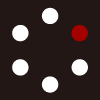
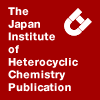
HETEROCYCLES
An International Journal for Reviews and Communications in Heterocyclic ChemistryWeb Edition ISSN: 1881-0942
Published online by The Japan Institute of Heterocyclic Chemistry
e-Journal
Full Text HTML
Received, 5th July, 2010, Accepted, 7th September, 2010, Published online, 8th September, 2010.
DOI: 10.3987/COM-10-12007
■ Alkylations of 10H-2,7-Diazaphenothiazine to Alkyl-2,7-diazaphenothiazinium Salts and 7-Alkyl-2,7-diazaphenothiazines
Beata Morak-Młodawska, Krystian Pluta,* Kinga Suwińska, and Małgorzata Jeleń
Department of Organic Chemistry, The Medical University of Silesia, Jagielloñska Str. 4, 41-200 Sosnowiec, Poland
Abstract
Alkylations of 10H-2,7-diazaphenothiazine (1) with alkyl halides led to different products depending on the reaction conditions: 10-methyl derivative (2), 2,10-dimethyl-2,7-diazaphenothiazinium and 7,10-dimethyl-2,7-diazapheno-thiazinium iodides (3-4), 2,7-dialkyl-2,7-diazaphenothiazidiinium dihalides (5-8) and 7-alkyl-2,7-diazaphenothiazines (9-12). The last compounds were the isomers of recently published 10-alkyl-2,7-diazaphenothiazines. 2,7-Dialkyl-2,7-diazaphe-nothiazidiinium dihalides (5) and (8) were transformed into 7-alkyl-2,7-diazaphe-nothiazines (9) and (12) in basic conditions. The structures of the products were determined on the basis of physical properties, 1H NMR (ROESY, COSY) and MS spectra, and were confirmed by X-ray analysis of compounds (3-5).INTRODUCTION
Phenothiazines are an important class of heterocyclic compounds because of significant biological activities such as antipsychotic, antihistaminic, antitussive and antiemetic and interesting chemical properties.1 Recent reports deal with anticancer, antiplasmid and antibacterial activities, reversal of multidrug resistance (MDR) and potential treatment in Alzheimer’s, Creutzfeldt-Jakob and AIDS diseases of classical and new synthesized phenothiazines.2-10 A number of new phenothiazines have been obtained by modifications of the parent phenothiazine structure by an introduction of a new substituent at the thiazine nitrogen atom or at the benzene ring and by a substitution of one or two benzene rings with homoaromatic and heteroaromatic rings (most often azine rings).9-12
In our previous papers13,14 we described synthesis of new type of azaphenothiazine, 10H-dipyrido[3,4-b;-3’,4’-e][1,4]thiazine, (named also as 10H-2,7-diazaphenothiazine (1)) and its 10-alkyl, aryl, heteroaryl and dialkylaminoalkyl derivatives. The parent compound (1) and 10-methyl derivative (2) (less) showed promising anticancer activity against lung cancers HOP-62 and HOP-92, colon cancers COLO 205 and HCT-116, renal cancers RXF393 and A498, and leukemia HL-60(TB).15 Compounds (1) and (2) showed also immunosuppressive activity.16
As compound (1) possesses three nitrogen atoms, we studied possibilities of alkylations of two pyridine nitrogen atoms to form 2,7-, 2,10- and 7,10-dialkyl-2,7-diazaphenothiazinium salts and 7-alkyl-2,7-diazaphenothiazines.
RESULTS AND DISCUSSION
There is only a few reports17-21 on the alkylations of other nitrogen atoms (=N- in azines) than the thiazine ones (=NH) in azaphenothiazines. We found that when methylation of the parent compound (1) with methyl iodide in basic conditions (DMF, NaH) at room temperature was prolonged (from 24 h to 72 h), not only the product of the thiazine nitrogen atom methylation (10-methylderivative (2)) was obtained (in 19% yield) but first of all the products of methylation of both the thiazine and the pyridine nitrogen atoms: 2,10-dimethyl-2,7-diazaphenothiazinium iodide (3) and 7,10-dimethyl-2,7-diazaphenothiazinium iodide (4) in 51% yield (Scheme 1).
