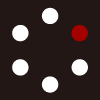
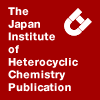
HETEROCYCLES
An International Journal for Reviews and Communications in Heterocyclic ChemistryWeb Edition ISSN: 1881-0942
Published online by The Japan Institute of Heterocyclic Chemistry
e-Journal
Full Text HTML
Received, 24th May, 2010, Accepted, 17th June, 2010, Published online, 18th June, 2010.
DOI: 10.3987/COM-10-S(E)37
■ A Concise Total Synthesis of (±)-Centrolobine
Haruhiko Fuwa,* Kenkichi Noto, and Makoto Sasaki
Tohoku University, 1-1, Tsutsumidori, Amamiya, Aoba-ku, Sendai, 981-8555, Japan
Abstract
A concise total synthesis of (±)-centrolobine has been achieved in four linear steps from commercially available p-benzyloxybenzaldehyde via our developed domino olefin cross-metathesis/intramolecular oxa-conjugate cyclization.INTRODUCTION
(−)-Centrolobine is a diarylheptanoid natural product isolated from the heartwood of Centrolobium robustum and from the stem of Brosimum potabile in the Amazon rain forest, while its enantiomer, (+)-centrolobine, was identified from the closely related species Centrolobium tomentosum1 (Figure 1). These natural products and related compounds display anti-inflammatory, anti-bacterial, and anti-leishmanial activities. The gross structure was elucidated by a racemic synthesis of an O-methyl derivative. The absolute configuration of (−)-centrolobine was unequivocally determined by its enantioselective synthesis by Colobert and co-workers.2 The 2,6-cis-disubstituted tetrahydropyran-containing structure of centrolobine has attracted the attention of many synthetic organic chemists; more than twenty total and formal syntheses of centrolobine have been reported to date.2,3 We describe herein a concise total synthesis of (±)-centrolobine ((±)-1), which represents the shortest synthesis reported so far.
RESULTS AND DISCUSSION
Our synthesis of (±)-centrolobine was based on our recently developed domino olefin cross-metathesis/intramolecular oxa-conjugate cyclization4-6 catalyzed by the Hoveyda—Grubbs second-generation catalyst7 (HG-II), as illustrated in Scheme 1. The domino reaction allows for the stereoselective synthesis of substituted tetrahydropyrans in a single reaction vessel from readily available acyclic precursors.
The synthesis started with addition of vinylmagnesium bromide to commercially available p-benzyloxybenzaldehyde to give allylic alcohol (±)-28 in 95% yield (Scheme 2). Oxidation of (±)-2 with 2-iodoxybenzoic acid (IBX) provided enone 3 in 87% yield. On the other hand, alcohol (±)-4,9 the coupling partner, was prepared by the reaction of anisaldehyde with 4-pentenylmagnesium bromide in 95% yield.
As illustrated in Scheme 3, segments 3 and (±)-4 (1.5 equiv)10 thus prepared were reacted in the presence of 10 mol % of HG-II in CH2Cl2 at 100 °C under microwave irradiation for 20 min. 1H NMR analysis (500 MHz) of the crude reaction mixture indicated that the diastereomer ratio at the C611 stereogenic center was ca. 13:1 in favor of the desired 2,6-cis-disubstituted tetrahydropyran (±)-5.3i After separation of the undesired 2,6-trans isomer by flash chromatography on silica gel, (±)-5 was isolated in 74% yield as a single stereoisomer. The stereochemistry of (±)-5 was established by an NOE experiment as shown. Deprotection of the benzyl group with concomitant reduction of the carbonyl group of (±)-5 was best achieved by hydrogenolysis using 10% Pd/C in THF at room temperature,12 leading to (±)-1 in 69% yield. The spectroscopic data of the synthetic material were in full accordance with those reported.2,3
CONCLUSION
We have accomplished a concise total synthesis of (±)-centrolobine via our developed domino olefin cross-metathesis/intramolecular oxa-conjugate cyclization, which proceeded in only four linear steps from commercially available p-benzyloxybenzaldehyde. The present total synthesis constitutes the shortest synthesis of 1 reported so far,2,3 thereby illustrating the efficiency and usefulness of our newly developed strategy for the synthesis of substituted tetrahydropyrans.
