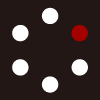
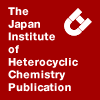
HETEROCYCLES
An International Journal for Reviews and Communications in Heterocyclic ChemistryWeb Edition ISSN: 1881-0942
Published online by The Japan Institute of Heterocyclic Chemistry
e-Journal
Full Text HTML
Received, 8th July, 2010, Accepted, 23rd August, 2010, Published online, 23rd August, 2010.
DOI: 10.3987/COM-10-12012
■ Triacylated Anthocyanidin 3-Arabinosylglucoside-7,3’-diglucosides Isolated from the Bluish Flowers of Tradescantia virginiana Cultivars and Their Distribution in the Tradescantieae
Fumi Tatsuzawa,* Norio Saito, Kazushi Maeyama, Masato Yokoi, Atsushi Shigihara, and Toshio Honda
Laboratoryof Olericultural and Floricultural Science, Faculty of Agriculture, Iwate University, 3-18-8 Ueda, Morioka, Iwate 020-8550, Japan
Abstract
Two triacylated anthocyanidin 3-arabinosylglucoside-7,3’-diglucosides were isolated from the purple-violet - violet-blue flowers of Tradescantia virginiana cultivars. The structures were determined to be 3-O-[6-O-(2-O-(trans-caffeoyl)-α-arabinofuranosyl)-β-glucopyranoside]-7,3’-O-di-[6-O-(trans-caffeoyl)-β-glucopyranoside]s of delphinidin and cyanidin. The former is a new anthocyanin in plants, whereas the latter, a rare anthocyanin has been recognized to be present in Zebrina pendula. Both anthocyanins exhibited typical three λmax in the visible region at 537, 574 and 620 nm for the delphinidin glycoside and at 510, 545 and 580 nm for the cyanidin glycoside in a 5.6 pH solution. These spectroscopic characteristics might be responsible for forming the intramolecular co-pigmentation between anthocyanidin and caffeic acid residues in these pigments. By HPLC analysis of nine species of the tribe Tradescantieae it was revealed that polyacylated anthocyanidin 3-arabinosylglucoside-7,3’-diglucosides were commonly found in all these plants as their major anthocyanins.INTRODUCTION
In the family of Commelinaceae, there are two subfamilies, such as the Cartonematoideae and Commelinoideae. The latter subfamily is further classified into two tribes, has divided into the tribe Commelineae and the tribe Tradescantieae.1 From the chemotaxonomic point of view of anthocyanin components in this family, two distinctive patterns have been recognized, such as anthocyanidin 3,5-diglucosides in the Commelineae and anthocyanidin 3,7,3’-glycosides in the Tradescantieae.2 Regarding the Tradescantieae, Yoshitama (1978) firstly reported the occurrence of cyanidin and delphinidin 3,7,3’-triglucosides acylated with caffeic and ferulic acids, in the flowers of Tradescantia reflexa.3 Stirton and Harbone (1980) also reported the presence of anthocyanidin 3,7,3’-glycosides by investigation of 28 species of 10 genera in this tribe. Thereafter, five polyacylated cyanidin 3-arabinosylglucoside-7,3’-diglucosides were found in this tribe; such as Zebrinin and monodecaffeylzebrinin from Zebrina pendula by Idaka et al. (1987),4 Tradescantin from T. pallida by Shi et al. (1992, 1993),5,6 and Baublis and Berber-Jimenez (1995),7 Rhoeonin from Rhoeo spathacea, and Setcreasin from Setcreasea purpurea by Idaka et al. (1987).8
In our continuing works on flower color variation due to acylated anthocyanins of the ornamental plants,9 we were interested in anthocyanin pigments of the blue-violet flowers of Tradescantia virginiana cultivars and decided to elucidate their anthocyanin structures, which were anticipated to be heavily acylated with a number of hydroxycinnamic acids.
In this paper, we wish to report the structure elucidation of triacylated delphinidin and cyanidin 3-arabinosylglucoside-7,3’-diglucosides, in which the former is a new anthocyanin, and the later is not new but a rare anthocyanin. For the sake of convenience, this new anthocyanin is named as “Virginianin”. Furthermore, the distribution of these polyacylated anthocyanins was surveyed for nine species in the Tradescantoideae.
