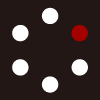
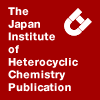
HETEROCYCLES
An International Journal for Reviews and Communications in Heterocyclic ChemistryWeb Edition ISSN: 1881-0942
Published online by The Japan Institute of Heterocyclic Chemistry
e-Journal
Full Text HTML
Received, 29th June, 2010, Accepted, 2nd August, 2010, Published online, 4th August, 2010.
DOI: 10.3987/REV-10-677
■ Selenium-Containing Heterocycles Using Selenoamides, Selenoureas, Selenazadienes, and Isoselenocyanates
Masayuki Ninomiya, Dinesh R. Garud, and Mamoru Koketsu*
Department of Materials Science and Technology, Faculty of Engineering, Gifu University, 1-1 Yanagido, Gifu, Gifu 501-1193, Japan
Abstract
In recent years, considerable attention has been devoted to the synthesis of selenium-containing heterocycles because of their interesting reactivities and potential pharmaceutical applications. The significant starting materials for the selenium-containing heterocycles synthesis are selenoamides, selenoureas, selenazadienes, and isoselenocyanates. This review article introduces the developed synthetic methods mainly on our findings.CONTENTS
1. Introduction
2. Using selenoamide
3. Using selenourea
4. Using selenazadiene
5. Using isoselenocyanate
6. Conclusions
7. Acknowledgements
8. References
1. INTRODUCTION
The selenium element was firstly discovered in 1817,1 and has a form of red amorphous powder, glass-like substance, or gray metal. It was predicted to be a dangerous component causing livestock poisoning2 until it was recognized as an essential nutrient3 found in some selenoproteins in 1950s.4 The chemistry of selenium-containing compounds has not been developed in comparison with that of sulfur-containing compounds because of the instability and strong toxicity of some organoselenium compounds. However, the synthetic study of selenium-containing heterocycles is becoming increasingly interesting on due to their unique reactivities5,6 and diverse biological activities.
To date, it has been reported that selenium-containing heterocycles show anti-cancer,7–10 anti-inflammatory,11,12 anti-melanogenesis,13,14 and neuroprotective15 properties. For example, ebselen (2-phenyl-1,2-benzisoselenazol-3(2H)-one; EB) demonstrated potential as a non-steroidal anti-inflammatory agent acting like a glutathione peroxidase mimic.16–18 Selenazolopyrimidone was prepared and showed anti-tumor activity against mouse leukemia.19 The other examples are potent anti-viral agent selenazofurin (2-β-ribofuranosyl-1,3-selenazole-4-carboxamide),20,21 and histamine H2-agonist ameselamine (2-amino-5-(2-aminoethyl)-4-methyl-1,3-selenazole).22 Besides, our investigations resulted in the preparation of selenazine derivatives with anti-bacterial and anti-tumor effects.23,24 Hence, selenium-containing heterocycles are considered to be not merely chemically interesting, but also medicinally important25–28 and industrially possible for development in the cosmetic and drugs potentially.
Useful key starting materials to access to a broad series of selenium-containing heterocycles in organoselenium chemistry are selenoamides, selenoureas, selenazadienes, and isoselenocyanates.29–33 Our group has reported the synthesis of a variety of selenium-containing heterocycles using them. This review article summarizes in the recent development of such synthesis methods of selenium-containing heterocycles using the selenoamides, selenoureas, selenazadienes, and isoselenocyanates.
2. USING SELENOAMIDE
Selenoamides are widely used in the synthesis of five- and six-membered selenium-containing heterocycles. Because the selenoamides exhibit selenoamide-selenolimidate tautomerism and bear two reactive sites, their investigations are of great interest for the heterocycles synthesis.
The primary selenoamides (1) as starting materials for the synthesis of selenium-containing heterocycles were prepared by the reaction of nitriles with appropriate selenating reagents including phosphorus selenide (P2Se5),34,35 hydrogen selenide (H2Se), Al2Se3, NaSeH,36 tris(trimethylsilyl)monoselenophosphate, and potassium selenobenzoate37 (Scheme 1).
In addition, secondary and tertiary selenoamides (2) were prepared by the reaction of amides with appropriate selenating reagents such as LiAlHSeH,38,39 a mixture of (iBu2AlSe)2 and (iBu2Al)Sen,40,41 (MeAl)2Se,42 selenium-Lawesson’s (Woollins) reagent,43,44 and (Et4N)2WSe445,46 (Scheme 1).
Reaction of primary selenobenzamides (1) with α-haloketones gave various kinds of 2,4-disubstituted 1,3-selenazoles (3).33,34,47–50 Reaction with 3-chloropentane-2,4-dione afforded 5-acetyl-2-alkyl-4-methylselenazoles (4).51,52 Reaction with 1,3-dichloro-2-propanone gave 2-aryl-4-(chloromethyl)selenazoles (5).53,54 Reaction with ethyl bromopyruvate yielded ethyl 2-alkyl-4-selenazolecarboxylates (6).55,56 And reaction with 6-bromo-7,8-dihydro-5(6H)-quinolinone hydrobromide afforded 2-aryl-4,5-dihydroselenazolo[4,5-f]quinolines (7)57 (Scheme 2).
Reaction of primary alkyl58 and aryl59 selenoamides (1) with α-haloacyl halides in pyridine afforded 1,3-selenazol-4-ones (8). When chloro(phenyl)acetyl chloride was used as α-haloacyl halides, the reaction gave 4-hydroxy-5-phenyl-1,3-selenazole (9), the tautomeric enol form of (8) (Scheme 3).