Compounds (3) and (4) turned out to be inseparable and what more they formed monocrystals containing both the compounds in the ratio of 1:1. In order to confirm their structures, X-ray analysis was carried out (Figure 1).
When the alkylations with alkyl halides (methyl, ethyl and butyl iodides and benzyl chloride) in ethanol (without a base) at room or elevated temperature (depending on the alkylating agents), 2,7-dialkyl-2,7-diazaphenothiazidiinium dichloride and diiodides (5–8, in 30-41% yield), and 7-alkyl-2,7-diazaphenothiazines (9–12, in 25-37% yield) were obtained (Scheme 2).
In those conditions the NH group of the thiazine ring was not alkylated but only the pyridine nitrogen atoms.
To confirm which nitrogen atoms were alkylated and to exclude the isomeric structure (13) (Figure 1), X-ray analysis of disalt (5) was carried out (Figure 4).
7-Methyl- and 7-benzyl-2,7-diazaphenothiazines (9) and (12) were obtained directly from salts (5) and (8) in 68% and 70% yields in the action of aqueous sodium hydroxide, respectively.
Physical and spectroscopic properties of 2,7-diazaphenothiazines
All alkylations were monitored by TLC analysis. The chromatograms of 2,7-diazaphenothiazinium halides (3-8) and 7-alkyl-2,7-diazaphenothiazines (9-12) showed colored spots [(3-4) - bright yellow, (5-6) - bright orange, (7) - orange, (8) - intensive orange, (9-10) - intensive yellow, (11) - yellow and (12) - canary yellow] which became more intensive during irradiation with UV lamp. When these chromatograms were sprayed with a phenothiazine detection mixture (sulfuric acid-water-ethanol 1:1:8),22 unlike to the chromatograms of 10-alkyl-2,7-diazaphenothiazines,13 the spot color disappeared for compound (3-8) or became bright yellow (9-12). The Rf values of 7-alkyl-2,7-diazaphenothiazines (9–12) were lower than analogous values of isomeric 10-alkyl-2,7-diazaphenothiazines. In contrary to 10-methyl-2,7-diazaphenothiazine (2) and 7-alkyl-2,7-diazaphenothiazines (9–12), salts (3–8) were soluble in water. The Beilstein test of salts (3–8) showed the presence of the halogen atom and the reactions with silver nitrate showed the chlorine and iodine atoms of anionic nature.
The 1H NMR spectra of the obtained azaphenothiazines were recorded in deuteriochloroform or dimethyl sulphoxide-d6 and revealed two singlet signals and four doublet signals showing unsymmetrical structure of the diazaphenothiazine system. Two singlet signals and two doublet signals were considered as α-pyridinyl proton ones, two doublet signals as β-pyridinyl proton ones. The structure of 7-alkyl-2,7-diazaphenothiazines (9–12) was based on the unquestionable assignment of the protons with the help of homonuclear 1H-1H ROESY and COSY experiments for 7-ethyl derivative (10). Four signals aromatic proton signals at 6.58, 7.45, 8.02 and 8.09 ppm were doublets and two signals at 7.31 and 8.32 ppm were singlets. The ethyl group signals were found as a triplet at 1.58 ppm (CH3) and a quartet (CH2) at 4.21 ppm. ROESY experiment showed correlation of the CH2 and CH3 groups with each other and with the singlet proton at 7.31 ppm and the doublet proton at 8.09 ppm, which were assigned as H6 and H8 protons, respectively (Figure 2). H8 proton correlated (ROESY, COSY) with the doublet proton at 7.45 ppm (J = 6.8 Hz) assigned as H9. The most upfield doublet proton at 6.58 ppm (being β-pyridinyl proton) correlated (ROESY, COSY) with the doublet proton at 8.02 ppm (J = 5.1 Hz) and both protons were assigned as H4 and H3, respectively.