RESULTS AND DISCUSSION
Four anthocyanin pigments (1 - 4) were extracted from the violet – violet-blue flowers of Tradescantia virginiana cultivars with 5% HOAc, and purified using Diaion HP-20 resin column chromatography (CC), preparative paper chromatography (PC), and preparative HPLC according to the procedure described previously.10 Chromatographic and spectroscopic properties of these pigments are indicated in Table 1 and EXPERIMENTAL. Upon acid hydrolysis, all four pigments 1 – 4 gave glucose, arabinose, and caffeic acid. Delphinidin was found in the hydrolysates of pigments 1 and 3, and cyanidin also was found in the hydrolysated of pigments 2 and 4. Moreover, ferulic acid was found in the hydrolysates of pigments 3 and 4. Upon alkaline hydrolysis, pigments 1 and 3 resulted the same delphinidin glycoside, and pigments 2 and 4 resulted in the same cyanidin glycoside as their deacyl anthocyanins. The cyanidin glycoside was identified as cyanidin 3-arabinosylglucoside-7,3’-diglucoside in comparison with the authentic cyanidin 3-arabinosylglucoside-7,3’-diglucoside, obtained from zebrinin on alkaline hydrolysis, by the analysis of TLC and HPLC (See in EXPERIMENTAL). On the other hand, the delphinidin glycoside was presumed to be delphinidin 3-arabinosylglucoside-7,3’-diglucoside by the analytical results (See in EXPERIMENTAL).
FAB mass measurement of pigments 1 - 4 gave their molecular ions [M]+ (m/z) at 1407 (pigment 1), 1391 (pigment 2), 1421 (pigment 3) and 1405 (pigment 4), respectively. Their chemical compositions based on FAB MS were determined as shown in Table 1. On the basis of above results, the structures of pigments 1 - 4 were presumed to be tricaffeoylcyanidin 3-arabinosylglucoside-7,3’-diglucoside as pigment 1, dicaffeoylmonoferuloylcyanidin 3-arabinosylglucoside-7,3’-diglucoside as pigment 3, tricaffeoyldelphinidin 3-arabinosylglucoside-7,3’-diglucoside as pigment 2, and dicaffeoylmonoferuloyldelphinidin 3-arabinosylglucoside-7,3’-diglucoside as pigment 4. The structures of pigments 1 and 2 were further elucidated based on the analyses on their 1H and 13C NMR spectra [500 MHz for 1H and 125.78 MHz for 13C spectra in CF3CO2D-CD3OD (1:9) or DCl-CD3OD (1:9), including 2D COSY, 2D NOESY, HMQC and HMBC spectra]. However, the further structural elucidations of pigments 3 and 4 could not be performed, unfortunately, due to its small amount available.
Pigment 1
The chemical shifts of 13 aromatic protons of delphinidin and caffeic acid moieties with their coupling constants were assigned by the analysis of 2D COSY spectrum of pigment 1 as shown in Table 2. The proton NMR spectrum of pigment 1 exhibited six olefin proton signals of caffeic acid moieties with large coupling constants (J=15.9, 15.6, and 15.9) suggesting to be trans configurations. The proton chemical shifts of the sugar moieties were observed in the region of δ 5.33 – 3.36, where the three anomeric protons of glucoses (A, B, and C) resonated at δ 5.33 (d, J =7.6 Hz, Glc B-H1), δ 5.08 (d, J =7.0 Hz, Glc C-H1), and δ 5.04 (d, J =7.6 Hz, Glc A-H1) (Table 2). Based on the observed coupling constants (Table 2), three glucoses were assumed to have β-pyranose form. Another anomeric proton of arabinose moiety was observed at δ 5.12 as a singlet. By the observed coupling constants (J 2,3=3.1 Hz, J 3,4=6.7 Hz, and J 4,5=11.9 Hz), the arabinose moiety was confirmed to have α-furanose form.
