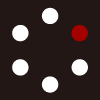
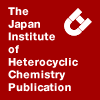
HETEROCYCLES
An International Journal for Reviews and Communications in Heterocyclic ChemistryWeb Edition ISSN: 1881-0942
Published online by The Japan Institute of Heterocyclic Chemistry
e-Journal
Full Text HTML
Received, 30th April, 2010, Accepted, 14th June, 2010, Published online, 14th June, 2010.
DOI: 10.3987/COM-10-11968
■ One-Pot Two-Step Synthesis of N3-Functionalized 3,4-Dihydropyrimidinones in the Presence of TMSCl
Zheng-Jun Quan,* Rong-Guo Ren, Yu-Xia Da, Zhang Zhang, Xiao-Dong Jia, Cai-Xia Yang, and Xi-Cun Wang*
Gansu Key Laboratory of Polymer Materials, College of Chemistry and Chemical Engineering, Northwest Normal University, Lanzhou, Gansu 730070, China
Abstract
Novel 3,4-dihydropyrimidinones modified with N3-alkyloxymethyl, aminomethyl, arylsulfonylmethyl, and azidomethyl groups can be regioselectively obtained over their isomeric N1 compounds in good yields by reaction of 3,4-dihydropyrimidinones with paraformaldehyde and alcohol, amine, sodium ben-zenesulfinate, and sodium azide, respectively, by a one-pot two-step strategy in the presence of chlorotrimethylsilane. The advantages of this method are the sim-ple procedure, the high regioselectivity of the products, no requirement for a base catalyst, and the mild reaction conditions.INTRODUCTION
In past decades, dihydropyrimidinones (DHPMs) and their derivatives have attracted considerable interest due to their heterocyclic scaffold1-8 and interesting pharmacological properties. They form the underlying structure for many clinically important substances, such as calcium channel modulators, antihypertensives, α1a adrenergic agonists, mitotic kinesin inhibitors, and hepatitis B virus replication suppressors.9-11 Among DHPM derivatives, most of the pharmacologically attractive forms are N3-substituted analogues.3 The N-alkylation of dihydropyrimidin-2-ones is one way of functionalizing the ring to achieve important bioactive properties. Most N-alkylated pyrimidin-2-ones are obtained from SN2 displacement of an electrophile, such as a diazoalkane,12 alkyl halide,13-15 alkyl sulfate, and alkyl phosphate,16 with the pyrimidine reacting as the nucleophile. Generally, most nucleophilic reactions17 involving N-alkylation of pyrimidinones yield N1-alkylated products.18
The classical method for the preparation of N3-substituted DHPMs, which employs a base-mediated alkylation of S-alkylated or O-alkylated pyrimidinones, results in protected N3-alkylated dihydropyrimidines.19 An alternative for this reaction is the “Atwal modification” of the Biginelli condensation.20, 21 Kappe et al. have reported the synthesis of N3-acylated DHPMs by acylation of DHPMs with ethyl chloroformate and N,N-dimethylcarbamoyl chloride22 or with anhydrides.23 Reaction of DHPMs with a base (LDA or n-BuLi) followed by quenching with electrophiles, resulted in a mixture of N1 and N3 position products.24, 25 N3-Acetoxymethyl26 DHPM has also been synthesized by standard acylation reaction of acetyl chloride with N3-hydroxylmethylated DHPM, which was produced by treating DHPM with aqueous formaldehyde and potassium carbonate at reflux temperature for 48 h.27 The N-alkyl-pyrimidin-2-ones were also generated by the cyclocondensation of 4-alkoxy-1,1,1-trihalo-3-alken-2-ones with methyl and allylureas and the N-alkylation of 4-(trihalomethyl)-pyrimidin-2-ones with methyl iodide and allyl bromide.28 DHPMs could be regioselectively substituted at the N3 position by aza-Michael addition reactions of DHPMs to α,β-ethylenic compounds.29,30 N3,S-Dimethylated 1,6-dihydropyrimidine has also been prepared by the reaction of 3,4-dihydropyrimidine-2-thiones with dimethyl carbonate.31
Unfortunately, these strategies suffer from the disadvantage that the procedures need to be carried out in harsh conditions such as high temperature with long reaction time and/or in the presence of strong base. Sometimes unexplained low selectivity in the position of N3 to N1 also occurs. To the best of our knowledge, a comparative study on the synthesis of DHPM derivatives, including addition of alkyloxymethyl, arylsulfonylmethyl, and azidomethyl groups at the N3 position of the pyrimidine ring by a one-pot multi-component reaction, has not yet been carried out. In this article, we present a convenient approach for the preparation of N3 functionalized DHPM derivatives that involves addition of alkyloxymethyl, aminomethyl, arylsulfonylmethyl, and azidomethyl groups under mild conditions and in the absence of a base catalyst. This was performed by treatment of DHPMs with paraformaldehyde and chlorotrimethylsilane (TMSCl), followed by reactions with various substrates such as alcohols, morpholine, sodium benzenesulfinate, and sodium azide.
RESUSTS AND DISCUSSIONS
Initially, experiments were carried out for the optimal reaction conditions using the reaction for 2a as a typical reaction. A mixture of DHPM (1a) with TMSCl and paraformaldehyde in dichloromethane was stirred for 24 h. The mixture subsequently was added to methanol for another 4 h to give N3-methoxymethyl DHPM (2a). The catalysts, solvents, and reaction temperature greatly influenced the product yield. Use of 2.0 equiv of TMSCl with 5 equiv of paraformaldehyde in CH2Cl2 at 35 oC afforded 2a in 73% yield; however, in the case of 2.5 equiv of TMSCl, the yield of 2a increased to 82%. Increasing the amount of TMSCl to 3 equiv led to isolation of 2a in a lower yield (60 %). Different solvents also influenced the reaction yield, as the reaction worked well in CH2Cl2 and THF; however, the product 2a was not detected when MeCN, TFA, p-TSA, HCl, acetic acid, FeCl3, and CuCl2 were used as catalysts. Thus, we decided to use 2.5 equiv of TMSCl and 5 equiv of paraformaldehyde in CH2Cl2 at 35 oC as the optimal reaction conditions for the preparation of N3 substituted DHPMs.