EXPERIMENTAL
General remarks. All reactions sensitive to moisture and/or air were carried out under an atmosphere of argon in anhydrous solvents using oven-dried glassware. Anhydrous tetrahydrofuran and dichloromethane were purchased from Wako Pure Chemicals Industries, Ltd. All other chemicals were purchased at highest commercial grade and used directly. Analytical thin-layer chromatography was performed using E. Merck silica gel 60 F254 plates (0.25-mm thickness). Flash column chromatography was carried out using Kanto Chemical silica gel 60N (40-100 mesh, spherical, neutral) or Fuji Silysia silica gel BW-300 (200-400 mesh). IR spectra were recorded on a JASCO FT/IR-4100 spectrometer. 1H and 13C NMR spectra were recorded on a Varian Unity INOVA-500 or INOVA-600 spectrometer, and chemical shift values are reported in ppm (δ) downfield from tetramethylsilane with reference to internal residual solvent [1H NMR, CHCl3 (7.24), C6HD5 (7.15); 13C NMR, CDCl3 (77.0), C6D6 (128.0)]. Coupling constants (J) are reported in Hertz (Hz). The following abbreviations were used to designate the multiplicities: s = singlet; d = doublet; m = multiplet; br = broad. ESI-TOF mass spectra were measured on a Bruker microTOFfocus spectrometer.
Allylic alcohol (±)-2.8 To a solution of p-benzyloxybenzaldehyde (2.10 g, 9.89 mmol) in THF (50 mL) cooled to 0 °C was added vinylmagnesium bromide (1.0 M solution in THF, 11.9 mL, 11.9 mmol), and the resultant solution was stirred at 0 °C for 1 h. The reaction was quenched with saturated aqueous NH4Cl solution, and the resultant mixture was extracted with EtOAc. The organic layer was washed with brine, dried (Na2SO4), filtered, and concentrated under reduced pressure. Purification of the residue by flash chromatography on silica gel (10 to 20% EtOAc/hexanes) gave allylic alcohol (±)-2 (2.25 g, 95%) as colorless crystals. Mp 60.7—61.9 °C (lit.,8 60.3 °C); 1H NMR (500 MHz, CDCl3) δ 7.42 (d, J = 7.5 Hz, 2H), 7.37 (dd, J = 7.5, 7.5 Hz, 2H), 7.31 (dd, J = 7.5, 7.5 Hz, 1H), 7.28 (d, J = 8.5 Hz, 2H), 6.95 (d, J = 8.5 Hz, 2H), 6.03 (ddd, J = 17.0, 10.5, 6.0 Hz, 1H), 5.33 (d, J = 17.0 Hz, 1H), 5.17 (d, J = 10.5 Hz, 1H), 5.15 (m, 1H), 5.05 (s, 2H), 1.91 (br s, 1H).
Enone 3. To a solution of allylic alcohol (±)-2 (1.00 g, 4.17 mmol) in DMSO (40 mL) was added IBX (1.79 g, 6.26 mmol), and the resultant solution was stirred at room temperature for 3 h. The reaction was quenched with H2O, and the resultant mixture was extracted with EtOAc. The organic layer was washed with saturated aqueous Na2SO3 solution, saturated aqueous NaHCO3 solution, and brine, dried (Na2SO4), filtered, and concentrated under reduced pressure. Purification of the residue by recrystallization (EtOAc/hexanes) gave enone 3 (0.86 g, 87%) as colorless crystals. Mp 88.5—90.0 °C; IR (film) 1670, 1610, 1595, 1403, 1238, 1173, 786 cm-1; 1H NMR (500 MHz, CDCl3) δ 7.95 (d, J = 9.0 Hz, 2H), 7.43— 7.32 (m, 5H), 7.15 (dd, J = 17.0, 10.5 Hz, 1H), 7.02 (d, J = 9.0 Hz, 2H), 6.41 (dd, J = 17.0, 2.0 Hz, 1H), 5.86 (dd, J = 10.5, 2.0 Hz, 1H), 5.13 (s, 2H); 13C NMR (125 MHz, CDCl3) δ 189.2, 162.7, 136.1, 132.1, 131.0 (2C), 130.4, 129.3, 128.7 (2C), 128.2, 127.5 (2C), 114.7 (2C), 70.1; HRMS (ESI) calcd for C16H14O2Na [(M+Na)+] 261.0886, found 261.0881.