Treatment of primary selenoamides (1: 2.0 equiv.) with hydrazine in methanol for a few days resulted in the formation of 2,5-disubstituted 1,3,4-selenadiazoles (10) (Scheme 4).60
Reaction of primary selenoamides (1) with dimethyl acetylenedicarboxylate (DMAD) afforded 2-aryl-5-(methoxycarbonylmethylene)-4,5-dihydro-1,3-selenazol-4-ones (11) in moderate to high yields. Whereas, reaction of 1 with acetylenedicarboxylic acid gave 2-aryl-5-(carboxymethylene)-4-ethoxy-4,5-dihydro-1,3-selenazol-4-ols (12) (Scheme 5). In this case, the ethoxy group at C4 was introduced by the nucleophilic addition of oxygen of ethanol solvent.61
Many kinds of 4-hydroxy-4H-5,6-dihydro-1,3-selenazines (13) have been prepared in high yields by the reaction of primary selenoamides (1) with α,β-unsaturated ketones or aldehydes in the presence of borontrifluoride diethyl ether complex under mild conditions (Scheme 6). The products consist of diastereomers containing two or three asymmetric centers at the C4, C6, and/or C5 positions of selenazine ring. The predominant diastereomer has a cis relationship between the hydroxy group at C4 and the substituent at C6.62,63
6H-1,3,5-Oxoselenazines (14) were prepared by the reaction of primary selenoamides (1) with aldehydes (2.0 equiv.) in the presence of borontrifluoride diethyl ether complex (Scheme 7).64
Reaction of primary selenoamides (1) with diacyl chloride in the presence of triethylamine afforded 6-hydroxy-1,3-selenazin-4-ones (15) (Scheme 8).58,65
4,6-Bis(dimethylamino)-1,3-selenazinium salts (16) have been prepared by the reaction of primary selenoamides (1) with 1,3-bis(dimethylamino)-1,3-dichloropropenium chloride (Scheme 9).66
P-.F. Zhang et al., have reported the synthesis of 1,3-selenazoles using hypervalent iodine. α-Tosyloxylation of ketones and diketones with [hydroxyl(tosyloxy)iodo]benzene (HTIB), followed by treatment with selenoamides (1) provided convenient methods to prepare 1,3-selenazoles (17 and 18) (Scheme 10).67–69 These synthetic methods were simple, and mild and the yields were high.
3,5-Diaryl-1,2,4-selenadiazoles (19) were prepared in high yields from primary selenoamides (1: 2.0 equiv.) using poly[styrene(iodosodiacetate)] as an oxidant with the advantages of ease of manipulation, short reaction time, high yields, and regeneration and recycling of the polymer reagent with no loss of reactivity (Scheme 11).70
X. Huang et al., have developed a useful way to synthesize the 5-spirocyclopropane-annulated selenazoline-4-carboxylates (20) via tandem Michael addition of primary selenoamides (1) to bromo(cyclopropylidene)acetates followed by an intramolecular substitution under basic conditions.71 In addition, they have reported the synthesis of 6-spirocyclopropane-annulated 4-hydroxyselenazines (21) by the reaction of primary selenoamides (1) with 2-cyclopropylidenepropionaldehyde under the mild conditions without base (Scheme 12).72
K. Geisler et al., have reported efficient methods for the synthesis of 2-unsubstituted 1,3-selenazoles (22 and 23). The elimination of acyl group of 2-acyl-1,3-selenazoles gave the 1,3-selenazoles (22) in good yields, and lower field resonances in the range of 10.13–10.23 ppm were observed for the proton at 2-position in DMSO-d6. The cyclization of selenoformamide (1) with α-bromoketones provided an independent and useful approach to the 1,3-selenazoles (23) (Scheme 13). The advantage of this methodology lies in the fact that only two steps were required.73–75
1,2-Diaza-1,3-butadienes reacted easily with primary selenoamides (1) to afford the cyclized products (24 and 25). Reaction of the (4R*,5S*)-products (25) with 1,8-diazabicyclo[5.4.0]undec-7-ene (DBU) gave 4-methyl-1,3-selenazole-5-carboxylates (26) (Scheme 14). In contrast, the same treatment of (4S*,5S*)-products (24) did not reveal any formation of the selenazole-5-carboxylates (26), suggesting that the aromatization process involved an anti-elimination of the hydrazine moiety.76
Cycloaddition reaction of primary selenoamides (1) via α-selanyl proradienyl cation as intermediate using scandium catalyst yielded 4-(arylmethyl)selenazoles (27). The reaction was completely regioselective, presumably due to nitromethane-H2O system (Scheme 15).77
3. USING SELENOUREA
The use of selenourea as the precursor is one of the most efficient methods for the synthesis of heterocyclic compounds containing selenium atom. Therefore, their preparations and reactions are being actively investigated.
Selenoureas (28–30) are easily prepared by the reaction of isoselenocyanate with amines,78–80 carbodiimides with LiAlHSeH,81 and cyanamides with LiAlHSeH82,83 (Scheme 16).