Under optimal conditions, other N3-methoxymethyl DHPMs (2b-c) were prepared in good yields (Table 1, entries 1-3). We then investigated other alcohols, such as ethanol, isopropanol, and tert-butyl alcohol as substrates and the corresponding N3-alkyloxymethyl DHPMs were obtained (Table 2, entries 4-8). In general, N3-substituted DHPMs were generated with primary, secondary and tertiary aliphatic alcohol substrates. In fact, when using tert-butyl alcohol as a nucleophile substrate, a lower yield of product 2 h resulted (entry 8).
To expand the scope and limitation of this one-pot reaction, we then investigated amine, sodium benzenesulfinate, and sodium azide as substrates in the reaction. The reaction between DHPM, paraformaldehyde, and morpholine in the presence of TMSCl was studied and good yields of DHPM derivatives 3a-e were obtained (Method A) (Table 2). Reactions for 3a-e were more efficient than alcohols and gave higher yields. We postulated that this reaction was performed by the three-component reaction of DHPMs, formaldehyde, and morpholine in the presence of TMSCl. As expected, the direct treatment of DHPMs 1 and formaldehyde with morpholine in methanol in the presence of TMSCl (Method B) or acetic acid (Method C), with refluxing for 8 h, resulted in the formation of compounds 3a-e with higher yields than those of the two-step reactions (Method A). However, N3-alkyloxymethyl DHPM (2) could not be obtained by method B or C. Therefore, we could presumably assume that the reaction for compounds 3a-e was performed via the Mannich reaction32 that started with the dehydration of the amine with formaldehyde to give an imine ion, which reacted with DHPMs to afford the products 3a-e.
Consistent with previous studies, replacing alcohol with sodium benzenesulfinate or 4-methylphenylsulfinic acid sodium salt allowed the reactions to proceed smoothly and afforded the desired products 4a-h in high yields (Table 3). However, this reaction needed a longer reaction time (second step with 12 h), which was probably due to the poor solubility of sodium benzenesulfinate in CH2Cl2. The electronic effects of the substituents on the aromatic rings of DHPMs 1 are not important. In the presence of a nitro group at the aromatic rings of DHPMs 1, the yields such as 4g and 4h were not apparently lower (Table 2, entries 7 and 8).
Finally, we observed that the readily available and stable DHPMs offered themselves for introduction of an azido group at the N3 position of pyrimidine ring. The reactions for the synthesis of compounds 5a-d were smoothly carried out by the procedures described above, using sodium azide as substrate instead of alcohol (Table 3, entries 9-12). However, the yields of 5a–d were moderate to good with longer reaction time (second step with 8 h), which perhaps due to the poor reactivity of sodium azide.
The structures of the N3-functionalized dihydropyrimidinones were determined by 1H NMR, H,H-COSY, and two-dimensional HMBC NMR experiments, using 2a as a model compound. The structures of compounds 2g and 5b were unambiguously confirmed by X-ray crystallography, revealing the N3 selectivity products N3-(isopropylmethyl)-3, 4-dihydropyrimidine-2-ones (2g), N3-(morpholinomethyl)-3, 4-dihydropyrimidine-2-ones (3c) and N3-(azidomethyl)-3, 4-dihydropyrimidine-2-ones (5b), which confirmed the structures of the obtained products.33
We postulate that the reaction starts with the reaction of paraformaldehyde with TMSCl to give 6, which then reacts with DHPM to afford the crucial intermediate N3-chloromethylation DHPM (9) by two possible pathways forming ammonium ion (7) or iminium ion (8). Subsequently, 9 reacts with the various nucleophiles (alcohols, benzenesulfinic acid sodium salt, and sodium azide) to give the N3-functionalization DHPMs 2,4,5 (Scheme 1). Although neither of the intermediates 6 or 9 was isolated, intermediate 9 was detected by LC-MS; the other intermediate was not detected.
In conclusion, a variety of N3-substituted DHPMs were regioselectively prepared through a one-pot two-step reaction between of 3,4-dihydropyrimidinones, paraformaldehyde, chlorotrimethylsilane, and various substrates. To the best of our knowledge, the methodology described herein affording the novel functionalized DHPM products has not been reported previously. Furthermore, the desired compounds contain N3CH2- group, which increases the diversity of the molecule and can allow preparation of other interesting libraries. Synthesis and screening of desired compounds based on DHPM scaffolds may lead to the discovery of interesting biological activities.
EXPERIMENTAL
All reagents were obtained commercially and used without further purification. Melting points were determined on an XT-4 electrothermal micromelting point apparatus and uncorrected. NMR spectra were recorded at 400 (1H) and 100 (13C) MHz, respectively, on a Varian Mercury plus-400 instrument using CDCl3 as solvent and TMS as internal standard. Elemental analyses were performed on a Carlo-Erba 1106 Elemental Analysis instrument. Mass-spectra were recorded on a TRACE DSQ instrument. Compounds 1 were prepared following the reported methods.34
General procedure for compounds 2a-h. To a suspension of 3,4-dihydropyrimidinone (1.0 mmol), paraformaldehyde (5.0 mmol), and CH2Cl2 (5 mL), TMSCl (2.5 mmol) were added and the reaction mixture was stirred at 35 oC for 24 h. Alcohol (3 mmol) was then added and the reaction mixture was stirred for an additional 4 h until the reaction was complete (monitored by TLC). After reaction completion, the solid was filtered and the filtrate was quenched with saturated aqueous NaHCO3 solution (10 mL). The aqueous phase was extracted with CH2Cl2 (15 mL) and the combined organic phase was washed with brine (15 mL), dried over Na2SO4, and concentrated. Purification by crystallization from aq. EtOH (EtOH: H2O = 4:1) afforded N3-substituted 3,4-dihydropyrimidinone (2a-h).