All diazaphenothiazines and their salts (3–12) show promising potential antiinflammatory, anticancer, antihistaminic, antiviral, cardiotonic and immunomodulating activities.23
X-Ray study
There are only two reports on the crystal structure of azaphenothiazinium salts (quinobenzothiazinium and pyridoquinothiazinium chlorides) possessing different molecular conformations.24,25 The X-ray study of compounds (3–5) confirmed their structures as 2,10- and 7,10-dimethyldiazaphenothiazinium iodides (monosalts, Figure 3) and 2,7-dimethyldiazaphenothiazidiinium diiodide (disalt, Figure 4).
As was mentioned above, monosalts (3) and (4) form inseparable mixture and both tricyclic systems are arranged alternatively in the molecular unit. Both compounds are folded along the N···S axis. The folding angles between the planes of the two halves of the thiazine ring (i. e. S1/C3/C4/N3 and S1/C13/C14/N3) are 143.2(3) and 144.9(4)o for compounds (3) and (4), respectively. Similarly, the angle between the pyridine ring planes are 147.6(2) and 149.7(2)o, respectively. The central thiazine ring is in the boat conformation with the methyl group in equatorial location with the C18–N3···S1 angle of 170.2(2) and 167.1(2)o for compounds (3) and (4), respectively. The methyl groups of the external rings are coplanar with the pyridine rings with the torsion angles C13–C12–N2–C17 and C15–C16–N2–C17 of 179.3(7) and -178.2(7)o for compound (3), and 179.2(7) and 180.0(7)o for compound (4). Whereas the C–S–C angle is similar in both compounds (97.7(3) and 97.6(3)o), the C–N3–C angle is different (118.7(6) and 120.2(6)o). Similarly the C–S bonds lengths are equal in compound (4) but different in compound (3). The N3–C bonds lengths are different in both compounds (Table 1). The N3–CH3 bond lengths are significantly shorter than those N–CH3 bond lengths in the pyridine rings. These geometric parameters show significant contribution of resonance structures (3’) and (4’) in the molecules (Scheme 4).
The 1:1 mixture of (4) and (5) crystallizes with two water molecules. In the crystal the two types of diazaphenothiazinium cations are stacked alternately in columns along crystallographic a axis is (Figure 5). The distance between the r.m.s. planes of tricyclic systems of two neighbouring molecules in the stack is 3.61 Å. Between the columns along the b axis infinite 1D chains of hydrogen bonded water and iodide species are located (Table 2).
In contrast to monosalts (3) and (4), disalt (5) is planar what is the result of an increase of the C–N–C and C–S–C angles in central ring (123.53(6) and 100.98(6)o, respectively) in comparison to the values mentioned above for those compounds.
The N–CH3 and N–C bond lengths are the same or similar but the C–S bonds are different (Table 1). The molecule is located on crystallographic center of symmetry and, due to such location, is disordered statistically 50:50 over two positions (Figure 4). Water molecules and iodide anions are hydrogen bonded to form 1D infinite chains along crystallographic [101] direction. Additionally, the water molecules are acceptors of hydrogen of diazaphenothiazinium cations just extending from 1D to 2D hydrogen bond pattern (Table 2).
CONCLUSION
We report here synthesis of 2,7-, 2,10- and 7,10-dialkyl-2,7-diazaphenothiazinium monosalts and disalts (3–8), and 7-alkyl-2,7-diazaphenothiazines (9–12) from 10H-2,7-diazaphenothiazine (1) depending on the reaction conditions. The structures of products were determined using NOE and COSY experiments and X-ray analysis of the selected compounds.
EXPERIMENTAL
Melting points were determined in open capillary tubes on a Boetius melting point apparatus and are uncorrected. The 1H NMR spectra were recorded on a Varian Unity-Inova-300 and a Bruker DRX spectrometers at 300 and 500 MHz in deuteriochloroform and dimethyl sulphoxide-d6 with tetramethylsilane as the internal standard. Fast Atom Bombardment mass spectra (FAB MS) were run in glycerol on a Finnigan MAT 95 spectrometer at 70 eV. The thin layer chromatography was performed on silica gel 60 F254 (Merck 1.05735) with CHCl3-EtOH (5:1 and 10:1 v/v) and on aluminum oxide 60 F254 neutral (type E) (Merck 1.05581) with CHCl3-EtOH (10:1 v/v) as eluents.