M. Takahashi, et al., have reported the synthesis of 2-(N,N-diphenylamino)-5,6-diphenyl-5,6-dihydro-4H-1,3-selenazin-4-one (31) by the reaction of N,N-diphenylselenourea (30) with diphenylcyclopropenone at reflux (Scheme 17).84
Reaction of N,N-dialkylselenoureas (30) with chloropropenylidene iminium salt gave 2-amino-1,3-selenazinium salts (32) (Scheme 18).85
2-Amino-4H-5,6-dihydro-1,3-selenazin-4-ones (33) were obtained by the reaction of N,N’-substituted selenoureas (28) with α,β-unsaturated acid chlorides (Scheme 19).86,87
Condensation of unsubstituted selenourea (34: commercial compound) with 1,3-dichloroacetone afforded 2-amino-4-(chloromethyl)-1,3-selenazole hydrochloride (35).88 Reaction with α-haloketones gave 2-amino-1,3-selenazoles (36).22,32,52,89–91 Furthermore, reaction with hydrazonoyl bromides yielded 5-alkylazo-2-aminoselenazoles (37) (Scheme 20).92
Reaction of N,N’-dialkylselenoureas (29) with α-haloacyl halides in pyridine gave 2-imino-1,3-selenazolidin-4-ones (38).93 This type of selenazoles can exist in a tautomeric equilibrium between 2-amino and 2-imino-1,3-selenazolin-4-ones (Scheme 21).
Reaction of selenoureas (30) with chloroacetonitrile afforded 2-amino-4,5-dihydro-1,3-selenazoles (39).34,94 Furthermore, 2-piperidino-1,3-selenazole (40) was yielded by the reaction of 2-piperidino-4,5-dihydro-1,3-selenazol-4-iminium chloride (39) with sodium borohydride (2.0 equiv.). A reaction of 39 in water under reflux gave 2-piperidino-4,5-dihydro-1,3-selenazol-4-one (41) (Scheme 22).94
Several types of 2-amino-1,3-selenazoles (42–46) were prepared by the reaction of N,N-unsubstituted selenoureas (30) with α,β-unsaturated aldehydes,95 ketones,96 α-diketones,97 and ketones98 in the presence of ferric chloride in ethanol (Scheme 23). The formation of the products (43 and 44) could be explained by two pathways; the selenoureas (30) reacted with both β-carbonyl carbons of α,β-unsaturated ketones to give two kinds of the products (43 and 44).
Reaction of N,N-unsubstituted selenoureas (30) with DMAD without any catalyst yielded 2-amino-4,5-dihydro-1,3-selenazol-4-ones (47) (Scheme 24).99
The intramolecular cyclization of N-allylselenoureas (29) afforded five-membered ring 1,3-selenazolidines (48) through 5-exo closure or six-membered ring 1,3-selenazines (49) through 6-endo closure by the treatment of hydrogen chloride or iodine, respectively (Scheme 25).100
Reaction of selenourea (34) with bromomalonaldehyde in water gave 2-amino-1,3-selenazole-5- carbaldehyde (50) (Scheme 26).101
M. Narender et al., have demonstrated the novel and efficient biomimetic conversion of β-keto esters into 2-amino-1,3-selenazoles (51) using easily accessible N-bromosuccinimide and the appropriate selenourea (34) with β-cyclodextrin as a promoter in water.102 2-Amino-4-aryl-1,3-selenazoles (52) were synthesized from α-bromo ketones and unsubstituted selenourea (34) in the presence of β-cyclodextrin in water (Scheme 27).103
3-(2-Amino-1,3-selenazol-4-yl)-2H-chromen-2-ones (53) were prepared by the reaction of selenourea (34) with 3-bromoacetylcoumarins in the presence of CuPy2Cl2 as an efficient Lewis acid catalyst under solvent-free conditions (Scheme 28).104
S. V. Amosova et al., have reported a novel reaction of unsubstituted selenourea (34) with benzoylbromoacetylene proceeded in the presence of triethylamine to afford (E)-2,4-bis(benzoylmethylene)-1,3-diselenetanes (54), (E)-3,5-dibenzoyl-1,4-diselenafulvenes (55), and (Z)-3,5-dibenzoyl-1,4-diselenafulvenes (56), respectively (Scheme 29).105
S. Kolb et al., have prepared selenazolopyrimidine (58). The first stage led to the formation of 3,4-dihydropyrimidine-2-(1H)-selenone derivative (57) by using Biginelli condensation. Cyclocondensation of the derivative with chloroacetyl chloride gave the corresponding product (58) (Scheme 30).106
4. USING SELENAZADIENE
Though selenazadienes are superior starting materials to obtain selenium-containing heterocycles, only a few examples on the synthesis using selenazadienes have been reported in literature.