2a: Yield 82%; mp 157-159 oC. 1H NMR (400 MHz, CDCl3): δ = 9.05 (bs, 1H, NH), 7.38-7.26 (m, 5H, HAr), 5.33 (s, 1H, 4-CH), 5.19 (d, J = 10.4 Hz, 1H, NCHH), 4.22 (d, J = 10.4 Hz, 1H, NCHH), 4.12-4.07 (m, 2H, OCH2CH3), 3.32 (s, 3H, OCH3), 2.37 (s, 3H, 6-CH3), 1.22-1.18 (m, 3H, OCH2CH3). 13C NMR (100 MHz, CDCl3): δ = 165.5, 153.6, 146.3, 144.7, 128.5, 127.9, 127.4, 102.1, 75.6, 59.9, 57.9, 55.9, 18.3, 14.1. MS: m/z = 304 (M+). Anal. Calcd for C16H20N2O4 (304.14): C 63.14, H 6.62, N 9.20. Found: C 63.30, H 6.72, N 9.08.
2b: Yield 85%; mp 150-152 oC. 1H NMR (400 MHz, CDCl3): δ = 8.58 (bs, 1H, NH), 7.30-7.26 (m, 2H, HAr), 6.84-7.80 (m, 2H, HAr), 5.43 (s, 1H, 4-CH), 5.15 (d, J = 10.4 Hz, 1H, NCHH), 4.18 (d, J = 10.4 Hz, 1H, NCHH), 4.14-4.05 (m, 2H, OCH2CH3), 3.77 (s, 3H, OCH3), 3.32 (s, 3H, OCH3), 2.36 (s, 3H, 6-CH3), 1.26-1.18 (m, 3H, OCH2CH3). 13C NMR (100 MHz, CDCl3): δ = 165.5, 159.2, 153.4, 145.8, 133.9, 128.6, 113.8, 102.4, 75.4, 59.9, 57.3, 55.9, 55.2, 18.9, 14.1. MS: m/z = 334 (M+). Anal. Calcd for C17H22N2O5 (334.15): C 61.07, H 6.63, N 8.38. Found: C 61.25, H, 6.54, N 8.51.
2c: Yield 75%; mp 144-146 oC. 1H NMR (400 MHz, CDCl3): δ = 8.50 (bs, 1H, NH), 7.32-7.28 (m, 2H, HAr), 7.27-7.7.25 (m, 2H, HAr), 5.45 (s, 1H, 4-CH), 5.14 (d, J = 10.4 Hz, 1H, NCHH), 4.32 (d, J = 10.4 Hz, 1H, NCHH), 4.12-4.06 (m, 2H, OCH2CH3), 3.28 (s, 3H, OCH3), 2.36 (s, 3H, 6-CH3), 1.23-1.16 (m, 3H, OCH2CH3). 13C NMR (100 MHz, CDCl3): δ = 165.3, 153.1 146.1, 140.2, 133.8, 128.8, 128.7, 102.0, 75.7, 60.2, 57.4, 56.0, 18.5, 14.2, MS: m/z = 338 (M+). Anal. Calcd for C16H19ClN2O4 (338.10): C 56.72, H 5.65, N 8.27. Found: C 56.91, H, 5.74, N 8.13.
2d: Yield 88%; mp 172-174 oC. 1H NMR (400 MHz, CDCl3): δ = 8.12 (bs, 1H, NH), 7.35-7.27 (m, 5H, HAr), 5.93 (d, J = 10.0 Hz, 1H, NCHH), 5.68 (s, 1H, 4-CH), 4.66 (d, J = 10.0 Hz, 1H, NCHH), 4.19-4.12 (m, 2H, OCH2CH3), 3.68-3.64 (m, 1H, OCH2CH3), 3.56-3.52 (m, 1H, OCH2CH3), 2.36 (s, 3H, 6-CH3), 1.27-1.23 (m, 3H, OCH2CH3), 1.19-1.15 (m, 3H, OCH2CH3). 13C NMR (100 MHz, CDCl3): δ = 176.5, 165.2, 142.3, 140.5, 128.7, 128.3, 127.1, 103.7, 79.2, 64.6, 60.4, 57.2, 18.1, 14.9, 14.2. MS: m/z = 318 (M+). Anal. Calcd for C17H22N2O4 (318.16): C 64.13, H 6.97, N 8.80. Found: C 63.95, H 6.88, N 8.91.
2e: Yield 90%; mp 134-136 oC. 1H NMR (400 MHz, CDCl3): δ = 8.51 (bs, 1H, NH), 7.30-7.27 (m, 2H, HAr), 6.83-6.81 (m, 2H, HAr), 5.45 (s, 1H, 4-CH), 5.34 (d, J = 10.4 Hz, 1H, NCHH), 4.35 (d, J = 10.4 Hz, 1H, NCHH), 4.11-4.05 (m, 2H, OCH2CH3), 3.80 (s, 3H, OCH3), 3.61-3.57 (m, 1H, OCH2CH3), 3.52-3.42 (m, 1H, OCH2CH3), 2.37 (s, 3H, CH3), 1.24-1.16 (m, 6H, OCH2CH3×2). 13C NMR (100 MHz, CDCl3): δ = 165.5, 153.3, 145.9, 138.9, 137.7, 129.1, 127.3, 102.4, 73.9, 63.8, 60.0, 57.6, 21.1, 18.4, 14.9, 14.2. MS: m/z = 348 (M+). Anal. Calcd for C18H24N2O5 (348.17): C 62.05, H 6.94, N 8.04. Found: C 62.21, H 6.88, N 8.25.
