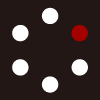
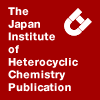
HETEROCYCLES
An International Journal for Reviews and Communications in Heterocyclic ChemistryWeb Edition ISSN: 1881-0942
Published online by The Japan Institute of Heterocyclic Chemistry
e-Journal
Full Text HTML
Received, 6th July, 2010, Accepted, 2nd August, 2010, Published online, 4th August, 2010.
DOI: 10.3987/COM-10-12009
■ New Nonadride Analogues from a Freshwater Isolate of an Undescribed Fungus Belonging to the Order Pleosporales
Tomoo Hosoe, James B. Gloer,* Donald T. Wicklow, Huzefa A. Raja, and Carol A. Shearer
Department of Chemistry, University of Iowa, Iowa City, IA 52242, U.S.A.
Abstract
Three new nonadride derivatives, tetrahydroepiheveadride (1), dideoxoepiheveadride (2) and deoxodihydroepiheveadride (3), and two new C-9 compounds, waquafranone A (7) and B (8), which were suggestive of precursors of nonadrides, along with other known compounds epiheveadride (4), deoxoepiheveadride (5), dihydroepiheveadride (6), sydonic acid (9), hydroxysydonic acid (10), folipastatin (11), and agonodepside B (12), from the newly described freshwater aquatic fungus Wicklowia aquatica. The chemical structures of 1 - 12 were elucidated by the spectroscopic and chemical investigation and, comparison to literature data.INTRODUCTION
During our ongoing studies of fungi from underexplored freshwater ecosystems as sources of new bioactive metabolites, a freshwater isolate of a previously undescribed fungus was subjected to chemical investigation. The isolate (strain F76-1) was obtained from herbaceous material collected from a backwater swamp in the Ocala National Forest, and appears to represent a new species belonging to the order Pleosporales (Dothideomycetes). Studies of an extract from fermentation of this isolate afforded three new antifungal nonadride analogues, along with the known nonadrides epiheveadride, dihydroepiheveadride, and deoxoepiheveadride. Two additional new C-9 compounds which were suggestive of precursors of the nonadrides were obtained from the extract. These compounds were isolated using column chromatography and HPLC, and their structures were elucidated by analysis of MS, 1D NMR, and 2D NMR data. Herein, we report new metabolites from a distinctive fungal isolate (F76-1) obtained from a freshwater ecosystem.
RESULTS AND DISCUSSION
Compounds 1-12 were obtained from the EtOAc extract of fermentation cultures of isolate (F76-1). Three of the compounds; epiheveadride (4), dihydroepiheveadride (5), and deoxoepiheveadride (6), were already known as antifungal substances originally reported from an unidentified fungus IFM52672 by Hosoe et al.1 The structure determination of three new analogues (1-3) described here was facilitated by the availability of these known metabolites. Two additional new C-9 compounds, waquafranone A (7) and B (8) were encountered as apparent monomer units that could be precursors to 1-6. Four other known compounds also obtained (9-12) were identified by comparison to literature data.2
The molecular formula of 1 was confirmed as C18H24O6 by HREI-MS spectrum. The 1H and 13C NMR spectra of 1 showed at broad peak and/or having a several minor peaks due to the existence of a mixture of stereoisomers associated with the presence of two hemiacetal units for which peaks can be observed around δ 6 ppm. A similar phenomenon was reported for 5, having a hemiacetal unit in its structure. Compound 1 was assumed to be a tetrahydro derivative of epiheveadride (4) on the basis of HREIMS data and 1D and 2D NMR analysis (Table 1 and Figure 2). Treatment of a sample of 1 with PCC afforded epiheveadride (4). The optical rotation of reactive product 4 was -162° (c 0.18, CHCl3), and that of a naturally-occurring 4 was -170.9° (c 1.11, CHCl3). 1 Thereby the structure of 1 was confirmed including absolute configuration at C-8 and C-9.