Synthesis of 10-methyl-2,7-diazaphenothiazine (2), 2,10-dimethyl-2,7-diazaphenothiazinium and 7,10-dimethyl-2,7-diazaphenothiazinium iodides (3) and (4)
To a stirred solution of 10H-2,7-diazaphenothiazine (1) (100 mg, 0.5 mmol) in dry DMF (5 mL) NaH (12 mg, 0.5 mmol, washed out with hexane) was added. The reaction mixture was stirred at rt for 1 h, methyl iodide (0.39 mL, 6 mmol) was added in 3 portions and the stirring was continued for 72 h. The solvent was distilled off in vaccuo, the residue was dissolved in CHCl3 and was purified by column chromatography (aluminum oxide, CHCl3-EtOH 5:1) to give:
1. 10-Methyl-2,7-diazaphenothiazine (2) (20 mg. 19%); mp 56-57 °C (EtOH) (lit.,13 mp 56-57 °C).
2. 2,10-Dimethyl-2,7-diazaphenothiazinium iodide (3) and 7,10-dimethyl-2,7-diazaphenothiazinium iodide (4) (a mixture 1:1, 90 mg, 51%). 1H NMR (DMSO-d6) δ: 3.09 (s, 3H, CH3), 3.49 (s, 3H, CH3), 3.98 (s, 3H, CH3), 4.16 (s, 3H, CH3), 7.03 (d, J = 5.4 Hz, 1H), 7.31 (d, J = 6,6 Hz, 1H), 7.32 (d, J = 5.4 Hz, 1H), 7.83 (d, J = 6.0 Hz, 1H), 8.21 (s, 1H,), 8.27 (d, J = 5.4 Hz, 1H), 8.32 (s, 1H), 8.33 (s, 1H), 8.36 (d, J = 5.4 Hz, 1H), 8.42 (d, J = 6.0 Hz, 1H), 8.46 (s, 1H), 8.48 (d, J= 6.6 Hz, 1H). FAB MS m/z: 231 (M+1–I, 30), 230 (M+1–HI, 100), 216 (M+1–CH3I, 12). Anal. Calcd for C12H12IN3S: C, 40.35; H, 3.39; N, 11.76. Found C, 40.12; H, 3.21; N, 11.51.
Synthesis of 2,7-dimethyl-2,7-diazaphenothiazidiinium diiodide (5) and 7-methyl-2,7-diazapheno-thiazine (9)
To a stirred solution of 10H-2,7-diazaphenothiazine (1) (100 mg, 0.5 mmol) in dry EtOH (5 mL) at rt, methyl iodide (0.09 mL, 1.5 mmol) was added and the stirring was continued for 48 h. The resulted solid was filtered off to give 2,7-dimethyl-2,7-diazaphenothiazidiinium diiodide (5) (100 mg, 41%), mp 249-250 °C (EtOH). 1H NMR (DMSO-d6) δ: 3.86 (s, 3H, CH3), 4.06 (s, 3H, CH3), 6.78 (d, J = 6.5 Hz, 1H, H4 or H9), 7.68 (d, J = 6.5 Hz, 1H, H4 or H9), 7.70 (s, 1H, H1 or H6), 8.15 (d, J = 6.5 Hz, 1H, H3 or H8), 8.29 (d, J = 6.5 Hz, 1H, H3 or H8), 8.31 (s, 1H, H1 or H6), 11.02 (s, 1H, NH). FAB MS m/z: 230 (M+1–2HI, 100), 216 (M+1–HI and CH3I, 24). Anal. Calcd for C12H13I2N3S: C, 29.71; H, 2.70; N, 8.66. Found C, 29.47; H, 2.60; N, 8.52.