Hydroxyalkene (±)-4.9 To a solution of anisaldehyde (3.65 mL, 30.0 mmol) in THF (120 mL) cooled to 0 °C was added 4-pentenylmagnesium bromide (1.0 M solution in Et2O, 35.5 mL, 35.5 mmol), and the resultant solution was stirred at 0 °C for 1.5 h. The reaction was quenched with saturated aqueous NH4Cl solution, and the resultant mixture was extracted with EtOAc. The organic layer was washed with brine, dried (MgSO4), filtered, and concentrated under reduced pressure. Purification of the residue by flash chromatography on silica gel (10 to 15% EtOAc/hexanes) gave hydroxyalkene (±)-4 (5.87 g, 95%) as a colorless clear oil. 1H NMR (600 MHz, C6D6) δ 7.14 (d, J = 9.0 Hz, 2H), 6.79 (d, J = 9.0 Hz, 2H), 5.71 (m, 1H), 4.98 (dd, J = 16.8, 1.2 Hz, 1H), 4.94 (dd, J = 9.6, 1.2 Hz, 1H), 4.36 (m, 1H), 3.32 (s, 3H), 1.96—1.92 (m, 2H), 1.71 (m, 1H), 1.58 (m, 1H), 1.47 (m, 1H), 1.39—1.23 (m, 2H); 13C NMR (150 MHz, C6D6) δ 159.4, 138.9, 137.9, 127.3 (2C), 114.7, 114.0 (2C), 73.9, 54.7, 39.1, 34.0, 25.5.
Tetrahydropyran (±)-5.3i To a solution of hydroxyalkene (±)-4 (197.2 mg, 0.956 mmol) in CH2Cl2 (6 ml) placed in a Biotage microwave vial were added enone 3 (148.3 mg, 0.622 mmol) and HG-II (39.0 mg, 0.062 mmol). The vial was flushed with argon and then sealed. The reaction mixture was heated at 100 °C under microwave irradiation for 20 min. After cooling to room temperature, the reaction mixture was directly loaded onto a silica gel column. Purification of the residue by flash chromatography on silica gel (0 to 10% Et2O/benzene then 4% acetone/hexanes) gave tetrahydropyran (±)-5 (191.6 mg, 74%) as colorless crystals. Mp 132.0—133.2 °C (lit.,3i 132—135 °C); IR (film) 2933, 1674, 1600, 1512, 1248, 1171, 1036, 834 cm-1; 1H NMR (500 MHz, CDCl3) δ 7.95 (d, J = 9.0 Hz, 2H), 7.42—7.31 (m, 5H), 7.22 (d, J = 9.0 Hz, 2H), 6.98 (d, J = 9.0 Hz, 2H), 6.82 (d, J = 9.0 Hz, 2H), 5.11 (s, 2H), 4.36 (apparent d, J = 11.5 Hz, 1H), 4.12 (dddd, J = 11.0, 6.0, 6.0, 5.0 Hz, 1H), 3.76 (s, 3H), 3.33 (dd, J = 16.0, 6.0 Hz, 1H), 2.98 (dd, J = 16.0, 6.0 Hz, 1H), 1.92 (m, 1H), 1.84—1.77 (m, 2H), 1.71 (ddddd, J = 13.0, 12.5, 4.0, 4.0, 3.5 Hz, 1H), 1.51 (dddd, J = 12.5, 11.5, 4.0, 3.5 Hz, 1H), 1.34 (dddd, J = 13.0, 11.0, 4.0, 3.5 Hz, 1H); 13C NMR (CDCl3) δ 197.1, 162.6, 158.7, 136.2, 135.4, 130.7, 130.6 (2C), 128.7 (2C), 128.2, 127.5 (2C), 127.1 (2C), 114.4 (2C), 113.5 (2C), 79.5, 75.1, 70.1, 55.2, 45.3, 33.0, 31.4, 23.8; HRMS (ESI) calcd for C27H28O4Na [(M+Na)+] 439.1880, found 439.1883.