In the NOESY spectrum of pigment 1, strong NOEs were observed between H-4 of delphinidin and H-1 of Glc A, H-6 of delphinidin and H-1 of Glc B, H-2’ of delphinidin and H-1 of Glc C, and also H-6 of Glc A and H-1 of arabinose, indicating that delphinidin was glycosylated with Glc A at OH-3, Glc B at OH-7, and Glc C at OH-3’, respectively (Figure 1), and that the OH-6 of Glc A was glycosylated with arabinose to form vicianose [α-arabinosyl(1→6)glucose]. By the analysis of its 2D COSY spectrum, five characteristic proton signals shifted to lower magnetic fields were assigned to four methylene protons at δ 3.93 and 4.90 (Glc B, H-6a and H-6b), δ 3.96 and 5.10 (Glc C, H-6a and H-6b), and a methine proton at δ 5.14 (arabinose H-2). Thus, three hydroxyl groups of the three sugars, OH-6 of Glc B, OH-6 of Glc C and OH-2 of arabinose were determined to be acylated with caffeic acid, respectively. Therefore, pigment 1 was determined to be delphinidin 3-O-[6-O-(2-O-(trans-caffeoyl)-α-arabinofuranosyl)-β- glucopyranoside]-7,3’-O-di-[6-O-(trans-caffeoyl)-β-glucopyranoside], which is a new anthocyanin in plants.11,12 This structure was confirmed by the analysis of HMQC and HMBC spectra (Table 2 and Figure 1).
Pigment 2
The FAB mass spectrum gave a molecular ion [M]+ at 1391 m/z indicating that pigment 2 was composed to be cyanidin 3-arabinosylglucoside-7,3’-diglucoside with three molecules of caffeic acid. By the analysis TLC and HPLC, pigment 2 was identical with monodecaffeoylzebrinin4 in comparison with the authentic monodecaffeoylzeburinin obtained from leaves of Zebrina pendula (Table 1). This structure was confirmed by the analysis of its NMR spectra as follows. The 1H NMR spectrum of pigment 2 was identical with that of pigment 1 except for the signals of cyanidin moiety (Table 2). The resonances of the aromatic protons of cyanidin appeared at δ 8.40 (dd, J =2.1 and 8.9 Hz, H-6’), 8.36 (s, H-4), 7.46 (brs, H-2’), 7.01 (d, J =8.9 Hz, H-5’), 6.78 (d, J =2.1 Hz, H-6), and 6.69 (d, J =2.1 Hz, H-8), and also nine aromatic protons of caffeic acid moieties were assigned as shown in Table 2. The olefinic proton signals of three caffeic acids exhibited large coupling constants (J =15.9 Hz; caffeic acid I, J =15.9 Hz; caffeic acid II, and J =15.9 Hz; caffeic acid III), supporting the presence of trans configurations for three caffeic acids. The three anomeric protons of glucose moieties were assigned at δ 5.03 (d, J =7.7 Hz, Glc A), δ 5.36 (d, J =7.7 Hz, Glc B), and δ 5.09 (d, J =7.3 Hz, Glc C), and these glucose moieties were determined to be β-pyranose forms based on their observed J values. The anomeric protons of arabinose moiety were observed at δ 5.12 as a singlet. Since the vicinal coupling constants of arabinose moiety exhibited J=2.2 – 12.2 Hz, pigment 2 was supported to have α-arabinofuranose form. The linkages and positions between its anthocyanidin and sugars or sugars and acids were determined to be identical with those of pigment 1 by the analysis of its NOESY and COSY spectra (Table 2 and Figure 1). Therefore, the structure of pigment 2 was determined to be cyanidin 3-O-[6-O-(2-O-(trans-caffeoyl)-α- arabinofuranosyl)-β-glucopyranoside]-7,3’-O-di-[6-O-(trans-caffeoyl)-β-glucopyranoside], which has already been reported in the plant of Zebrina pendula.4 However, its distribution is very rare and this report is second one in plants.