The filtrate was concentrated and purified by column chromatography (aluminum oxide, CHCl3-EtOH 5:1) to give 7-methyl-2,7-diazaphenothiazine (9) (40 mg, 37%), mp 69-70 °C (EtOH). 1H NMR (CDCl3) δ: 3.89 (s, 3H, CH3), 6.59 (d, J = 4.2 Hz, 1H, H4), 7.12 (s, 1H, H6 ), 7.46 (d, J = 6.1 Hz, 1H, H9), 7.71 (d, J = 4.2 Hz, 1H, H3), 8.03 (d, J = 6.1 Hz, 1H, H8), 8.37 (s, 1H, H1). EI MS m/z: 215 (M, 100), 200 (M–CH3, 52). Anal. Calcd for C11H9N3S: C, 61.37; H, 4.21; N, 19.52. Found C, 61.22; H, 4.14; N, 19.38.
Synthesis of 2,7-diethyl-2,7-diazaphenothiazidiinium diiodide (6) and 7-ethyl-2,7-diazapheno-thiazine (10)
To a stirred solution of 10H-2,7-diazaphenothiazine (1) (100 mg, 0.5 mmol) in dry EtOH (5 mL) at rt, ethyl iodide (0.24 mL, 3 mmol) was added and the stirring was continued at 45 oC for 120 h. The resulted solid was filtered off to give 2,7-diethyl-2,7-diazaphenothiazidiinium diiodide (6) (69 mg, 36%), mp 218-219 °C (EtOH). 1H NMR (DMSO-d6) δ: 1.41 (t, J = 7.2 Hz, 3H, CH3), 1.53 (t, J = 7.2 Hz, 3H, CH3), 4.21 (q, J = 7.2 Hz, 2H, CH2), 4.56 (q, J = 7.2 Hz, 2H, CH2), 6.80 (d, J = 6.5 Hz, 1H, H4 or H9), 7.64 (d, J = 6.5 Hz, 1H, H4 or H9), 7.71 (s, 1H, H1 or H6), 8.15 (d, J = 6.5 Hz, 1H, H3 or H8), 8.25 (d, J = 6.5 Hz 1H, H3 or H8), 8.30 (s, 1H, H1 or H6), 10.02 (s, 1H, NH). FAB MS m/z: 258 (M+1–2HI, 10), 230 (M+1–HI and C2H5I, 19) 185 (2gly+1, 100). Anal. Calcd for C14H17I2N3S: C, 32.77; H, 3.34; N, 8.19. Found C, 32.56; H, 3.23; N, 8.01.
The filtrate was concentrated and purified by column chromatography (aluminum oxide, CHCl3-EtOH 5:1) to give 7-ethyl-2,7-diazaphenothiazine (10) (38 mg, 34%); an oil. 1H NMR (CDCl3) δ: 1.58 (t, J = 7.8 Hz, 3H, CH3), 4.21 (q, J = 7.8 Hz, 2H, CH2), 6.58 (d, J = 5.1 Hz, 1H, H4), 7.31 (s, 1H, H6), 7.45 (d, J = 6.8 Hz 1H, H9), 8.02 (d, J = 5.1 Hz, 1H, H3), 8.09 (d, J = 6.8 Hz 1H, H8), 8.32 (s, 1H, H1). FAB MS m/z: 230 (M+1, 100), 200 (M–C2H5, 14). Anal. Calcd for C12H11N3S: C, 62.86; H, 4.84; N, 18.33. Found C, 62.69; H, 4.72; N, 18.13.
Synthesis of 2,7-dibutyl-2,7-diazaphenothiazidiinium diiodide (7) and 7-butyl-2,7-diazapheno-thiazine (11)
To a stirred solution of 10H-2,7-diazaphenothiazine (1) (100 mg, 0.5 mmol) in dry EtOH (5 mL) at rt, butyl iodide (0.18 mL, 1.6 mmol) was added and the reaction mixture was refluxed for 24 h. The solvent was distilled off, the residue was dissolved in a mixture CHCl3-EtOH (1:1) and purified by column chromatography (aluminum oxide, CHCl3-EtOH 1:1) to give:
1. 2,7-Dibutyl-2,7-diazaphenothiazidiinium diiodide (7) (80 mg, 30%), mp 210-212 °C (EtOH). 1H NMR (DMSO-d6) δ: 0.89 (m, 6Η, 2CΗ3), 1.28 (m, 4H, 2CH2), 1.76 (m, 4H, 2CH2), 4.12 (t, 2H, J = 7.0 Hz, NCH2), 4.32 (t, 2H, J = 7.0 Hz, NCH2), 6.81 (d, 1H, J = 6.0 Hz, H4 or H9), 7.73 (d, 1H, J = 6.0 Hz, H4 or H9), 7.75 (s, 1H, H1 or H6), 8.25 (d, 1H, J = 6.0 Hz, H3 or H8), 8.38 (d, 1H, J = 6.0 Hz, H3 or H8), 8.39 (s, 1H, H1 or H6), 11.01 broad s, 1H, NH).