2f: Yield 84%; mp 175-177 oC. 1H NMR (400 MHz, CDCl3): δ = 8.69 (bs, 1H, NH), 7.33-7.26 (m, 4H, HAr), 5.48 (s, 1H, C4-CH), 5.19 (d, J = 10.8 Hz, 1H, NCHH), 4.37 (d, J = 10.8 Hz, 1H, NCHH), 4.15-4.06 (m, 2H, OCH2CH3), 3.59-3.53 (m, 1H, OCH2CH3 ), 3.43-3.39 (m, 1H, OCH2CH3), 2.36 (s, 3H, 6-CH3), 1.26-1.20 (m, 3H, OCH2CH3), 1.18-1.12 (m, 3H, OCH2CH3). 13C NMR (100 MHz, CDCl3): δ = 165.3, 153.2, 146.3, 140.4, 133.7, 128.8, 128.6, 101.9, 74.2, 63.8, 60.1, 57.4, 18.4, 14.8, 14.2. MS: m/z = 352 (M+), 354 (M+2). Anal. Calcd for C17H21ClN2O4 (352.12): C 57.87, H 6.00, N 7.94. Found: C 58.01, H 6.08, N 7.81.
2g: Yield 71%; mp 127-129 oC. 1H NMR (400 MHz, CDCl3): δ = 8.56 (bs, 1H, NH), 7.39-7.32 (m, 3H, HAr), 7.31-7.32 (m, 2H, HAr), 5.53 (s, 1H, 4-CH), 5.26 (d, J = 10.4 Hz, 1H, NCHH), 4.34 (d, J = 10.4 Hz, 1H, NCHH), 4.13-4.07 (m, 2H, OCH2CH3), 3.74-3.68 (m, 1H, OCHCH3CH3 ), 2.35 (s, 3H, 6-CH3), 1.26-1.18 (m, 6H, CH(CH3)2), 1.07-1.05 (m, 3H, OCH2CH3). 13C NMR (100 MHz, CDCl3): δ = 165.5, 153.3, 146.1, 141.9, 128.5, 127.9, 127.4, 102.2, 71.9, 68.8, 59.9, 57.7, 22.7, 21.4, 18.4, 14.2. MS: m/z = 332 (M+). Anal. Calcd for C18H24N2O4 (332.17): C 65.04, H 7.28, N 8.43. Found: C 65.21, H 7.40, N 8.32.
2h: yield 65%; mp 142-144 oC. 1H NMR (400 MHz, CDCl3): δ = 8.76 (bs, 1H, NH), 7.38-7.24 (m, 5H, HAr), 5.60 (s, 1H, 4-CH), 5.40 (d, J = 10.0 Hz, 1H, NCHH), 4.12 (d, J = 10.0 Hz, 1H, NCHH), 4.10-4.06 (m, 2H, OCH2CH3), 2.32(s, 3H, CH3), 1.29-1.19 (m, 9H, CH3), 1.18-1.14 (m, 3H, OCH2CH3). 13C NMR (100 MHz, CDCl3): δ = 165.6, 152.7, 146.3, 141.8, 128.4, 127.9, 127.5, 102.0, 74.1, 67.9, 59.9, 57.0, 28.0, 18.4, 14.2. MS: m/z = 346 (M+), 348 (M+2). Anal. Calcd for C19H26N2O4 (346.19): C 65.87, H 7.56, N 8.09. Found: C 65.91, H 7.50, N 8.21.
General procedure compounds 3a-e. Method A: To a suspension of 3,4-dihydropyrimidinone (1.0 mmol), paraformaldehyde (5.0 mmol), and CH2Cl2 (5 mL), TMSCl (2.5 mmol) was added and the reaction mixture was stirred at 35 oC for 24 h. Morpholine (3 mmol) was then added and the reaction mixture was stirred for an additional 4 h until the reaction was complete (monitored by TLC). After reaction completion, the solid was filtered and the filtrate was quenched with saturated aqueous NaHCO3 solution (10 mL). The aqueous phase was then extracted with CH2Cl2 (15 mL) and the combined organic phase was washed with brine (15 mL), dried over Na2SO4, and concentrated. Purification by crystallization from aq. EtOH (EtOH: H2O = 6:1) afforded N3-morpholinomethyl 3,4-dihydropyrimidinone (3a-e).
Method B: A mixture of 3,4-dihydropyrimidinone (1.0 mmol), paraformaldehyde (5.0 mmol), TMSCl (0.5 mmol), and morpholine (3.0 mmol) in MeOH (5 mL) was refluxed under stirring for 8 h. The work-up was same as mentioned in Method A, giving compounds 3a-d.
Method C: A mixture of 3,4-dihydropyrimidinone (1.0 mmol), paraformaldehyde (5.0 mmol), AcOH (0.5 mmol), and morpholine (3.0 mmol) in MeOH (5 mL) was refluxed under stirring for 8 h. The work-up was same as mentioned in Method A, giving compounds 3a-d.
3a: Yield 90%; mp 176-178 oC. 1H NMR (400 MHz, CDCl3): δ = 8.73 (bs, 1H, NH), 7.35-7.24 (m, 5H, HAr), 5.59 (s, 1H, 4-CH), 4.24 (d, J = 12.0 Hz, 1H, NCHH), 4.17-4.08 (m, 2H, OCH2CH3), 3.72-3.66 (m, 4H, Hmorpholino), 3.42 (d, J = 12.0 Hz, 1H, NCHH), 2.62-2.57 (m, 2H, Hmorpholino), 2.49-2.44 (m, 2H, Hmorpholino), 2.35 (s, 3H, 6-CH3), 1.26-1.20 (m, 3H, OCH2CH3). 13C NMR (100 MHz, CDCl3): δ = 165.7, 153.9, 146.3, 141.7, 128.5, 127.8, 127.2, 101.9, 66.8, 65.7, 60.0, 58.2, 50.8, 18.4, 14.2. MS: m/z = 359 (M+). Anal. Calcd for C19H25N3O4 (359.18): C 63.49, H 7.01, N 11.69. Found: C 63.33, H 7.10, N 11.82.