HRESIMS analysis of Compound 2 gave molecular ion at m/z 304 corresponding to the molecular formula C18H24O2, which was consistent with 1H and 13C NMR spectra. (seven unsaturations). Compound 2 was proposed to be a dideoxo derivative of epiheveadride (4) upon comparison of 1H, 13C NMR, and HREIMS data for 4 with those of deoxyepiheveadride (6). This proposal was independently confirmed by analysis of 2D NMR data for 2 (Figure 2). As expected, a single, relatively sharp set of signals could be observed in the 1H NMR spectrum due to the absence of hemiacetal units in the structure. The absolute configuration of 2 was presumed to be 8R, 9R by analogy to other epiheveadride analogues.
The molecular formula of 3 was confirmed as C18H24O5 by HREI-MS spectrum. Compound 3 was assumed to be a deoxodihydroepiheveadride analogue upon comparison of 1H NMR, 13C NMR, and HREIMS data to those of 6. The regiochemical positions of the methylene (δH 4.66 and 4.81) and methine (δH 5.93) units in the two γ-lactone rings of 3 were determined by analysis of HMBC data.(Figure 2) Like the other epiheveadride analogues, compound 3 is proposed to have the 8R, 9R configuration. Treatment of a sample of 3 with PCC afforded deoxoepiheveadride (6). The optical rotation of reactive product 6 was -121° (c 0.075, CHCl3), and that of a naturally-occurring 6 was -83.0° (c 1.04, CHCl3).1 Thereby the stereochemistry of 3 was determined to be the 8R, 9R configuration.
The molecular formula of waquafranone A (7) was confirmed as C9H12O3 by HREI-MS spectrum (four unsaturations). The structure of waquafranone A (7) was independently determined by analysis of HREIMS, 1D NMR, and 2D NMR data. The relative configuration of waquafranone A (7) was assigned by analysis of 1H-1H coupling constants and NOESY correlations. (Figure 3) The coupling constants observed between 2-H and 3-H (9.5Hz) indicated that these protons have a trans-relative orientation. NOESY correlations of 3-H with 5-H indicated that these protons are on the same face of the ring system. Other correlation of 2-H with 9-H3 were used to place these protons on the opposite face of the molecule, thereby establishing the relative configurations of waquafranone A (7).
HREIMS analysis of waquafranone B (8) gave molecular ion at m/z 166.0628 (calcd. 166.0630) corresponding to the molecular formula C9H10O3, which was consistent with 1H and 13C NMR spectra (five unsaturations). Waquafranone B (8) is closely related to 7, and planer structure of 7 was identified in a similar manner. The stereochemistry of olefinic double bond (2 to 5) was determined by NOESY correlations of 9-H (δ 5.62) with 6-H2 and 7-H2. Both 7 and 8 resemble subunits of epiheveadrides 1-6, supporting the proposed formation of the epiheveadrides via dimerization of two C-9 units.
Three new nonadride analogues (1-3) were isolated from the fermentation extract of a freshwater aquatic fungal isolate, along with the known nonadrides, epiheveadride (4), dihydroepiheveadride (5), and deoxoepiheveadride (6). Two additional new C-9 compounds (7 and 8) were also obtained from the extract. The biosynthesis of nonadride has been reported by Barton and Sutherland.3 They have mentioned that the dimerzation of C-9 unit was proposed to give glaucanic acid, which is a kind of nonadride. Therefore, these C-9 compounds (7 and 8) are suggestive of monomeric precursors of the nonadrides, which are presumed to arise biosynthetically via dimerization of C-9 units. Interestingly, the new analogues (1-3) were inactive in disk assays against Aspergillus flavus and Fusarium verticillioides, but the known compounds 4-6 did show activity (Table 2).