The flower colors of T. virginiana cultivars
There are three different flower color types in the flowers of T. virginiana cultivars; such as a violet-blue flower (Violet-Blue 94A) of ‘True Blue’, a purple-violet flower (Purple-Violet 82C) of cultivar 2, and their intermediary color flowers of ‘Purple Dome’ (Violet 86B) and cultivar 1 (Violet 88B). By the analysis of HPLC, acylated delphinidin 3-arabinosylglucoside-7,3’-diglucosides were found in its violet-blue flower of ‘True Blue’ as dominant pigments, and acylated cyanidin 3-arabinosylglucoside-7,3’-diglucosides were found in the purple-violet flower of cultivar 2 as its major anthocyanins (Table 3). On the other hand, the intermediate color flowers of ‘Purple Dome’ and cultivar 1 contained both acylated 3-arabinosylglucoside-7,3’-diglucosides of cyanidin and delphinidin at considerable amounts (Table 3). On the light absorption responsible for flower colors, the absorption maxima of pigment 2 exhibit at 510, 542, and 580 nm in the pH 5.6 buffer solution, and are identical with those of the purple-violet flower of cultivar 2 (Figure 2-a). Moreover, the absorption maxima of pigment 1 observed at 537, 574, and 620 nm in the same pH 5.6 buffer solution, and are similar to those of the fresh flower of ‘True Blue’ (Figure 2-b). These absorption maxima of pigment 1 and 2 are comparable to the values of zebrinin (510, 542, and 583 nm) and cinerarin (537, 574, and 620 nm) in the buffer solution.9 Both these light absorption spectra of zebrinin and cinerarin are produced on basis of the intramolecular co-pigmentation between anthocyanidins and aromatic acid residues in their molecules.9 Therefore, it may be considered that these flower colors of T. virginiana cultivars are responsible for the intramolecular co-pigmentation of polyacylated anthocyanins between their anthocyanidins and aromatic acid residues.
The distribution of polyacylated anthocyanins in the plants of the Tradescantieae
Four polyacylated cyanidin 3-arabinosylglucoside-7,3’-diglucoside have been recognized in the Tradescantieae up to date, such as zebrinin and monodecaffeoylzebrinin from Zebrina pendula,4 tradescantin from the Tradescantia pallida,5-7 and rhoeonin from Rhoeo spathacea.8 In this paper, it was proved that anthocyanins of T. virginiana cultivars are constituted of polyacylated anthocyanins. Those anthocyanins can be classified into the group of acylated anthocyanidin 3-arabinosylglucoside-7,3’-diglucoside.
As mentioned above, Yoshitama (1987) reported the occurrence of acylated cyanidin and delphinidin 3,7,3’-triglucosides in the flowers of T. reflexa,3 and Stirton and Harborne (1980), also, reported that acylated cyanidin 3,7,3’-triglucosides were detected in 18 species of the tribe Tradescantieae.2 However, both reports became rather classical type of research on anthocyanins. Thus, we attempted to reinvestigated the detailed structures and the distributions in these plants.
As shown in Table 3, nine species in the Tradescantieae were investigated on their distributions of anthocyanin components by the analysis of HPLC, and the presence of nine acylated anthocyanins (pigments 1 – 9) were recognized in them. Pigments 1 – 4 were isolated from the flowers of T. virginiana cultivars and structures were elucidated in this study. Pigments 9, 8 and 7 were identified to be tradescantin, rhoeonin, and zebrinin in comparison with the authentic specimens isolated from T. pallida,7 Rhoeo spathacea,8 and Zebrina pendula.4 These pigments were further confirmed by the measurements of their FAB masses (Table 1). However, the structures of pigments 5 and 6 could not be elucidated due to their small amounts of available. From the results of these analyses, it was revealed that the distribution of acylated anthocyanidin 3-arabinosylglucoside-7,3’-diglucoside is very common in the plants of the Tradescantieae. Particularly, the occurrence of monodecaffeoylzebrinin is widely observed in this tribe. Thus, acylated anthocyanidin 3-arabinosylglucoside-7,3’-diglucosides were usefull to be a chemotaxonomic marker for the classification of plants in the Tradescantieae.