(±)-Centrolobine (±)-1.3 To a solution of tetrahydropyran (±)-5 (25.1 mg, 60.5 µmol) in THF (1.5 mL) was added 10% Pd/C (8.1 mg), and the resultant suspension was stirred at room temperature under an atmosphere of hydrogen (balloon) for 3 days. The resultant mixture was filtered through a pad of Celite, and the filtrate was concentrated under reduced pressure. Purification of the residue by flash chromatography on silica gel (6 to 20% acetone/hexanes) gave (±)-centrolobine (13.1 mg, 69%) as a colorless clear oil. IR (film) 3371, 2933, 1613, 1514, 1455, 1247, 1035, 829 cm-1; 1H NMR (500 MHz, CDCl3) δ 7.30 (d, J = 8.5 Hz, 2H), 7.03 (d, J = 8.5 Hz, 2H), 6.86 (d, J = 8.5 Hz, 2H), 6.71 (d, J = 8.5 Hz, 2H), 4.69 (br s, 1H), 4.28 (dd, J = 11.0, 1.5 Hz, 1H), 3.78 (s, 3H), 3.42 (m, 1H), 2.77—2.59 (m, 2H), 1.94—1.78 (m, 3H), 1.74—1.56 (m, 3H), 1.49 (dddd, J = 13.5, 12.5, 4.0, 3.5 Hz, 1H), 1.31 (dddd, J = 13.5, 11.0, 4.5, 4.0 Hz, 1H); 13C NMR (125 MHz, CDCl3) δ 158.7, 153.4, 135.8, 134.7, 129.5 (2C), 127.1 (2C), 115.0 (2C), 113.6 (2C), 79.1, 77.1, 55.3, 38.3, 33.3, 31.2, 30.7, 24.0; HRMS (ESI) calcd for C20H24O3Na [(M+Na)+] 335.1618, found 335.1612.
ACKNOWLEDGEMENTS
This work was supported in part by a Grant-in-Aid for Scientific Research on Innovative Areas “Organic Synthesis Based on Reaction Integration” (No. 22106504) from the Ministry of Education, Culture, Sports, Science and Technology (MEXT), Japan.
References
1. (a) I. L. De Albuquerque, C. Galeffi, C. G. Casinovi, and G. B. Marini-Bettolo, Gazz. Chim. Ital., 1964, 94, 287; (b) C. Galeffi, C. G. Casinovi, and G. B. Marini-Bettolo, Gazz. Chim. Ital., 1965, 95, 95; (c) L. V. Alegrio, R. Braz-Filho, and O. R. Gottlieb, Phytochemistry, 1989, 28, 2359; CrossRef (d) A. F. de C. Alcântara, M. R. Souza, and D. Piló-Veloso, Fitoterapia, 2000, 71, 613.