Our group prepared selenazadienes (59) by condensation of N,N-unsubstituted selenoureas (30) with N,N-dimethylformamide dimethylacetal (1.5 equiv.) at room temperature and shown its utility in the synthesis of selenium-containing heterocycles.107,108 F. Purseigle et. al., have prepared selenazadienes (60) starting from benzonitrile in two steps. Sodium hydrogen selenide was heated in the presence of benzonitrile at 80 oC in pyridine to give selenobenzamide (1). The condensation of the selenobenzamide (1) with N,N-dimethylformamide dimethylacetal resulted in the preparation selenazadienes (60) (Scheme 31).109
Hetero Diels-Alder reaction of selenazadienes (59) with DMAD gave 1,3-selenazol-4-ones (64) in good yields. Six-membered intermediates (61) were converted into five-membered intermediates (63) during
the process of purification using silica gel. Protonation of cycloaddition adducts (61) afforded selenoamidines (62). The amidines (62) were converted into the intermediates (63) by a nucleophilic recyclization (Scheme 32).108
Reaction of selenazadienes (59) with chloroacetonitrile yielded 1,3-selenazol-5-carbonitriles (65) in moderate to high yields (Scheme 33).107
Reaction of selenazadienes (59) with chloroacetyl chloride gave 1,3-selenazol-5-carboxylic acids (66) in good yields. Next, trapping of the intermediate, generated from the reaction of N,N-dimethyl- N’-(piperidinoselenocarbonyl)formamide with chloroacetyl chloride, with various alcohols and amines was investigated. The reactions afforded the corresponding carboxylates and carboxyamides (67) (Scheme 34).108,110
Reaction of selenazadienes (59) with α-haloketones afforded 5-acyl-2-amino-1,3-selenazoles (68). Furthermore, reaction of selenazadienes (59: 2.0equiv.) with 1,3-dichloro-2-propanone in the presence of triethylamine gave the corresponding bis(2-amino-5-selenazoyl) ketones (69) in high yields (Scheme 35).111
The sulfoxonium ylide, prepared in situ by treatment of the trimethylsulfoxonium iodide with sodium hydride in DMF, reacted with the selenazadienes (60) to give the 1,3-selenazol-2-ines (70) (Scheme 36).109
Reaction of selenazadiene (60) with electrophiles such as ethyl bromopyruvate and methoxylacetyl chloride in the presence of triethylamine afforded selenazole (71) and 6H-selenazin-6-one (72) (Scheme 37).109
K. Heuzè et. al., have reported Diels-Alder reaction of selenazadiene and 4,4-diethoxy-2-butyn-1-al which led to the corresponding 4H-1,3-selenazine (73) (Scheme 38).112
5. USING ISOSELENOCYANATE
Isoselenocyanates are included in one of the heterocumulenes, have been emerged as a powerful tool for the synthesis of selenium-containing heterocycles because they are easy to prepare and store, less-toxic, and safe to handle.113 Some years ago, we started a research program concerning the synthetic potential of isoselenocyanates as building blocks for selenoheterocycles. In 2007, our group showed a review of the utility of isoselenocyanates in the synthesis of a variety of four-, five-, and six-membered selenium-containing heterocycles.31 Herein, we introduce the recent progress of the heterocycles using isoselenocyanates by recent articles.
The classical method of synthesis of isoselenocyanates (74) involved the addition of elemental selenium to isonitriles79,114,115 or synthesis from the corresponding formamides.83 More convenient procedure consisted of the treatment of primary amines with CSe2 and HgCl2 in the presence of triethylamine,116 photochemical rearrangement of selecocyanates,117 and via cycloaddition by the reaction of nitrile oxides with primary selenoamides to give the corresponding isoselenocyanates (74) (Scheme 39).
Acyl isoselenocyanates (75) were prepared by the reaction of acyl chlorides with potassium selenocyanate (Scheme 40).118,119
We described the iodocyclization of O-allyl selenocarbamates (76), prepared from isoselenocyanates (74), using I2 or NIS as the electrophile; this led to 4-alkyl-2-imino-1,3-oxaselenolanes, which on the treatment with DBU resulted in the formation (Z)- or (E)-4-alkylidene-2-imino-1,3-oxaselenolanes (77) (Scheme 41).120
Isoselenocyanates (74) reacted with ethyl γ-chloroacetoacetate in the presence of triethylamine to yield 4-oxo-2-amino-4,5-dihydroselenophene-3-carboxylates (78) (Scheme 42).121
J. Fleischhauer et al., have demonstrated the reaction of Δ2-1,2-diazetines with various isoselenocyanates (74 and 75) yielded highly substituted 1,3,4-selenadiazines (79 and 80) (Scheme 43).122
Selenium-oxygen-containing five-, six-, and seven-membered heterocycles were prepared using the corresponding haloalcohols (Scheme 44). One-pot synthesis of 2-imino-1,3-oxaselenolanes (81) has been achieved by the reaction of isoselenocyanates (74) with 2-bromoethanol in good to excellent yields. The thermal rearrangement of 2-imino-1,3-oxaselenolanes (81) to 1,3-selenazolidin-2-ones (82) employing indium and ethyl bromoacetate was observed.123 Reaction with 3-chloropropanol in the presence of sodium hydride afforded 1,3-oxaselenan-2-imines (83). The reaction mechanism via nucleophilic attack of the alcoholate at the isoselenocyanate, followed by an 6-exo-tet cyclization, was most likely.124 The first synthesis of 1,3-oxaselenepanes (84) by the reaction with 4-bromobutanol in the presence of sodium hydride as a one-pot reaction was described. The Z/E isomerism for the exocyclic carbon-nitrogen double bond in the selenium-containing heterocycles was observed.125
One-pot synthesis of 2-imino-5-methylene-1,3-selenazolidines has been achieved by the reaction of isoselenocyanates (74) with propargylamines in high yields (Scheme 45).126
Reaction of acyl isoselenocyanates (75: 2.0 equiv.) with ethyl diazoacetate gave 2-acyl-1,2,3-selenadiazole derivatives (86). On treatment of the products with morpholine, the acyl group at N2 was removed to yield 5-acylamino-1,2,3-selenadiazole-4-carboxylates (87) as 2-unsubstituted forms (Scheme 46).127
G. L. Sommen et al., have reported the reaction of the intermediate ketene N,Se-hemiacatals (88), prepared from cyanomethylene derivatives by treatment with aryl isoselenocyanates (74) and triethylamine, with bis-electrophiles afforded 1,3-selenazinane derivatives (89–91).128,129 Reaction of N,Se-hemiacatals with propargyl chloride in the presence of triethylamine gave 1,3-selenazole derivatives (92) (Scheme 47).130
Reaction of diselenocarbamates (93), generated from isoselenocyanates (74) and sodium hydrogen selenide, with acryloyl chlorides afforded 2-selenoperhydro-1,3-selenazin-4-ones (97).131 The structure of 1,3-selenazin-4-one was confirmed by X-ray analysis. The reaction proceeded via intermediate (94) or (96). The intermediate (94), which was formed by the necleophilic substitution of the acryloyl chloride by the selenium atom of the diselenocarbamate (93), underwent a base catalyzed 1,3-acyl shift to give the rearranged intermediate (95). Finally, The selenium atom attacked the β-carbon of the acrylamide group and the 1,3-selenazines (97) were formed by intramolecular Michael addition (Scheme 48).