EXPERIMENTAL
General procedures
Thin layer chromatography (TLC) and paper chromatography (PC) were carried out on cellulose-coated plastic sheets (Merck) using eight mobile phases: BAW (n-BuOH/HOAc/H2O, 4:1:2, v/v/v), BuHCl (n-BuOH/2N HCl, 1:1, v/v, upper layer), AHW (HOAc/HCl/H2O, 15:3:82, v/v/v), 1% HCl, and Forestal (HOAc/HCl/H2O, 30:3:10, v/v/v) for anthocyanins, and BAW, APW (EtOAc/pyridine/H2O, 15:7:5, v/v/v), EFW (Et2O/HCO2H/H2O, 5:2:1, v/v/v), and 15% HOAc-H2O for sugars and organic acid with UV light and aniline hydrogen phthalate spray regent.13
Analytical HPLC was performed LC 10A system (Shimadzu) equipped with PDA-detector (Shimadzu SPD-M10Avp), using a Waters C18 (4.6 φ x 250 mm) column at 40 oC with a flow rate of 1 mL/min and monitoring at 530 nm. The eluant was applied as a linear gradient elution for 40 min from 20 to 85 % solvent B (1.5% H3PO4, 20% HOAc, 25% MeCN in H2O) in solvent A (1.5% H3PO4 in H2O). UV-Vis spectra of pigments were recorded on a MPS-2450 (Shimadzu) in 0.1% HCl-MeOH and pH5.6 buffer (from 360 to 700 nm). Spectral absorption of the flowers was directly measured on the intact petals using a recording spectrophotometer operated as double-beam instrument (Type MPS-2450 Shimadzu).13 FAB mass spectra were obtained in the positive ion mode using the magic bullet (5:1 mixture of dithiothreitol and dithioerythritol) as a matrix.
NMR spectra ware recorded at 500 MHz for 1H spectra and at 125.78 MHz for 13C spectra in CD3OD-DCl (9:1) and DMSO-d6-CF3CO2D (9:1). Chemical shifts are reported relative to a tetramethylsilane (TMS) internal standard (δ), and coupling constants (J) are in Hz.
Plant materials
Nursery plants of T. virginiana cultivars, T. sillamontana, and T. spathacea were purchased from Takii Co. Ltd (Kyoto), and Sakata Co. Ltd (Yokohama) and grown in greenhouses and fields at Iwate and Chiba Universities. The flowers of T. virginiana cultivars exhibited from purple-violet to violet-blue colors; cultivar ‘Purple Dome’ = Violet 86B by Royal Horticultural Society Color Chart and its intact petal λmax (510), 542, 580, (620) nm; ‘True Blue’ = Violet-Blue 94A and λmax 540, 578, 620 nm; cultivar 1 = Violet 88B and λmax (510), 541, 580, (623 nm); cultivar 2 = Purple-Violet 82C and λmax 510, 542, 580 nm. Flowers and leaves were collected in June and July in 2003 - 2009, dried overnight at 40oC, and kept refrigerator at 4oC until needed. Cyanotis kewensis, Dichorisandra thyrsiflora, Tradescantia fluminensis ‘Laeomensis’, T. paludosa, T. purpsii, T. pallida ‘Purple Heart’, T. zebrina (=Zebrina pendula) ‘Purpsii’ and ‘Quadricolor’, and T. spathacea (=Rhoeo spathacea) were kindly gotten from the Minami-Bousou Plant Garden (Minami-Bousou City, Chiba), and were confirmed by Dr. M. Yokoi.
Isolation and purification of anthocyanins
Pigments 1 – 4 from T. virginiana cultivars
Dried flower (ca. 200 g) of T. virginiana cultivars were immersed in 5% HOAc (5 L) for 5 h at rt and extracted. The extract was passed through a Diaion HP-20 resin (Mitsubishi Chemical’s Ion Exchange Resins) column (90 x 150 mm), on which anthocyanins were absorbed. The column was thoroughly washed with H2O (10 L) and eluted 5% HOAc-MeOH (1L) to recover anthocyanins. After concentration, the eluates were separated and purified with paper chromatography using BAW. The separated pigments 1 – 4 were further purified with preparative HPLC. Preparative HPLC was performed on a Waters C18 (19 x 150 mm) column at 40oC with a flow rate of 4 ml/min-1 and monitoring at 530 nm.
The solvent used was as follows; a linear gradient elution for 15 min from 50 to 70% solvent B (1.5% H3PO4, 20% HOAc, 25% MeCN in H2O) in solvent A (1.5% H3PO4 in H2O). Finally, four anthocyanins were obtained as follows; pigment 1 (ca. 150 mg), pigment 2 (ca. 60 mg), pigment 3 (ca. 5 mg), and pigment 4 (ca. 4 mg), respectively.