In previous paper,1 we reported that reduction of the carbonyl group at C-12 in nonadrides is more related to the intensity of the antifungal activity than stereochemical difference of side-chains at C-8 and C-9. The current result has newly suggested that the presence of the carbonyl group at C-11 is important for the intensity of the antifungal activity.
EXPERIMENTAL
General Experimental Procedures
Optical rotations were measured with a Rudolph Autopo III automatic polarimeter. UV measurements were performed with an Amersham Biosciences Ultrospec 2100 pro UV/Visble spectrophotometer. IR measurements employed a JASCO FT/IR-4100 instrument. 1H and 13C NMR spectra were recorded on either a Bruker AVANCE-300 or a Bruker DRX-400 spectrometer. NOESY data were recorded on the Bruker DRX-400. HMBC and HMQC experiments were performed using a Bruker AVANCE-600 spectrometer. HPLC was carried out using a Beckman system Gold instrument with a model 166 UV detector. Other general procedures and instrumentation have been described previously.4
Fungal material
The isolate (F76-1) was obtained from herbaceous material collected from a backwater swamp in the Ocala National Forest in northern Florida, and appears to represent a new species belonging to the order Pleosporales (Dothideomycetes). Most recently, this fungus was described as a new genus and species, Wicklowia aquatica.5
Extraction and Isolation
Fermentation was carried out in a single 500-mL Erlenmeyer flask containing 450 g (50 g × 9) of rice. Distilled H2O (450 mL) was added to the flask, and the contents were soaked overnight before autoclaving at 15 lb/in. for 30 min. The flask was cooled to room temperature, inoculated with 3.0 mL of spore inoculumn, and incubated for 21 days at 25°C. After incubation, the fermented rice substrate was mechanically fragmented and then extracted repeatedly with EtOAc. The combined EtOAc extracts were filtered and evaporated to give a yellow oil. The EtOAc extract (1.46 g) was partitioned between hexane and MeCN. The obtained MeCN fraction (0.82 g) was chromatographed using a Sephadex LH-20 column [solvent system: hexane/CH2Cl2 (1:4) 180 mL, CH2Cl2/acetone (3:2) 220 mL, (1:4) 200 mL, acetone 200 mL, MeOH 200 mL] to yield 16 fractions. Each fraction was chromatographed using a HPLC (MeCN/H2O, a liner gradient 20-100% over 40 min) and obtained deoxodihydroepiheveadride (2: 25 mg), epiheveadride (4: 5 mg), and dihydroepiheveadride (6: 10 mg) for fraction 2, tetrahydroepiheveadride (3: 31 mg), and deoxoepiheveadride (5: 44 mg) for fraction 7, dideoxoepiheveadride (1: 57 mg), and folipastatin (11: 0.5 mg) for fraction 9, sydonic acid (9: 5 mg), and agonodepside B (12: 1.8 mg) for fraction 10, waquafranone A (7: 6 mg) for fraction 11, hydroxysydonic acid (10: 2 mg), and waquafranone B (8: 1.4 mg) for fraction 12.
Tetrahydroepiheveadride (1) Colorless amorphous solid; [α] -35.2°(c 2.87, MeOH); HREIMS Found: 336.1576 (M+), Calcd: 336.1573 (for C18H24O6); UV λmax (MeOH) nm (log ε) 214 (4.01); IR νmax (KBr) 3397(OH), 2961, 2932, 2874, 1756(C=O), 1458 cm-1; The assignments of 1H- and 13C-NMR spectral signals are summarized in Table 1.
Dideoxoepiheveadride (2) Colorless amorphous solid; [α]25D -20.2°(c 1.24, CHCl3); HREIMS Found: 304.1670 (M+), Calcd: 304.1675 (for C18H24O4); UV λmax (MeOH) nm (log ε) 212 (4.28); IR νmax (KBr) 2961, 2932, 2872, 1751 (C=O), 1453 cm-1; The assignments of 1H- and 13C-NMR spectral signals are summarized in Table 1.