6. CONCLUSIONS
In summary, this review provides recent advances in the synthesis of selenium-containing heterocycles using selenoamides, selenoureas, selenazadienes, and isoselenocyanates. A clearer understanding of the reaction details of selenoamides, selenoureas, selenazadienes, and isoselenocyanates will provide chemists with yet further tools for their synthetic endeavors in synthesis of selenium-containing biological active heterocycles.
7. ACKNOWLEDGEMENTS
This work was supported by a Grant-in-Aid for Science Research from the Ministry of Education, Culture, Sports, Science and Technology of Japan (No. 15550030 and 17550099) to which we are grateful.
References
1. J. J. Berzelius, Afhandl. Fys. Kemi Mineral, 1818, 6, 42.
2. C. F. Quin, M. L. Galeas, J. L. Freeman, and E. A. H. Pilon-Smits, Integr. Environ. Assess. Manag., 2007, 3, 460. CrossRef
3. R. Naithani, Mini-Rev. Med. Chem., 2008, 8, 657. CrossRef
4. L. A. Wessjohann, A. Schneider, M. Abbas, and W. Brandt, Biol. Chem., 2007, 388, 997. CrossRef
5. A. R. Katritzky, C. W. Rees, and E. F. V. Scriven, Comprehensive Heterocyclic Chemistry II. A Review of the Literature 1982–1995, Elsevier Science, Oxford, 1996, vol. 1–11.
6. T. Wirth, Organoselenium Chemistry: Modern Development in Organic Synthesis, Springer, Berlin, 2000.
7. W. Wu, K. Murakami, M. Koketsu, Y. Yamada, and I. Sakai, Anticancer Res., 1999, 19, 5375.
8. K. El-Bayoumy and R. Sinha, Mutat. Res., 2004, 551, 181. CrossRef
9. L. Patrick, Altern. Med. Chem., 2004, 9, 239.
10. H. J. Ahn, M. Koketsu, E. M. Yang, Y. M. Kim, H. Ishihara, and H. O. Yang, J. Cell. Biochem., 2006, 99, 807. CrossRef
11. K. N. Nam, M. Koketsu, and E. H. Lee, Eur. J. Pharmacol., 2008, 589, 53. CrossRef
12. S. Y. Choi, Y. O. Jo, M. Koketsu, H. Ishihara, S. H. Kim, and S. Y. Kim, J. Korean Soc. Appl. Biol. Chem., 2009, 52, 371. CrossRef
13. M. Koketsu, S. Y. Choi, H. Ishihara, B. O. Lim, H. Kim, and S. Y. Kim, Chem. Pharm. Bull., 2002, 50, 1594. CrossRef
14. E. H. Lee, Y.-J. Lim, S. K. Ha, T. H. Kang, M. Koketsu, C. H. Kang, S. Y. Kim, and J.-H. Park, J. Pharm. Pharmacol., 2010, 62, 352.
15. A. Nishina, A. Sekiguchi, R. Fukumoto, M. Koketsu, and S. Furukawa, Biochem. Biophys. Res. Commun., 2007, 352, 360. CrossRef
16. H. Sies and H. Masumoto, Adv. Pharmacol., 1997, 26, 1153.
17. G. Mugesh and H. B. Singh, Chem. Soc. Rev., 2000, 29, 347. CrossRef
18. Y. Nakamura, Q. Feng, T. Kumagai, H. Ohigashi, T. Osawa, N. Noguchi, E. Niki, and K. Uchida, J. Biol. Chem., 2002, 277, 2687. CrossRef
19. H. Ito, J. Z. Wang, K. Shimura, J. Sakakihara, and T. Ueda, Anticancer Res., 1990, 10, 891.
20. S. K. Wray, R. H. A. Smith, B. E. Gilbert, and V. Knight, Antimicrob. Agents Chemother., 1986, 29, 67.
21. D. F. Smee, J. H. Huffman, L. L. Hall, J. W. Huggins, and R. W. Sidwell, Antiviral Chem. Chemother., 1990, 1, 211.
22. van der H. Goot, J. C. Ericks, R. Leurs, and H. Timmerman, Bioorg. Med. Chem. Lett., 1994, 4, 1913. CrossRef