FAB MS m/z: 314 (M+1–2HI, 100), 258 (M+1–HI and C4H9I, 88). Anal. Calcd for C18H25I2N3S: C, 37.98; H, 4.43; N, 7.38. Found C, 37.75; H, 4.33; N, 7.29.
2. 7-Butyl-2,7-diazaphenothiazine (11) (20 mg, 29%), mp 62-63 °C (EtOH). 1H NMR (CDCl3) δ: δ: 0.97 (t, J = 6.8 Hz, 3H, CH3), 1.33 (m, 2H, CH2), 1.71 (m, 2H, CH2), 3.68 (t, J = 6.8 Hz, 2H, NCH2), 6.44 (d, J = 4.9 Hz, 1H, H4), 6.48 (d, J = 6.0 Hz, 1H, H9), 6.61 (s, 1H, H6), 7.13 (d, J = 4.9 Hz, 1H, H3), 7.85 (d, J = 6.0 Hz, 1H, H8), 7.98 (s, 1H, H1). EI MS m/z: 257 (M, 100), 201 (M–C4H8, 74). Anal. Calcd for C14H15N3S: C, 65.34; H, 5.87; N, 16.33. Found C, 65.13; H, 5.71; N, 16.16.
Synthesis of 2,7-dibenzyl-2,7-diazaphenothiazidiinium dichloride (8) and 7-benzyl-2,7-diazapheno-thiazine (12)
To a stirred solution of 10H-2,7-diazaphenothiazine (1) (100 mg, 0.5 mmol) in dry EtOH (5 mL) at rt, benzyl chloride (0.15 mL, 1.5 mmol) was added and the reaction mixture was refluxed for 48 h. After cooling the resulted solid was filtered off to give 2,7-dibenzyl-2,7-diazaphenothiazidiinium dichloride (8) (90 mg, 40%), mp 211-212 °C (EtOH). 1H NMR (DMSO-d6) δ: 5.32 (s, 2H, CH2), 5.56 (s, 2H, CH2), 6.87 (d, J = 5.0 Hz, 1H, H4 or H9), 7.42-7.45 (m, 10H, 2C6H5), 7.67 (d, J = 5.0 Hz, 1H, H4 or H9), 7.95 (s, 1H, H1 or H6), 8.32 (d, J = 5.0 Hz, 1H, H3 or H8), 8.45 (s, 1H, H1 or H6), 8.49 (d, J = 5.0 Hz, 1H, H3 or H8), 11.08 (s, 1H, NH). FAB MS m/z: 382 (M+1–2HCl, 68), 292 (M+1–HCl and C6H5CH2Cl, 42), 185 (2gly+1, 100). Anal. Calcd for C24H21Cl2N3S: C, 63.43; H, 4.66; N, 9.25. Found C, 63.22; H, 4.57; N, 9.02.
The filtrate was concentrated and purified by column chromatography (aluminum oxide, CHCl3-EtOH 10:1) to give 7-benzyl-2,7-diazaphenothiazine (12) (37 mg, 37%); 59-61 °C (EtOH). 1H NMR (CDCl3) δ: 5.28 (s, 2H, CH2), 6.58 (d, J = 5.0 Hz, 1H, H4), 7.22 (s, 1H, H6), 7.33 (m, 2H, C6H2), 7.45 (m, 2H, C6H3), 7.47 (d, J = 6.1 Hz, 1H, H9), 8.01 (m, 2H, H3 and H8), 8.35 (s, 1H, H1). FAB MS m/z: 292 (M+1, 100), 201 (M+1–CH2C6H5, 28). Anal. Calcd for C17H13N3S: C, 70.08; H, 4.50; N, 14.42. Found C, 69.88; H, 4.32; N, 14.23.