Deoxodihydroepiheveadride (3) Colorless amorphous solid; [α]25D -25.7°(c 1.56, CHCl3); HREIMS Found: 320.1612 (M+), Calcd: 320.1624 (for C18H24O5); UV λmax (MeOH) nm (log ε) 211 (4.26); IR νmax (KBr) 3388(OH), 2960, 2931, 2873, 1753(C=O), 1674, 1456 cm-1; The assignments of 1H and 13C NMR spectral signals are summarized in Table 1.
Waquafranone A (7) Colorless amorphous solid; HREIMS Found: 166.0628 (M+), Calcd: 16.0630 (for C9H10O3); UV λmax (MeOH) nm (log ε) 203 (3.17); IR νmax (KBr) 2976, 2937, 2880, 1718(C=O), 1640, 1463, 1431 cm-1; 1H-NMR (300 MHz, CDCl3): δ1.07 (3H, t, J = 7.4 Hz, 8-H3), 1.13 (3H, d, J = 7.2 Hz, 9-H3), 2.08 (2H, qd, J = 7.6, 6.4 Hz, 7-H2), 2.75 (1H, qd, J = 9.5, 7.4 Hz, 3-H), 3.13 (1H, t, J = 9.5 Hz, 2-H), 5.33 (1H, dd, J = 15.2, 9.4 Hz, 5-H), 5.74 (1H, dt, J = 15.2, 6.4 Hz, 6-H). 13C-NMR (100 MHz, CDCl3): δ 13.9 (q, C-8), 15.4 (q, C-9), 26.6 (t, C-7), 42.7 (d, C-3), 53.6 (d, C-2), 125.6 (d, C-5), 138.8 (d, C-6), 177.4 (s, C-1), 179.2 (s, C-4). HMBC (600 MHz, CDCl3): 2-H→C-1, 3, 4, 5, 6, 9; 3-H→C-1, 2, 4, 5, 9; 5-H→C-1, 2, 3, 7; 6-H→C-1, 2, 3, 7, 8; 7-H2→C-5, 6, 8; 8-H3→C-6, 7; 9-H3→C-2, 3, 4.
Waquafranone B (8) Colorless amorphous solid; HREIMS Found: 168.0786 (M+), Calcd: 168.0786 (for C9H12O3); [α]25D -116.6°(c 0.28, MeOH); UV λmax (MeOH) nm (log ε) 207 (3.99); IR νmax (KBr) 2965, 2934, 2874, 1685(C=O), 1624, 1438, 1302 cm-1; 1H-NMR (300 MHz, CDCl3): δ 6.97 (1H, t, J = 7.8 Hz, 5-H), 2.18 (2H, dd, J = 7.8, 7.6 Hz, 6-H2), 1.49 (2H, qd, J = 7.6, 7.4 Hz, 7-H2), 0.94 (3H, t, J = 7.4 Hz, 8-H3), 5.62 (1H, d, J = 1.7 Hz, 9-H), 6.45 (1H, d, J = 1.7 Hz, 9-H). 13C-NMR (100 MHz, CDCl3): δ 14.2 (q, C-8), 129.8 (q, C-9), 23.0 (t, C-7), 137.9 (s, C-3), 132.4 (s, C-2), 146.8 (d, C-5), 32.6 (t, C-6), 169.7 (s, C-1), 169.4 (s, C-4). HMBC (600 MHz, CDCl3): 5-H→C-1, 2, 3, 6, 7; 6-H2→C-2, 5, 7, 8; 7-H2→C-5, 6, 8; 8-H3→C-6, 7; 9-H2→C-2, 3, 4.