2. (a) F. Colobert, R. D. Mazery, G. Solladié, and M. C. Carreno, Org. Lett., 2002, 4, 1723; CrossRef (b) M. C. Carreno, R. D. Mazery, A. Urbano, F. Colobert, and G. Solladié, J. Org. Chem., 2003, 68, 7779. CrossRef
3. (a) S. Marumoto, J. J. Jaber, J. P. Vitale, and S. D. Rychnovsky, Org. Lett., 2002, 4, 3919; CrossRef (b) P. A. Evans, J. Cui, and S. J. Gharpure, Org. Lett., 2003, 5, 3883; CrossRef (c) E. Lee, H. J. Kim, and W. S. Jang, Bull. Korean Chem. Soc., 2004, 25, 1609; CrossRef (d) L. Boulard, S. BouzBouz, J. Cossy, X. Franck, and B. Figadere, Tetrahedron Lett., 2004, 45, 6603; CrossRef (e) P. A. Clarke and W. H. C. Martin, Tetrahedron Lett., 2004, 45, 9061; CrossRef (f) K. P. Chan and T.-P. Loh, Org. Lett., 2005, 7, 4491; CrossRef (g) P. A. Clarke and W. H. C. Martin, Tetrahedron, 2005, 61, 5433; CrossRef (h) M. P. Jennings and R. T. Clemens, Tetrahedron Lett., 2005, 46, 2021; CrossRef (i) S. Chandrasekhar, S. J. Prakash and T. Shyamsunder, Tetrahedron Lett., 2005, 46, 6651; CrossRef (j) G. Sabitha, K. B. Reddy, G. S. K. K. Reddy, N. Fatima, and J. S. Yadav, Synlett, 2005, 2347; CrossRef (k) V. Böhrsch and S. Blechert, Chem. Commun., 2006, 1968; CrossRef (l) C. H. A. Lee and T.-P. Loh, Tetrahedron Lett., 2006, 47, 1641; CrossRef (m) K. R. Prasad and P. Anbarasan, Tetrahedron, 2007, 63, 1089; CrossRef (n) T. Washio, R. Yamaguchi, T. Abe, H. Nambu, M. Anada, and S. Hashimoto, Tetrahedron, 2007, 63, 12037; CrossRef (o) M. Dziedzic and B. Furman, Tetrahedron Lett., 2008, 49, 678; CrossRef (p) M. Pham, A. Allatabaksh, and T. G. Minehan, J. Org. Chem., 2008, 73, 741; CrossRef (q) T. Takeuchi, M. Matsuhashi, and T. Nakata, Tetrahedron Lett., 2008, 49, 6462; CrossRef (r) H. Zhou and T.-P. Loh, Tetrahedron Lett., 2009, 50, 4368; CrossRef (s) A. He, N. Sutivisedsak, and C. D. Spilling, Org. Lett., 2009, 11, 3124; CrossRef (t) B. Schmidt and F. Hölter, Chem. Eur. J., 2009, 15, 11948; CrossRef (u) Ch. R. Reddy, P. P. Madhavi and S. Chandrasekhar, Tetrahedron Asymmetry, 2010, 21, 103; CrossRef (v) D. K. Mohapatra, R. Pal, H. Rahaman, and M. K. Gurjar, Heterocycles, 2010, 80, 219; CrossRef (w) W. Chaładaj, R. Kowalczyk, and J. Jurczak, J. Org. Chem., 2010, 75, 1740. CrossRef
4. For a review, see: S. J. Connon and S. Blechert, Angew. Chem. Int. Ed., 2003, 42, 1900. CrossRef
5. For a review, see: C. F. Nising and S. Bräse, Chem. Soc. Rev., 2008, 37, 1218. CrossRef
6. H. Fuwa, K. Noto, and M. Sasaki, Org. Lett., 2010, 12, 1636. CrossRef
7. S. B. Garber, J. S. Kingsbury, B. L. Gray, and A. H. Hoveyda, J. Am. Chem. Soc., 2000, 122, 8168. CrossRef
8. J. Krauß and D. Unterreitmeier, Arch. Pharm., 2002, 335, 94. CrossRef
9. Kinetic resolution of (±)-4 to prepare optically active (R)-4 has been reported by Spilling and co-workers.3s Thus, our synthetic strategy can also be applicable to an enantioselective synthesis of centrolobine.
10. Hydroxyalkene (±)-4 was used in excess because it is readily available in only one step from anisaldehyde.
11. The carbon numbering is arbitrary.
12. Although the same transformation has already been reported by Chandrasekhar and co-workers,3i we could not reproduce the result under the reported conditions (H2, Pd/C, HCl, EtOH/EtOAc/H2O (5:1:1), room temperature). Accordingly, we examined several reaction conditions and found that THF is the most suitable solvent for the present reaction, although reductive cleavage of the tetrahydropyran ring was still observed under these conditions (< 20%).