Synthesis of 7-alkyl-2,7-diazaphenothiazines (9) and (12) from 2,7-dialkyl-2,7-diazaphenothiazi-diinium dihalides (5) and (8)
To a solution of 2,7-dialkyl-2,7-diazaphenothiazidiinium dihalides (5) or (8) (0.2 mmol) in water (2 mL). 15% aqueous NaOH solution (0.1 mL) was added. The solution became dark red and turbid. After 0.5 h the solution was extracted with CHCl3 (3 x 3 mL). The extracts were dried with anhydrous sodium sulfate and evaporated in vaccuo. The obtained residue was purified by column chromatography (aluminum oxide, CHCl3-EtOH 10:1) to give 7-methyl-2,7-diazaphenothiazine (9) (30 mg, 68%) or 7-benzyl-2,7-diazaphenothiazine (12) (41 mg, 70%).
X-Ray analysis
Data for 2,7-diazaphenothiazinium salts (3), (4) and (5) were collected on a KappaApexII difractometer with graphite monochromated MoKα radiation (λ = 0.71073 Å). The structures were solved by direct methods (SHELXS-97)26 and refined by full-matrix least-squares minimization based on all unique F2 (SHELXL-97-2).27
A. The crystals of a 1:1 mixture of compounds (3) and (4) were grown from EtOH-H2O (5:1) solution. Crystal data: [C12H12N3S]+·I–·H2O, Mr = 375.23, monoclinic, a = 7.0775(2), b = 20.3626(8), c = 18.9590(7) Å, α = 95.111(2)°, space group P21/n, V = 2721.4(2) Å3, Z = 8, μ = 2.50 mm−1. 17319 reflections were collected which 5480 were independent and 4424 reflections with I > 2σ(I) (Rint = 0.099). the structure was refined to R[F2 > 2σ(F2)] = 0.066 and wR(F2) = 0.133. Absorption correction: multi-scan from symmetry-related measurements Sortav29 (Blessing 1995) (Tmin = 0.523, Tmax = 0.785).
B. The crystals of compound (5) were grown from EtOH-H2O (5:1) solution.
Crystal data: [C12H13N3S]2+·2I–·H2O, Mr = 503.13, monoclinic, a = 6.3362(3), b = 14.9233(6), c = 8.7638(4) Å, β = 98.784(2)°, space group P21/n, V = 818.96(6) Å3, Z = 2, μ = 3.96 mm−1. 6037 reflections were collected which 2377 were independent and 1982 reflections with I > 2σ(I) (Rint = 0.054). The structure was refined to R[F2 > 2σ(F2)] = 0.047 and wR(F2) = 0.092. Absorption correction: part of the refinement model (ΔF) SHELX97-227 I/σ threshold for reflections = 5.0 Δ(U)/λ2 = 0.0, highest even order spherical harmonic = 8, highest odd order spherical harmonic = 5 (Tmin = 0.315, Tmax = 0.749).
For both structures H-atoms were included in geometric positions and refined as ‘riding’ atoms (H-atoms of water molecules were located in a difference Fourier map of the electron density and included in the refinement, and their positional parameters were refined) with isotropic thermal parameters based upon the corresponding bonding carbon atom (Uiso = 1.5Ueq for CH3 and H2O and Uiso = 1.2Ueq for the aromatic carbons).
ACKNOWLEDGMENT
The work is supported by the Medical University of Silesia (grant KNW-1-008/10).
References
. #Part CXXII in the series of Azinyl Sulfides.
1. R. R. Gupta and M. Kumar, M. in Phenothiazines and 1,4-Benzothiazines – Chemical and Biological Aspects, ed. by R. R. Gupta, Elsevier, Amsterdam, 1988, pp. 1-161.