Oxidation of Tetrahydroepiheveadride (1) by PCC (Pyridinium chlorochromate)
The CH2Cl2 solution (3 mL) of tetrahydroepiheveadride (1) (15 mg) was added to a CH2Cl2 suspension (1 ml) of PCC (70 mg) and the mixture was refluxed for 1 h with stirring. The reaction mixture was filtered, and the filtrate was evaporated in vacuo. The residue obtained was chromatographed on silica gel (Kieselgel 60, Merck) with CH2Cl2, followed by recrystallization from Et2O to give epiheveadride (4) (4 mg), which was identical with naturally occurring epiheveadride (4) on basis of comparisons of the [α]20D, 1H-NMR, EIMS, mp, TLC, and HPLC behavior.
Oxidation of Tetrahydroepiheveadride (3) by PCC (Pyridinium chlorochromate)
The CH2Cl2 solution (1 mL) of tetrahydroepiheveadride (3) (11 mg) was added to a CH2Cl2 suspension (1 ml) of PCC (15 mg) and the mixture was stirred for 1.5 h at rt. The reaction mixture was filtered, and the filtrate was evaporated in vacuo. The residue obtained was chromatographed on silica gel (Kieselgel 60, Merck) with benzene/acetone (2:1) to give deoxoepiheveadride (6) (1.5 mg), which was identical with naturally occurring deoxoepiheveadride (6) on basis of comparisons of the [α]D, 1H-NMR, EIMS, and TLC behavior.
Antimicrobial property
Antifungal activity was determined using the agar diffusion method with paper disc (6 mm in diameter), for Aspergillus flavus NRRL6541 and Fusarium verticillioides NRRL25457 as described in the previous paper,4 loaded with 100 µg of compound on test plate.
ACKNOWLEDGEMENTS
Financial assistance for this research was provided by the National Science Foundation (CHE-0315591) and the National Institutes of Health (GM 60600). The authors also thank the staff of the University of Iowa High Field NMR and Mass Spectrometry facilities for their assistance.
References
1. T. Hosoe, K. Fukushima, T. Itabashi, K. Nozawa, K. Takizawa, K. Okada, G. M. C. Takaki, and K. Kawai, J. Antibiot., 2004, 57, 573; T. Hosoe, K. Fukushima, T. Itabashi, K. Nozawa, K. Takizawa, and K. Kawai, Heterocycles, 2004, 63, 2581. CrossRef
2. T. Hamasaki, K. Nagayama, and Y. Hatsuda, Agric. Biol. Chem., 1978, 42, 37; K.Hamano, M. Kinoshitra-Okami, A. Hemmi, A. Sato, M. Hisamoto, K. Matsuda, K. Yoda, H. Haruyama, T. Hosoya, and K. Tazawa, J. Antibiot., 1992, 45, 1195; S. Cao, A. S. Y. Lee, Y. Huang, H. Flotow, S. Ng, M. S. Butler, and A.D. Buss, J. Nat. Prod., 2002, 65, 1037. CrossRef
3. D. H. R. Barton, and J. K. Sutherland, J. Chem. Soc., 1965, 1769; CrossRef D. H. R. Barton, L. M. Jackman, and L. Rodriguez-Hahn, and J. K. Sutherland, J. Chem. Soc., 1965, 1772; CrossRef D. H. R. Barton, L. D. S. Godinho, and J. K. Sutherland, J. Chem. Soc., 1965, 1779; CrossRef J. E. Baldwin, D. H. R. Barton, J. L. Bloomer, L. M. Jackman, L. Rodriguez-Hahn, and J. K. Sutherland, Experentia, 1962, 18, 345; CrossRef J. K. Sutherland, Fortschr. Chem. Org. Naturst., 1967, 25, 131.
4. P. Jiao, D. C. Swenson, J. B. Gloer, J. Campbell, and C. A. Shearer, J. Nat. Prod., 2006, 69, 1667; CrossRef D. C. Swenson, J. B. Gloer, J. Campbell, and C. A. Shearer, Org. Lett., 2006, 8, 3191. CrossRef
5. H. A. Raja, A. Ferrer, C. A. Sheaser, and N. Miller, Mycoscience, 2010, 51, 208. CrossRef