3b: Yield 88%; mp 198-200 oC. 1H NMR (400 MHz, CDCl3): δ = 7.65 (bs, 1H, NH), 7.25 (d, J = 8.8 Hz, 2H, HAr), 6.82 (d, J = 8.8 Hz, 2H, HAr), 5.54 (s, 1H, 4-CH), 4.51 (d, J = 12 Hz, 1H, NCHH), 4.14-4.09 (m, 2H, OCH2CH3), 3.78 (s, 3H, CH3O), 3.74-3.69 (m, 4H, Hmorpholino), 3.39 (d, J = 12 Hz, 1H, NCHH), 2.61-2.56 (m, 2H, Hmorpholino), 2.49-2.44 (m, 2H, Hmorpholino), 2.33 (s, 3H, 6-CH3), 1.26-1.21 (m, 3H, OCH2CH3). 13C NMR (100 MHz, CDCl3): δ = 165.8, 159.2, 153.3, 145.5, 133.8, 128.4, 113.8, 102.5, 66.9, 65.6, 60.1, 57.6, 55.2, 50.9, 18.7, 14.2. MS: m/z = 389 (M+). Anal. Calcd for C20H27N3O5 (389.20): C 61.68, H 6.99, N 10.79. Found: C 61.85, H 7.10, N 10.92.
3c: Yield 85%, mp 202-204 oC. 1H NMR (400 MHz, CDCl3): δ = 8.36 (bs, 1H, NH), 7.25 (m, 2H, HAr), 7.10 (m, 2H, HAr), 5.55 (s, 1H, 4-CH), 4.52 (d, J = 12.0 Hz, 1H, NCHH), 4.14-4.11 (m, 2H, OCH2CH3), 3.70-3.68 (m, 4H, Hmorpholino), 3.40 (d, J = 12.0 Hz, 1H, NCHH), 2.57 (m, 2H, Hmorpholino), 2.48 (m, 2H, Hmorpholino), 2.35 (s, 3H, 6-CH3), 2.32 (s, 3H, CH3), 1.25-1.18 (m, 3H, OCH2CH3). 13C NMR (100 MHz, CDCl3) δ = 165.7, 153.9, 146.4, 140.9, 137.5, 129.2, 127.1, 101.6, 66.8, 65.6, 59.9, 57.9, 50.9, 21.1, 18.5, 14.2. MS: m/z = 373 (M+). Anal. Calcd for C20H27N3O4 (373.20): C 64.32, H7.29, N 11.25. Found: C 64.45, H 7.40, N 11.12.
3d: Yield 86%; mp 197-199 oC. 1H NMR (400 MHz, CDCl3): δ = 7.81 (bs, 1H, NH), 7.30-7.21 (m, 4H, HAr), 5.58 (s, 1H, 4-CH), 4.49 (d, J = 12 Hz, 1H, NCHH), 4.15-4.10 (m, 2H, OCH2CH3), 3.67-3.66 (m, 4H, Hmorpholino), 3.37 (d, J = 12 Hz, 1H, NCHH), 2.59-2.56 (m, 2H, Hmorpholino), 2.46-2.43 (m, 2H, Hmorpholino), 2.27 (s, 3H, 6-CH3), 1.25-1.18 (m, 3H, OCH2CH3). 13C NMR (100 MHz, CDCl3): δ = 165.5, 153.2, 146.0, 140.1., 133.7, 128.7, 128.6, 101.9, 66.9, 65.8, 60.2, 57.5, 50.8, 18.7, 14.2. MS: m/z = 393 (M+). Anal. Calcd for C19H24ClN3O4 (393.15): C 57.94, H 6.14, N 10.67. Found: C 57.61, H 6.20, N 10.43.
3e: Yield 92%; mp 212-214 oC 1H NMR (400 MHz, CDCl3): δ = 8.43 (bs, 1H, NH), 7.44-7.42 (m, 2H, HAr), 7.27-7.21 (m, 2H, HAr), 5.57 (s, 1H, 4-CH), 4.50 (d, J = 12.0 Hz, 1H, NCHH), 4.15-4.12 (m, 2H, OCH2CH3), 3.70-3.67 (m, 4H, Hmorpholino), 3.39 (d, J = 12 Hz, 1H, NCHH), 2.60-2.56 (m, 2H, Hmorpholino), 2.47-2.44 (m, 2H, Hmorpholino), 2.33(s, 3H, 6-CH3), 1.26-1.22 (m, 3H, OCH2CH3). 13C NMR (100 MHz, CDCl3): δ = 165.5, 153.6, 146.4, 140.7, 131.6, 129.0, 121.8, 101.6, 66.8, 65.7, 60.2, 57.6, 50.8, 18.5, 14.2. MS: m/z = 437 (M+). Anal. Calcd for C19H24BrN3O4 (437.10): C 52.06, H 5.52, N 9.59. Found: C 52.20, H 5.63, N 9.50.
General procedure compounds 4a-h: To a suspension of 3,4-dihydropyrimidinone (1, 1.0 mmol), paraformaldehyde (5.0 mmol, 5.0 equiv), and CH2Cl2 (5 mL), TMSCl (2.5 mmol, 2.5 equiv) were added and the reaction mixture was stirred at 35 oC for 24 h. Sodium benzenesulfinate (3 mmol) was then added and the reaction mixture was stirred for an additional 12 h. The work-up was same as mentioned in above processes, giving compounds 4a-h.
4a: Yield 76%; mp 193-194 oC. 1H NMR (400 MHz, CDCl3): δ = 7.96-7.94 (m, 2H, HAr), 7.68-7.55 (m, 3H, HAr), 7.35-7.27 (m, 5H, HAr), 5.67 (s, 1H, 4-CH), 5.30 (d, J = 14.4 Hz, 1H, NCHH), 4.14-4.05 (m, 2H, OCH2CH3), 3.95 (d, J = 14.4 Hz, 1H, NCHH), 2.27 (s, 3H, 6-CH3), 1.24-1.12 (m, 3H, OCH2CH3). 13C NMR (100 MHz, CDCl3): δ = 164.9, 151.8, 145.2, 139.9, 137.5, 134.2, 129.2, 128.9, 128.8, 128.7, 128.5, 127.6, 127.3, 102.2, 64.0, 60.5, 60.3, 18.3, 14.1. MS: m/z = 414 (M+). Anal. Calcd for C21H22N2O5S (414.12): C 60.85, H 5.35, N 6.76. Found: C 61.00, H 5.43, N 6.88.