2. L. Amaral, M. Vivieros, and J. E. Kristiansen, Trop. Med. Int. Health, 2001, 6, 1016. CrossRef
3. L. Amaral and J. E. Kristiansen, Int. J. Antimicrob. Agents, 2001, 18, 411. CrossRef
4. D. Ordway, M. Viveiros, C. Leandro, R. Bettencourt, J. Almeida, M. Martins, J. E. Kristiansen, J. Molnar, and L. Amaral, Antimicrob. Agents Chemother., 2003, 47, 917. CrossRef
5. M. Viveiros, M. Martins, I. Couto, J. E. Kristiansen, J. Molnar, and L. Amaral, In vivo, 2005, 19, 733.
6. L. Amaral, M. Martins, and M. Viveiros, J. Antimicrob. Chemother., 2007, 59, 1237. CrossRef
7. N. Motohashi, M. Kawase, T. Kurihara, A. Hever, S. Nagy, I. Ocsocvszki, M. Tanaka, and J. Molnar, Anticancer Res., 1996, 16, 2525.
8. N. Motohashi, T. Kurihara, H. Sakagami, D. Szabo, K. Csuri, and J. Molnar, Anticancer Res., 1999, 19, 1859.
9. N. Motohashi, M. Kawase, S. Saito, and H. Sakagami, Curr. Drug Targets, 2000, 1, 237. CrossRef
10. N. Motohashi, M. Kawase, K. Satoh, and H. Sakagami, Curr. Drug Targets, 2006, 7, 1055. CrossRef
11. J. J. Aaron, M. D. Gaye Seye, S. Trajkovska, and N. Motohashi in Topics in Heterocyclic Chemistry, vol. 16, ed. by R. R. Gupta, Springer-Verlag, Berlin, 2009, pp 153-231.
12. K. Pluta, B. Morak-Młodawska, and M. Jeleń, J. Heterocycl. Chem., 2009, 46, 355. CrossRef
13. B. Morak-Młodawska and K. Pluta, Heterocycles, 2007, 71, 1347. CrossRef
14. B. Morak and K. Pluta, Heterocycles, 2009, 78, 1289. CrossRef
15. K. Pluta, M. Jeleń, B. Morak-Młodawska, M. Zimecki, J. Artym, and M. Kocięba – “Anticancer Activity of newly Synthesized Azaphenothiazines in NCI’s Anticancer Screening” – Pharmacol. Reports, 2010, 62, 319.
16. M. Zimecki, J. Artym, M. Kocięba, K. Pluta, B. Morak-Młodawska, and M. Jeleń, Cell. Mol. Biol. Lett., 2009, 14, 622. CrossRef
17. F. H. Clarke, G. B. Silverman, C. M. Watnick, and N. Sperber, J. Org. Chem., 1961, 26, 1126. CrossRef
18. E. Werle, E. Kopp, and G. Leysath, Arzneim.-Forsch., 1962, 4, 443.
19. G. Pappalardo, F. Vittorio, G. Ronsisvalle, and F. Duro, Ann. Chim., 1973, 63, 255.
20. S. Carter and G. Cheeseman, Tetrahedron, 1977, 33, 827. CrossRef
21. W. Saari, D. Cochran, Y. Lee, E. Cresson, J. Springer, M. Williams, J. Totaro, and G. Yarbrough, J. Med. Chem., 1983, 26, 564. CrossRef
22. E. Stahl, Thin-layer chromatography, Springer-Verlag, Berlin, 1969, p. 508.
23. Prediction of Activity Spectra for Substance, http://ibmc.msk.ru/PASS/.
24. A. Zięba and A. Maślankiewicz, Eur. J. Org. Chem., 2000, 2947. CrossRef
25. A. Zięba and K. Suwińska, Heterocycles, 2006, 68, 495. CrossRef
26. G. M. Sheldrick, SHELXS-97, Program for X-Ray Crystal Structure Solution, Goettingen University, Germany, 1997.
27. G. M. Sheldrick, SHELXL-97-2, Program for X-Ray Crystal Structure Refinement, Goettingen University, Germany, 1998.
28. M. N. Burnett and C. K. Johnson, Report ORNL-6895. Oak Ridge National Laboratory, Oak Ridge, Tennessee, 1996