OH. The solution was extracted with 30 mL CHCl3 (3x) and the extract washed (3x) with 5.0 M NH4OAc (25 mL). After removal of the organic layers under reduced pressure, the solid was purified (silica gel, EtOAc) and recrystallized (EtOAc/n-hexane) to afford (5a) as yellow crystals: yield: 0.11 g (9%); mp 188 °C; 1H NMR (CDCl3):δ 14.24 (s, 1H), 11.98 (s, 1H), 8.47 (m, 1H), 7.68 (m, 1H), 7.19 (m, 1H), 7.11 (d, J = 1.1 Hz, 1H), 7.03 (d, J = 1.1 Hz, 1H), 6.89 (m, 1H), 4.29 (s, 3H), 1.81 (s, 3H); 13C NMR (CDCl3): δ 164.01, 160.91,147.67, 157.16, 145.78, 141.32, 140.09, 138.59, 126.62, 125.94, 123.11, 122.66, 122.16, 121.30, 119.37, 82.23, 37.46, 26.63; IR (cm-1): 3456, 2964, 1640, 1442, 1576, 1442, 1262, 1093, 1031; ESI-MS m/z: 320 (18%, M+). Anal. Calcd for C18H16N4O2: C, 67.49, H, 5.03, N, 17.49. Found: C, 67.22; H, 5.16; N, 17.63.
1-Methyl-3-(1-methyl-1H-imidazol-2-yl)-1-(py-ridin-2-yl)-1H-benzo[f]isoindole-4,9-diol (6a)
1a (0.64 g, 3.00 mmol) was dissolved in 10 mL absolute MeOH and cooled to -10 °C. CuCl2 (1.61 g, 12 mmol in 15 mL absolute MeOH) and 1,4-naphthoquinone (0.48 g, 3.00 mmol in 20 mL absolute MeOH) were added to the suspension followed by a few min of cooling in an ice bath. NEt3 (1.21 g, 12.0 mmol) was added and the solution was stirred for 1h on ice and 1h at room temperature. The volume of the mixture was reduced and 50 mL NH4OH (10%) were added, while stirring in air. The crude product was filtered, washed with water, and purified by column chromatography (silica gel, EtOAc) to afford (6a) as yellow crystals: yield: 0.45 g (41%); mp 260 °C; 1H NMR (CDCl3): δ 15.40 (s, 1H), 12.63 (s, 1H), 8.54 (m, 1H), 8.39 (m, 1H), 8.25 (m, 1H), 7.69 (m, 2H), 7.48 (m, 2H), 7.20 (m, 1H), 7.17 (d, J =1.1 Hz, 1H), 7.05 (d, J = 1.1 Hz, 1H), 4.33 (s, 3H), 1.87 (s, 3H); 13C NMR (62 MHz): δ 165.03, 160.92, 147.68, 144.09, 141.56, 141.04, 138.54, 129.47, 129.01, 127.52, 126.33, 125.89, 125.32, 124.65, 123.00, 122.57, 121.02, 116.85, 81.46, 37.68, 27.35; IR (cm-1): 3442; 2970, 1583, 1476, 1368, 1359, 1029; ESI-MS m/z 370 (9%, M+). Anal. Calcd for C22H18N4O2: C, 71.34; H, 4.90; N, 15.13. Found: C, 71.22; H, 4.90; N, 15.11.
3-(1-Methyl-1H-imidazol-2-yl)-1-phenyl-1-(pyridin-2-yl)-1H-benzo[f]isoindole-4,9-diol (6f)
A solution composed of (1f) (0.69 g, 2.50 mmol in 8 mL MeOH), CuCl2 (0.034 g, 0.25 mmol in 2 mL MeOH), 1,4-naphthoquinone (0.40 g, 2.50 mmol in 35 mL MeOH), and NEt3 (0.025 g, 0.25 mmol) was stirred for 4h at room temperature, while bubbling dry air through the solution. The precipitate formed was filtered and recrystallized from EtOH to afford (6f) as yellow crystals: yield: 0.82 g (76%):. mp 279 °C; 1H NMR (CDCl3): δ 15.84 (s, 1H), 12.64 (s, 1H), 8.70 (m, 1H), 8.35 (m, 1H), 8.21 (m, 1H), 8.07 (m, 2H), 7.71 (d, J = 1.1 Hz, 1H), 7.55 (m, 3H), 7.43 (d, J = 1.1 Hz, 1H), 7.21 (m, 3H), 7.11 (m, 2H), 4.31 (s, 3H);13C NMR (CDCl3): 163.09, 159.56, 148.16, 143.54, 141.53, 141.42, 139.77, 128.73, 128.02, 127.91, 127.75, 127.60, 127.35, 127.18, 126.10, 125.86, 124.40, 124.09, 122.61, 122.15, 177.17, 86.88, 37.49; IR (cm-1): 437, 1635, 1438, 1350, 1022; ESI-MS m/z 432 (7%, M+). Anal. Calcd for C27H20N4O2: C, 74.99, H, 4.66, N, 12.95. Found: C, 74.29; H, 4.63; N, 12.87.