23. M. Koketsu, H. Ishihara, and M. Hatsu, Res. Commun. Mol. Pathol. Pharmacol., 1998, 101, 179.
24. M. Koketsu, H. Ishihara, W. Wu, M. Koji, and I. Saiki, Eur. J. Pharm. Sci., 1999, 9, 157. CrossRef
25. Y.-J. Park, M. Koketsu, J. M. Kim, J.-H. Yeo, and H. Ishihara, Biol. Pharm. Bull., 2003, 26, 1657. CrossRef
26. A. Sekiguchi, A. Nishina, H. Kimura, R. Fukumoto, K. Kanoh, H. Ishihara, and M. Koketsu, Chem. Pharm. Bull., 2005, 53, 1439. CrossRef
27. A. Sekiguchi, A. Nishina, H. Kimura, R. Fukumoto, M. Kogami, H. Ishihara, and M. Koketsu, Biol. Pharm. Bull., 2006, 29, 1404. CrossRef
28. H. Tsukagoshi, M. Koketsu, M. Kato, M. Kurabayashi, A. Nishina, and H. Kimura, FEBS Journal, 2007, 274, 6046. CrossRef
29. M. Koketsu and H. Ishihara, Curr. Org. Chem., 2003, 7, 175. CrossRef
30. M. Koketsu and H. Ishihara, Curr. Org. Synthesis, 2006, 3, 439. CrossRef
31. D. R. Garud, M. Koketsu, and H. Ishihara, Molecules, 2007, 12, 504. CrossRef
32. A. R. Katritzky, C. A. Ramsden, E. F. V. Scriven, and R. J. K. Taylor, Comprehensive Heterocyclic Chemistry III. A Review of the Literature 1995–2007, Elsevier Science, Oxford, 2008, vol. 2, pp. 463–477.
33. A. R. Katritzky, C. A. Ramsden, E. F. V. Scriven, and R. J. K. Taylor, Comprehensive Heterocyclic Chemistry III. A Review of the Literature 1995–2007, Elsevier Science, Oxford, 2008, vol. 4, pp. 755–821.
34. K. Geisler, W.-D. Pfeiffer, A. Künzler, H. Below, E. Bulka, and P. Langer, Synthesis, 2004, 875. CrossRef
35. K. Geisler, A. Jacobs, A. Künzler, M. Mathes, I. Girrleit, B. Zimmermann, E. Bulka, W.-D. Pfeiffer, and P. Langer, Synlett, 2002, 1983. CrossRef