4b: Yield 85%; mp 206-207 oC. 1H NMR (400 MHz, CDCl3): δ = 7.85-7.81 (m, 3H, HAr), 7.36-7.26 (m, 6H, HAr), 5.65 (s, 1H, 4-CH), 5.28 (d, J = 14.4 Hz, 1H, NCHH), 4.14-4.04 (m, 2H, OCH2CH3), 3.93 (d, J = 14.4 Hz, 1H, NCHH), 2.44 (s, 3H, CH3), 2.26 (s, 3H, 6-CH3), 1.26-1.18 (m, 3H, OCH2CH3). 13C NMR (100 MHz, CDCl3): δ = 164.9, 151.9, 145.4, 145.3, 140.0, 134.6, 129.9, 128.9, 128.8, 128.7, 127.6, 102.2, 64.0, 60.5, 60.2, 21.7, 18.3, 14.1. MS: m/z = 428 (M+). Anal. Calcd for C22H24N2O5S (428.14): C 61.67, H 5.65, N 6.54. Found: C 61.52, H 5.57, N 6.41.
4c: Yield 80%; mp 195-197 oC 1H NMR (400 MHz, CDCl3): δ = 8.08 (br, 1H, NH), 7.95-7.93 (m, 2H, HAr) 7.67-7.65 (m, 1H, HAr), 7.58-7.54 (m, 2H, HAr), 7.27-7.18 (m, 2H, HAr), 6.85-6.83 (m, 2H, HAr), 5.58 (s, 1H, 4-CH), 5.28 (d, J = 14.4 Hz, 1H, NCHH), 4.11-4.06 (m, 2H, OCH2CH3), 3.96 (d, J = 14.4 Hz, 1H, NCHH), 3.78 (s, 3H, OCH3), 2.22 (s, 3H, CH3), 1.22-1.18 (m, 3H, OCH2CH3). 13C NMR (100 MHz, CDCl3): δ = 164.9, 159.7, 152.3, 145.2, 137.6, 134.1, 132.1, 129.2, 128.8, 128.7, 114.0, 102.2, 63.9, 60.1, 59.9, 55.2, 18.1, 14.1. MS: m/z = 444 (M+). Anal. Calcd for C22H24N2O6S (444.14): C 59.45, H 5.44, N 6.30; Found: C 61.61, H 5.52, N 6.42.
4d: Yield 70%; mp 194-196 oC. 1H NMR (400 MHz, CDCl3): δ = 8.28 (br, 1H, NH), 7.82-7.80 (m, 2H, HAr) 7.36-7.34 (m, 2H, HAr), 7.20-7.17 (m, 2H, HAr), 6.84-6.82 (m, 2H, HAr), 5.56 (s, 1H, 4-CH), 5.27 (d, J = 14.4 Hz, 1H, NCHH), 4.13-4.03 (m, 2H, OCH2CH3), 3.99 (d, J = 14.4 Hz, 1H, NCHH) 3.78 (s, 3H, OCH3), 2.44 (s, 3H, CH3), 2.23 (s, 3H, CH3) 1.25-1.18 (m, 3H, OCH2CH3). 13C NMR (100 MHz, CDCl3): δ = 165.0, 159.7, 152.2, 145.3, 145.1, 134.7, 132.1, 129.9, 128.9, 128.7, 127.6, 114.1, 102.2, 67.0, 63.9, 60.0, 55.2, 21.7, 18.1, 14.1. MS: m/z = 458 (M+). Anal. Calcd for C23H26N2O6S (458.15): C 60.25, H 5.72, N 6.11. Found: C 60.37, H 5.81, N 6.02.
4e: Yield 79%; mp 197-199 oC. 1H NMR (400 MHz, CDCl3): δ = 7.95-7.93 (m, 2H, HAr), 7.92 (br, 1H, NH), 7.69-7.65 (m, 1H, HAr), 7.58-7.54 (m, 2H, HAr), 7.16-7.11 (m, 4H, HAr), 5.60 (s, 1H, 4-CH), 5.28 (d, J = 14.4 Hz, 1H, NCHH), 4.13-4.04 (m, 2H, OCH2CH3), 3.96 (d, J = 14.4 Hz, 1H, NCHH), 2.33 (s, 3H, CH3), 2.23 (s, 3H, 6-CH3), 1.22-1.19 (m, 3H, OCH2CH3). 13C NMR (100 MHz, CDCl3): δ = 164.9, 151.9, 145.1, 138.5, 137.6, 136.9, 134.2, 129.5, 129.2, 128.8, 127.5, 102.3, 64.0, 60.2, 21.1, 18.3, 14.1. MS: m/z = 428 (M+). Anal. Calcd for C22H24N2O5S (428.14): C 61.67, H 5.65, N 6.54. Found: C 61.80, H 5.58, N 6.43.
4f: Yield 86%; mp 196-198 oC. 1H NMR (400 MHz, CDCl3): δ = 7.82-7.80 (m, 1H, HAr), 7.36-7.26 (m, 6H, HAr), 7.17-7.10 (m, 1H, HAr), 5.60 (s, 1H, 4-CH), 5.29 (d, J = 14.4 Hz, 1H, NCHH), 4.13-4.04 (m, 2H, OCH2CH3), 3.93 (d, J = 14.4 Hz, 1H, NCHH), 2.44 (s, 3H, CH3), 2.32 (s, 3H, CH3), 2.26 (s, 3H, 6-CH3), 1.24-1.18 (m, 3H, OCH2CH3). 13C NMR (100 MHz, CDCl3): δ = 164.9, 151.7, 145.4, 145.1, 138.5, 137.0, 136.9, 134.6, 129.9, 129.5, 128.9, 127.6, 102.3, 64.0, 60.5, 21.8, 21.7, 18.3, 14.1. MS: m/z = 442 (M+). Anal. Calcd for C23H26N2O5S (442.16): C 62.42, H 5.92, N 6.33. Found: C 62.60, H 5.83, N 6.45.