Pigments 1 – 4; Characterization of pigments 1 – 4 were carried out as follows. All anthocyanins are dark purple-violet powders; for UV-Vis, TLC, HPLC, and FAB-MS, see Table 1. Pigment 1; HR-FAB MS calc. 1407.3463 (C65H67O35). Found 1407.3485. Pigment 2; HR-FAB MS calc. 1391.3514 (C65H67O34). Found 1391.3516.
Authentic pigments
Tradescantin was obtained from T. pallida ‘Purple Heart’,7 zebrinin was obtained from T. zebrina (=Z. pendula),4 and rhoeonin was obtained from T. spathacea (=R. spathacea).8 The structures of these anthocyanins were confirmed by the analysis of chemical and spectroscopic methods (see Table 1).
Deacyl pigment 1 (delphinidin 3-arabinosylglucoside-7,3’-diglucoside); Dark purple-violet powder; UV-Vis λmax (PDA of HPLC): 274, 513 nm, Rf values of TLC (x 100): BAW 4, BuHCl 1, 1%HCl 18, AHW 48, HPLC: Rt (min): 5.5.
Deacyl pigment 2 (cyanidin 3-arabinosylglucoside-7,3’-diglucoside); Dark purple-violet powder; UV-Vis λmax (PDA of HPLC): 278, 502 nm, Rf values of TLC (x 100): BAW 10, BuHCl 2, 1%HCl 33, AHW 61, HPLC: Rt (min): 7.1.
3-4. Distribution survey of anthocyanins in the plants of Tradescantia and its relatives
Dried petal (ca. 50 mg) or fresh petal (ca. 0.5 g) and fresh leaves (ca. 0.5 g) were extracted with MAW (MeOH/HOAc/H2O, 4:1:5, v/v/v). Quantitative analysis of anthocyanins was performed by HPLC on a Waters C18 (4.6 x 250 mm) column at 40oC with a flow rate of 1 ml/min and monitoring at 530 nm. Solvent system was used: a linear gradient elution for 40 min from 20 to 85% solvent B in solvent A.
References
1. R. B. Faden and D.R. Hunt, Taxon, 1991, 40, 19. CrossRef
2. J. Z. Stirton and J.B. Harborne, Biochem. Syst. Ecol., 1980, 8, 285. CrossRef
3. K. Yoshitama, Bot. Mag. Tokyo, 1978, 91, 207. CrossRef
4. E. Idaka, Y. Ohashi, T. Ogawa, T. Kondo, and T. Goto, Tetrahedron Lett., 1987, 28, 1901. CrossRef
5. Z. Shi, M. Lin, and F. J. Francis, J. Food Sci., 1992, 57, 758. CrossRef
6. Z. Shi, H. Doun, and F. J. Francis, J. Food Sci., 1993, 58, 1068. CrossRef
7. A. J. Baublis and M. D. Berber-Jimenez, J. Agric Food Chem., 1995, 43, 640. CrossRef
8. E. Idaka, T. Ogawa, T. Kondo, and T. Goto, Agric. Biol. Chem., 1987, 51, 2215. CrossRef
9. T. Honda and N. Saito, Heterocycles, 2002, 56, 633. CrossRef
10. N. Saito, F. Tatsuzawa, E. Suenaga, K. Toki, K. Shinoda, A. Shigihara, and T. Honda, Phytochemistry, 2008, 69, 3139. CrossRef
11. J. B. Harborne and H. Baxter, “The Handbook of Natural Flavonoids”, Vol. 2, 1999, John Wiley & Sons, Chichester, (New York, Weinheim, Brisben, Shingapore, and Toronto).
12. Ø. M. Andersen and M. Jordheim, The anthocyanins. “Flavonoids: Chemistry, Biochemistry and Applications”, p. 471, ed. by Ø. M. Andersen and K. R. Markham, 2006, pp. 471-551. CRC Press, (Boca Raton, London, and New York).
13. J. B. Harborne, Phytochemical Methods, second ed., 1984, Chapman and Hall, (London). CrossRef
14. M. Yokoi and N. Saito, Phytochemistry, 1973, 12, 1783. CrossRef