36. X. R. Zhao, M. D. Ruan, W. Q. Fan, and X. J. Zhou, Synth. Commun., 1994, 24, 1761. CrossRef
37. H. Ishihara, K. Yoshimura, and M. Koketsu, Chem. Lett., 1998, 1287. CrossRef
38. H. Ishihara, M. Koketsu, Y. Fukuda, and F. Nada, J. Am. Chem. Soc., 2001, 123, 8408. CrossRef
39. M. Koketsu, Y. Okayama, H. Aoki, and H. Ishihara, Heteroatom Chem., 2002, 13, 195. CrossRef
40. G. M. Li and R. A. Zingaro, J. Chem. Soc., Perkin Trans. 1, 1998, 647. CrossRef
41. G. M. Li and R. A. Zingaro, Phosphorus, Sulfur Silicon Relat. Elem., 1998, 136, 525.
42. G. M. Li, R. A. Zingaro, M. Segi, J. H. Reibenspies, and T. Nakajima, Organometallics, 1997, 16, 756. CrossRef
43. P. T. Wood and J. D. Woollins, J. Chem. Soc., Chem. Commun., 1988, 1190. CrossRef
44. P. Bhattacharyya and J. D. Woollins, Tetrahedron Lett., 2001, 42, 5949. CrossRef
45. A. Müller, Angew. Chem., Int. Ed. Engl., 1981, 20, 934. CrossRef
46. V. Saravanan, C. Mukherjee, S. Das, and S. Chandrasekaran, Tetrahedron Lett., 2004, 45, 681. CrossRef
47. V. I. Cohen, Synthesis, 1979, 66. CrossRef
48. L. L. Lai and D. H. Reid, Synthesis, 1993, 870. CrossRef
49. R. N. Hanson, R. W. Giese, M. A. Davis, and S. M. Costello, J. Med. Chem., 1978, 21, 496. CrossRef
50. V. P. Litvinov and V. D. Dyachenko, Russ. J. Org. Chem., 1999, 35, 1377.
51. A. Shafiee, A. Shafaati, and B. Habibi-Khameneh, J. Heterocycl. Chem., 1989, 26, 709. CrossRef
52. A. Shafiee, M. A. Ebrahimzadeh, and A. Maleki, J. Heterocycl. Chem., 1999, 36, 901. CrossRef
53. A. Shafiee, A. Mazloumi, and V. I. Cohen, J. Heterocycl. Chem., 1979, 16, 1563. CrossRef
54. A. Silberg, I. Siniti, and H. Mantsch, Chem. Ber., 1961, 94, 2887. CrossRef
55. P. W. K. Woo, J. Labelled Compd. Radiopharm., 1988, 25, 1149. CrossRef
56. P. D. Cook and D. J. McNamara, J. Heterocycl. Chem., 1986, 23, 155. CrossRef
57. A. Shafiee and M. Rezayazdi, J. Heterocycl. Chem., 1995, 32, 177. CrossRef
58. M. Koketsu, Y. Takenaka, and H. Ishihara, Synthesis, 2001, 731. CrossRef
59. M. Koketsu, Y. Takenaka, and H. Ishihara, Heteroatom Chem., 2003, 14, 106. CrossRef
60. V. I. Cohen, J. Heterocycl. Chem., 1979, 16, 365. CrossRef
61. M. Koketsu, T. Sasaki, H. Ando, and H. Ishihara, J. Heterocycl. Chem., 2007, 44, 231. CrossRef
62. M. Koketsu, T. Senda, K. Yoshimura, and H. Ishihara, J. Chem. Soc., Perkin Trans. 1, 1999, 453. CrossRef
63. M. Koketsu, Y. Takenaka, S. Hiramatsu, and H. Ishihara, Heterocycles, 2001, 55, 1181. CrossRef
64. M. Sekiguchi, A. Ogawa, S. Fujiwara, I. Ryu, N. Kambe, and N. Sonoda, Chem. Lett., 1990, 913. CrossRef
65. M. Koketsu, S. Hiramatsu, and H. Ishihara, Chem. Lett., 1999, 485. CrossRef
66. I. Shibuya and H. Nakanishi, Bull. Chem. Soc. Jpn., 1987, 60, 2686. CrossRef
67. P.-F. Zhang and Z.-C. Chen, Synthesis, 2000, 1219. CrossRef
68. P.-F. Zhang and Z.-C. Chen, J. Heterocycl. Chem., 2001, 38, 503. CrossRef
69. G.-B. Zhou, P.-F. Zhang, Z.-C. Chen, and Y.-J. Pan, Chem. J. Internet, 2004, 6, 63.
70. X. Huang and J. Chen, Synth. Commun., 2003, 33, 2823. CrossRef
71. X. Huang, W.-L. Chen, and H.-W. Zhou, Synlett, 2004, 329. CrossRef
72. X. Huang, L. Yu, and Z.-H. Chen, Synth. Commun., 2005, 35, 1253. CrossRef
73. K. Geisler, A. Künzler, H. Below, E. Bulka, W.-D. Pfeiffer, and P. Langer, Synlett, 2003, 1195. CrossRef
74. K. Geisler, A. Künzler, H. Below, E. Bulka, W.-D. Pfeiffer, and P. Langer, Synthesis, 2004, 97. CrossRef
75. H. Below, W.-D. Pfeiffer, K. Geisler, M. Lalk, and P. Langer, Eur. J. Org. Chem., 2005, 3637. CrossRef
76. O. A. Attanasi, P. Filippone, F. R. Perrulli, and S. Santeusanio, Eur. J. Org. Chem., 2002, 2323. CrossRef
77. M. Yoshimatsu, T. Yamamoto, A. Sawa, T. Kato, G. Tanabe, and O. Muraoka, Org. Lett., 2009, 11, 2952. CrossRef
78. D. Keil and H. Hartmann, Synthesis, 2004, 15. CrossRef
79. M. Koketsu, N. Suzuki, and H. Ishihara, J. Org. Chem., 1999, 64, 6473. CrossRef
80. J. G. Fernández-Bolaños, Ó. López, V. Ulgar, I. Maya, and J. Fuentes, Tetrahedron Lett., 2004, 45, 4081. CrossRef
81. M. Koketsu, N. Takakura, and H. Ishihara, Synth. Commun., 2002, 32, 3075. CrossRef
82. M. Koketsu, Y. Fukuta, and H. Ishihara, Tetrahedron Lett., 2001, 42, 6333. CrossRef
83. M. Koketsu, Y. Fukuta, and H. Ishihara, J. Org. Chem., 2002, 67, 1008. CrossRef
84. M. Takahashi, S. Watanabe, and T. Kasai, Heterocycles, 1980, 14, 1921. CrossRef
85. J. Leibscher and H. Hartmann, Tetrahedron Lett., 1976, 24, 2005. CrossRef