4g: Yield 70%; mp 166-168 oC. 1H NMR (400 MHz, CDCl3): δ = 8.20 (d, J = 8.4 Hz, 2H, HAr), 7.94 (d, J = 8.4 Hz, 2H, HAr), 7.70 (br, 1H, NH), 7.60-7.57 (m, 3H, HAr), 7.26-7.24 (m, 2H, HAr), 5.87 (s, 1H, 4-CH), 5.34 (d, J = 14.4 Hz, 1H, NCHH), 4.17-4.11 (m, 2H, OCH2CH3), 3.85 (d, J = 14.4 Hz, 1H, NCHH), 2.29 (s, 3H, 6-CH3), 1.26-1.22 (m, 3H, OCH2CH3). 13C NMR (100 MHz, CDCl3): δ = 164.4, 151.5, 148.0, 147.0, 146.1, 137.2, 134.5, 129.4, 128.8, 128.5, 124.2, 101.5, 64.1, 60.7, 59.9. 18,6, 14.2. MS: m/z = 459 (M+). Anal. Calcd for C21H21N3O7S (459.11): C 54.89, H 4.61, N 9.15. Found: C 54.99, H 4.72, N 9.02.
4h: Yield 72%; mp 208-210 oC. 1H NMR (400 MHz, CDCl3): δ = 8.20 (d, J = 8.8 Hz, 2H, HAr), 7.80 (d, J = 8.4 Hz, 2H, HAr), 7.50-7.48 (m, 2H, HAr), 7.38-7.30 (m, 2H, HAr), 7.27 (br, 1H, NH), 5.88 (s, 1H, 4-CH), 5.32 (d, J = 14.4 Hz, 1H, NCHH), 4.15-4.11 (m, 2H, OCH2CH3), 3.83 (d, J = 14.4 Hz, 1H, NCHH), 2.46 (s, 3H, CH3), 2.31 (s, 3H, 6-CH3), 1.25-1.21 (m, 3H, OCH2CH3). 13C NMR (100 MHz, CDCl3): δ = 164.4 151.6 147.9 147.0 146.2 145.7 134.3 130.0 128.7 128.5 124.2 101.4 64.1 60.6 59.8 21.8 18.5 14.1 MS: m/z = 473 (M+). Anal. Calcd for C22H23N3O7S (473.13): C 55.80, H 4.90, N 8.87. Found: C 55.92, H 4.99, N 8.75.
General procedure compounds 5a-d: To a suspension of 3,4-dihydropyrimidinone (1.0 mmol), paraformaldehyde (5.0 mmol), and CH2Cl2 (5 mL), TMSCl (2.5 mmol) were added and the reaction mixture was stirred at 35 oC for 24 h. Sodium azide (3 mmol) was then added and the reaction mixture was stirred for an additional 8 h. The work-up was same as mentioned in above processes, giving compounds 5a-d.
5a: Yield 72%; mp 136-138 oC. 1H NMR (400 MHz, CDCl3): δ = 8.24 (br, 1H, NH), 7.35-7.26 (m, 5H, HAr), 5.54 (s, 1H, 4-CH), 5.17 (d, J = 12.0Hz, 1H, NCHH), 4.26 (d, J = 12.0Hz, 1H, NCHH), 4.11-4.08 (m, 2H, OCH2CH3), 2.37 (s, 3H, 6-CH3), 1.23-1.19 (m, 3H, OCH2CH3). 13C NMR (100 MHz, CDCl3): δ = 165.2, 152.9, 145.5, 141.2, 128.8, 128.4, 127.3, 102.4, 60.9, 60.3, 60.2, 18.5, 14.1. MS: m/z = 315 (M+). Anal. Calcd for C15H17N5O3 (315.13): C 57.13, H 5.43, N 22.21. Found: C 57.25, H 5.25, N 22.07.
5b: Yield 74%; mp 184-186 oC. 1H NMR (400 MHz, CDCl3): δ = 8.15 (br, 1H, NH), 7.28-7.25 (m, 2H, HAr), 6.86-6.83 (m, 2H, HAr), 5.39 (s, 1H, 4-CH), 5.16 (d, J = 12.4Hz, 1H, NCHH), 4.24 (d, J = 12.4Hz, 1H, NCHH), 4.22-4.06 (m, 2H, OCH2CH3), 3.78 (s, 3H, OCH3), 2.36 (s, 3H, 6-CH3), 1.26-1.120 (m, 3H, OCH2CH3). 13C NMR (100 MHz, CDCl3): δ = 165.2 159.5, 153.0, 145.2, 133.4, 128.6, 114.0, 102.5, 60.8, 60.2, 59.7, 55.2, 18.5, 14.2. MS: m/z = 345 (M+). Anal. Calcd for C16H19N5O4 (345.14): C 55.64, H 5.55, N 20.28. Found: C 55.50, H 5.41, N 20.39.
5c: Yield 70%; mp 176-178 oC. 1H NMR (400 MHz, CDCl3): δ = 8.51 (br, 1H, NH), 7.26-7.23 (m, 2H, HAr), 7.14-7.12 (m, 2H, HAr), 5.40 (s, 1H, 4-CH), 5.16 (d, J = 12.4Hz, 1H, NCHH), 4.17 (d, J = 12.4Hz, 1H, NCHH), 4.09-4.05 (m, 2H, OCH2CH3), 2.35 (s, 3H, CH3), 2.33 (s, 3H, 6-CH3), 1.26-1.120 (m, 3H, OCH2CH3). 13C NMR (100 MHz, CDCl3): δ = 165.3, 153.3, 145.6, 138.3, 138.2, 129.4, 127.2, 102.3, 60.9, 60.1, 60.0, 21.1, 18.4, 14.1. MS: m/z = 329 (M+). Anal. Calcd for C16H19N5O3 (329.15): C 58.35, H 5.81, N 21.26. Found: C 58.25, H 5.69, N 21.39.