ACKNOWLEDGEMENTS
We are grateful to the Deutsche Forschungsgemeinschaft (DFG) for financial support.
References
1. S. R. Chemler and P. H. Fuller, Chem. Soc. Rev., 2007, 36, 1153. CrossRef
2. V. V. Rostovtsev, L. G. Green, V. V. Fokin, and K. B. Sharpless, Angew. Chem. Int. Ed., 2002, 41, 2596. CrossRef
3. C. W. Tornoe, C. Christensen, and M. Meldal, J. Org. Chem., 2002, 67, 3057. CrossRef
4. V. D. Bock, H. Hiemstra, and J. H. van Maarseveen, Eur. J. Org. Chem., 2006, 51. CrossRef
5. S. Kamijo, C. Kanazawa, and Y. Yamamoto, J. Am. Chem. Soc., 2005, 127, 9260. CrossRef
6. C. Kanazawa, S. Kamijo, and Y. Yamamoto, J. Am. Chem. Soc., 2006, 128, 10662. CrossRef
7. W. Z. Gao, X. M. Zhang, and M. Raghunath, Org. Lett., 2005, 7, 4241. CrossRef
8. C. Najera and J. M. Sansano, Angew. Chem. Int. Ed., 2005, 44, 6272. CrossRef
9. E. Jnoff and L. Ghosez, J. Am. Chem. Soc., 1999, 121, 2617. CrossRef
10. O. G. Mancheno, R. G. Arrayas, and J. C. Carretero, J. Am. Chem. Soc., 2004, 126, 456. CrossRef
11. R. Noto, M. Gruttadauria, P. Lomeo, V. Frenna, and G. Werber, J. Heterocycl. Chem., 1995, 32, 1277. CrossRef
12. H. O. Barun, H. Ila, H. Junjappa, and O. M. Singh, J. Org. Chem., 2000, 65, 1583. CrossRef
13. M. E. Bluhm, M. Ciesielski, H. Görls, and M. Döring, Angew. Chem. Int. Ed., 2002, 41, 2962. CrossRef
14. M. E. Bluhm, M. Ciesielski, O. Walter, and M. Döring, Inorg. Chem., 2003, 42, 8878. CrossRef
15. M. Ciesielski, D. Pufky, and M. Döring, Tetrahedron, 2005, 61, 5942. CrossRef
16. M. Ciesielski, O. Walter, and M. Döring, J. Inorg. Biochem., 2001, 86, 475.
17. M. Döring, M. Ciesielski, and H. Görls, J. Inorg. Biochem., 1999, 74, 117.
18. M. Döring, M. Ciesielski, O. Walter, and H. Görls, Eur. J. Inorg. Chem., 2002, 1615. CrossRef
19. J. March, Advanced Organic Chemistry. 4th Ed.; Wiley Interscience: New York, 1992.
20. G. Wulff and H. Böhnke, Angew. Chem., 1986, 98, 101; CrossRef Angew. Chem., Int. Ed. Engl., 1986, 25, 90. CrossRef
21. The dependencies of yield and selectivity on the reagent amount have been studied in detail on the example of the synthesis of pyridine (2a). If copper(II) was applied stoichiometrically (3 mol per mol aldimine) only 39% of (2a) was obtained but 8% of the corresponding imidazo[1,5-a]-pyridine was found as a by-product. If the amount of of Cu(II) was enhanced to 4 equivalents per mol aldimine the yield of (2a) increased to 53% and only 2% of the imidazo[1,5-a]-pyridine was obtained. If an even higher excess of Cu(II) was applied the yield of (2a) decreased again. However, the yield of (2a) was moderate at all reagent concentratioms owing to the formation of several other side products. As a characteristic by-product of this reaction the corresponding ketone (1-acetylpyridine) was isolated. Its formation can be explained by an oxidative scission of the aldimine.
22. A plausible alternative to the stepwise mechanism to give this intermediate is a concerted mechanism (1,3-dipolar cycloaddition).
23. H. Brunner and M. Niemetz, Monatsh. Chem., 2002, 133, 115. CrossRef
24. J. D. Bower and G. R. Ramage, J. Chem. Soc., 1955, 2834. CrossRef
25. S. O. Winthrop and G. Gavin, J. Org. Chem., 1959, 24, 1936. CrossRef
26. F. H. Allen and O. Kennard, Chem. Design Automation News, 1993, 8, 31.
27. Siemens area detector absorption program, Siemens, 1997.
28. D. H. Davies, J. Hall, and E. H. Smith, J. Chem. Soc., Perkin Trans. 1, 1991, 2691. CrossRef