86. M. Koketsu, M. Taura, and H. Ishihara, J. Heterocycl. Chem., 2004, 41, 783. CrossRef
87. K. Kanoh, H. Ishihara, and M. Koketsu, Synthesis, 2007, 2617. CrossRef
88. R. N. Hanson and M. A. Davis, J. Heterocycl. Chem., 1981, 18, 205. CrossRef
89. S. Archer and R. McGarry, J. Heterocycl. Chem., 1982, 19, 1245. CrossRef
90. R. N. Hanson, J. Heterocycl. Chem., 1984, 21, 57. CrossRef
91. A. M. Farag, K. M. Dawood, Z. E. Kandeel, and M. S. Algharib, J. Chem. Res., Synop., 1996, 530.
92. H. M. Hassaneen, A. M. Farag, M. S. Algaharib, and A. S. Shawali, Org. Prep. Proced. Int., 1988, 20, 505. CrossRef
93. M. Koketsu, F. Nada, and H. Ishihara, Synthesis, 2002, 195. CrossRef
94. M. Koketsu, H. Tanaka, and H. Ishihara, Chem. Lett., 2005, 34, 1260. CrossRef
95. M. Koketsu, K. Kanoh, and H. Ishihara, Heterocycles, 2006, 68, 2647. CrossRef
96. M. Koketsu, K. Kanoh, and H. Ishihara, Heterocycles, 2006, 68, 2145. CrossRef
97. M. Koketsu, K. Kanoh, and H. Ishihara, Heterocycles, 2007, 74, 1009. CrossRef
98. M. Koketsu, K. Kanoh, H. Ando, and H. Ishihara, Heteroatom Chem., 2006, 17, 88. CrossRef
99. M. Koketsu, K. Kanoh, and H. Ishihara, Heterocycles, 2006, 68, 2627. CrossRef
100. M. Koketsu, T. Kiyokuni, T. Sakai, H. Ando, and H. Ishihara, Chem. Lett., 2006, 626. CrossRef
101. M. A. Salvador, L. V. Reis, P. Almeida, and P. F. Santos, ARKIVOC, 2008, 90.
102. M. Narender, M. S. Reddy, V. P. Kumar, B. Srinivas, R. Sridhar, Y. V. D. Nageswar, and K. R. Rao, Synthesis, 2007, 3469. CrossRef
103. M. Narender, M. S. Reddy, V. P. Kumar, V. P. Reddy, Y. V. D. Nageswar, and K. R. Rao, J. Org. Chem., 2007, 72, 1849. CrossRef
104. J. V. Madhav, B. S. Kuarm, and B. Rajitha, Synth. Commun., 2008, 38, 3514. CrossRef
105. S. V. Amosova, V. N. Elokhina, A. S. Nakhmanovich, L. I. Larina, A. V. Martynov, B. R. Steele, and V. A. Potapov, Tetrahedron Lett., 2008, 49, 974. CrossRef
106. S. Kolb, O. Mondésert, M.-L. Goddard, D. Jullien, B. O. Villoutreix, B. Ducommun, C. Garbay, and E. Braud, ChemMedChem, 2009, 4, 633. CrossRef
107. M. Koketsu, F. Nada, T. Mio, and H. Ishihara, Heterocycles, 2003, 60, 1211. CrossRef
108. M. Koketsu, M. Imagawa, T, Mio, and H. Ishihara, J. Heterocycl. Chem., 2005, 42, 831. CrossRef
109. F. Purseigle, D. Dubreuil, A. Marchand, J. P. Pradère, M. Goli, and L. Toupet, Tetrahedron, 1998, 54, 2545. CrossRef
110. M. Koketsu, T. Mio, and H. Ishihara, Synthesis, 2004, 233. CrossRef
111. M. Koketsu, M. Kogami, H. Ando, and H. Ishihara, Synthesis, 2006, 31. CrossRef
112. K. Heuzè, F. Purseigle, D. Dubreuil, and J. P. Pradère, Synth. Commun., 1998, 28, 301. CrossRef
113. H. Heimgartner, Y. Zhou, P. K. Atanassov, K. Plamen, and G. L. Sommen, Phosphorus, Sulfur Silicon Relat. Elem., 2008, 183, 840. CrossRef
114. E. Bulka, K.-D. Ahlers, and E. Tucek, Chem. Ber., 1967, 100, 1367. CrossRef
115. D. H. R. Barton, S. I. Parekh, M. Tajbakhsh, E. A. Theodorakis, and C.-L. Tse, Tetrahedron, 1994, 50, 639. CrossRef
116. L. Henriksen and U. Ehrbar, Synthesis, 1976, 519. CrossRef
117. H. Suzuki, M. Usuki, and T. Hanafusa, Synthesis, 1979, 705. CrossRef
118. I. B. Douglas, J. Am. Chem. Soc., 1937, 59, 740. CrossRef
119. M. Koketsu, Y. Yamamura, H. Aoki, and H. Ishihara, Phosphorus, Sulfur Silicon Relat. Elem., 2006, 181, 2699. CrossRef
120. D. R. Garud, M. Makimura, H. Ando, H. Ishihara, and M. Koketsu, Tetrahedron Lett., 2007, 48, 7764. CrossRef
121. G. L. Sommen, A. Linden, and H. Heimgartner, Lett. Org. Chem., 2007, 4, 7. CrossRef
122. J. Fleischhauer, R. Beckert, W. Günther, S. Kluge, S. Zahn, J. Weston, D. Berg, and H. Görls, Synthesis, 2007, 2839. CrossRef
123. Y. Toyoda, D. R. Garud, and M. Koketsu, Heterocycles, 2009, 78, 449. CrossRef
124. G. L. Sommen and H. Heimgartner, Polish J. Chem., 2007, 81, 1413.
125. D. R. Garud, Y. Toyoda, and M. Koketsu, Tetrahedron Lett., 2009, 50, 3035. CrossRef
126. M. Koketsu, T. Sakai, T. Kiyokuni, D. R. Garud, H. Ando, and H. Ishihara, Heterocycles, 2006, 68, 1607. CrossRef
127. H. Heimgartner, Y. Zhou, P. K. Atanassov, and G. L. Sommen, Phosphorus, Sulfur Silicon, 2008, 183, 840. CrossRef
128. G. L. Sommen, A. Linden, and H. Heimgartner, Helv. Chim. Acta, 2007, 90, 472. CrossRef
129. G. L. Sommen, A. Linden, and H. Heimgartner, Helv. Chim. Acta, 2007, 90, 1849. CrossRef
130. G. L. Sommen, A. Linden, and H. Heimgartner, Helv. Chim. Acta, 2008, 91, 209. CrossRef
131. D. R. Garud, N. Tanahashi, M. Ninomiya, and M. Koketsu, Tetrahedron, 2009, 65, 4775. CrossRef