5d: Yield 75%; mp 180-182 oC. 1H NMR (400 MHz, CDCl3): δ = 8.35 (br, 1H, NH), 7.30-7.26 (m, 4H, HAr), HAr), 5.42 (s, 1H, 4-CH), 5.10 (d, J = 12.8Hz, 1H, NCHH), 4.30 (d, J = 12.8Hz, 1H, NCHH),4.13-4.07 (m, 2H, OCH2CH3), 2.35 (s, 3H, 6-CH3), 1.25-1.120 (m, 3H, OCH2CH3). 13C NMR (100 MHz, CDCl3): δ = 165.0, 153.0, 145.9, 139.9, 134.2, 128.9, 128.7, 102.0, 61.1, 60.3, 59.8, 18.5, 14.2. MS: m/z = 349 (M+). Anal. Calcd for C15H16ClN5O3 (349.09): C 51.51, H 4.61, N 20.01. Found: C 51.42, H 4.53, N 20.17.
ACKNOWLEDGMENT
We are thankful for the financial support from the National Nature Science Foundation of China (No. 20902073), the Natural Science Foundation of Gansu Province (No. 096RJZA116), and Scientific and Technological Innovation Engineering program of Northwest Normal University (nwnu-kjcxgc-03-64).
References
1. P. Biginelli, Gazz. Chim. Ital., 1893, 23, 360.
2. C. O. Kappe, Tetrahedron, 1993, 49, 6937. CrossRef
3. C. O. Kappe, Acc. Chem. Res., 2000, 33, 879. CrossRef
4. C. O. Kappe and A. Stadler, Org. React., 2004, 63, 1.
5. D. Dallinger, A. Stadler, and C. O. Kappe, Pure Appl. Chem., 2004, 76, 1017. CrossRef
6. L. Z. Gong, X. H. Chen, and X. Y. Xu, Chem. Eur. J., 2007, 13, 8920. CrossRef
7. M. A. Kolosov, V. D. Orlov, D. A. Beloborodov, and V. V. Dotsenko, Mol. Diversity, 2009, 13, 5. CrossRef
8. Z.-J. Quan, Z. Zhang, Y.-X. Da, and X.-C. Wang, Chin. J. Org. Chem., 2009, 29, 876 (In Chinese).
9. K. Singh, D. Arora, E. Poremsky, J. Lowery, and R. S. Moreland, Eur. J. Med. Chem., 2009, 44, 1997. CrossRef
10. C. O. Kappe, Eur. J. Med. Chem., 2000, 35, 1043. CrossRef
11. K. Singh, D. Arora, K. Singh, and S. Singh, Mini Rev. Med. Chem., 2009, 9, 95. CrossRef
12. J. L. Wong and D. S. Fuchs, J. Org. Chem., 1971, 36, 848. CrossRef
13. K. Danel, E. Larsen, E. B. Pedersen, B. F. Vestergaard, and C. Nielsen, J. Med. Chem., 1996, 39, 2427. CrossRef
14. D. E. Jane, K. Hoo, R. Kamboj, M. Deverill, D. Bleakman, and A. Mandelzys, J. Med. Chem., 1997, 40, 3645. CrossRef
15. K. K. Ogilvie and S. L. Beaucage, Tetrahedron Lett., 1978, 19, 1663. CrossRef
16. K. Yamauchi and M. Kinoshita, J. Chem. Soc., Perkin Trans. 1, 1973, 391. CrossRef
17. A. Gambacorta, D. Tofani, M. A. Loreto, T. Gasperi, and R. Bernini, Tetrahedron, 2006, 62, 6848. CrossRef
18. K. Folkers and T. B. Johnson, J. Am. Chem. Soc., 1934, 56, 1374. CrossRef
19. C. O. Kappe and P. Roschger, J. Heterocycl. Chem., 1989, 26, 55. CrossRef
20. A. L. Marzinzik and E. R. Felder, J. Org. Chem., 1998, 63, 723. CrossRef
21. M. J. Lusch and J. A. Tallarico, Org. Lett., 2004, 6, 3237. CrossRef
22. C. O. Kappe, Bioorg. Med. Chem. Lett., 2000, 10, 49. CrossRef
23. D. Dallinger, N. Y. Gorobets, and C. O. Kappe, Org. Lett., 2003, 5, 1205. CrossRef
24. K. Singh, S. Singh, and A. Mahajan, J. Org. Chem., 2005, 70, 6114. CrossRef
25. K. Singh and S. Singh, Tetrahedron Lett., 2006, 47, 8143. CrossRef
26. P. Csomós, L. T. Kanerva, G. Bernáth, and F. Fülöp, Tetrahedron: Asymmetry, 1996, 7, 1789. CrossRef
27. B. Schnell, W. Krenn, K. Faber, and C. O. Kappe, J. Chem. Soc., Perkin Trans. 1., 2000, 4382. CrossRef
28. N. Zanatta, D. Faoro, L. da S. Fernandes, P. B. Brondani, D. C. Flores, A. F. C. Flores, H. G. Bona-corso, and M. A. P. Martins, Eur. J. Org. Chem., 2008, 5832. CrossRef
29. X.-C. Wang, Z.-J. Quan, and Z. Zhang, Tetrahedron, 2007, 63, 8227. CrossRef
30. X.-C. Wang, Z.-J. Quan, J.-K. Wang, Z. Zhang, and M. Wang, Bioorg. Med. Chem. Lett., 2006, 16, 4592. CrossRef
31. X.-C. Wang, Z.-J. Quan, and Z. Zhang, Chin. J. Chem., 2008, 26, 368. CrossRef
32. Compounds 3a and 3b have been synthesized via Mannich reaction: M. N. Purohit, K. Bhavya, and G. V. Pujar, J. Pharm. Chem., 2009, 3, 1.
33. CCDC No. 746488 for 2g, 752370 for 3c and 749553 for 5b contain the supplementary crystal-lographic data for this paper. These data can be obtained free of charge from The Cambridge Crystallographic Data Centre via www.ccdc.cam.ac.uk/data_request/cif.
34. N. Y. Fu, Y. F. Yuan, Z. Cao, S. W. Wang, J. T. Wang, and C. Peppe, Tetrahedron, 2002, 58, 4801. CrossRef