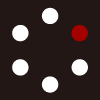
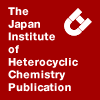
HETEROCYCLES
An International Journal for Reviews and Communications in Heterocyclic ChemistryWeb Edition ISSN: 1881-0942
Published online by The Japan Institute of Heterocyclic Chemistry
e-Journal
Full Text HTML
Received, 13th July, 2010, Accepted, 16th August, 2010, Published online, 16th August, 2010.
DOI: 10.3987/COM-10-12017
■ Rearrangement of 2-(2,5-Dioxopyrrolidin-1-yl)guanidine: An Efficient Synthesis and Structure of 3-(5-Amino-1H-1,2,4-triazol-3-yl)propanoic Acid and Derivatives
Victor M. Chernyshev,* Anna V. Chernysheva, and Zoya A. Starikova
Department of Chemistry, South-Russia State Technical University, Prosveschenya, 132, Novocherkassk 346428, Russia
Abstract
The reaction between aminoguanidine and succinic acid in water under acid catalysis yields a mixture of guanyl- and digyanylhydrazides of succinic acid, which turns into a poorly separable mixture of 3-(5-amino-1H-1,2,4-triazol-3-yl)propanoic acid (3) and 3,3'-(ethane-1,2-diyl)bis(1H-1,2,4-triazol-5-amine) (5) in basic media. The fusion of aminoguanidine hydrochloride with succinic anhydride at 150-170 °C results in the regioselective formation of 2-(2,5-dioxopyrrolidin-1-yl)guanidine hydrochloride (11). Compound 11 upon heating in an aqueous solution in the presence of alkali quantitatively rearranges into 3-(5-amino-1H-1,2,4-triazol-3-yl)propanoic acid (3). This reaction represents a new rearrangement in the 2,5-dioxopyrrolidine series. Investigations by pKa determination, IR, NMR and X-ray revealed that compound 3 exists both in unionized and zwitterionic forms.INTRODUCTION
Substituted azoles containing amino and carboxyl groups as substituents (so-called “azoleamino acids”)1 are valuable building blocks in medicinal chemistry. These compounds are used for the preparation of heteroaromatic oligopeptides, which are promising candidates for controlling gene expression2 and inhibiting protein-protein interactions.3 The best application in the 1,2,4-triazole series involves 5-amino-1H-1,2,4-triazole-3-carboxylic acid (1), which has been used for producing ribavirin and derivatives, which have anti-HCV and anti-HIV activities,4 antibiotics,5 water soluble azo dyes6 and metal-organic hybrid frameworks.7 Therefore, we hypothesize that homologues of compound 1, such as 2-(5-amino-1H-1,2,4-triazol-3-yl)acetic acid (2) and 3-(5-amino-1H-1,2,4-triazol-3-yl)propanoic acid (3), are also of great interest (Figure 1). The structural fragment of compound 2 is a constituent of semisynthetic cephalosporin antibiotics,5 and acyl derivatives of the ethyl ester of compound 2 exhibit anti-inflammatory activity.8
A general approach for the preparation of compounds 1-3 is the acylation of aminoguanidine by dicarboxylic acids in aqueous solutions and subsequent cyclization of the formed 2-guanylhydrazides in alkaline media.8,9 This method produces compound 1 from aminoguanidine and oxalic acid in high yields,9b but less favorable results are obtained with other dicarboxylic acids.10 For example, the reaction of aminoguanidine with malonic acid proceeds slowly and nonselectively relative to that with oxalic acid and gives a mixture of guanylhydrazide and diguanylhydrazide, which then form the desired compound 2 and by-product 4 upon alkaline cyclization (Figure 1).10 After kinetic and thermodynamic investigation of the reaction between aminoguanidine and malonic acid, we improved the synthetic procedure and enhanced the yield of compound 2 to 44%-51%.10 An analogous reaction of aminoguanidine with succinic acid has not been well studied. To the best of our knowledge, synthesis of compound 3 was described only in a patent,11 and the structural and chemical properties of this substance have not been investigated.
Herein, we report the effective synthesis and structure of compound 3 and some of its derivatives.
RESULTS AND DISCUSSION
Refluxing a mixture of aminoguanidine hydrogen carbonate and succinic acid in water for 8 h according to the described procedure11 yielded a white crystalline substance with a melting point (mp) of 183-185 °C (lit.11 184-185 °C). Elemental and spectral analyses as well as mp determination of a mixture with an authentic sample revealed that the obtained substance was the starting succinic acid rather than compound 3. Because of the absence of any spectral data in the cited patent,11 we assume that the structure of the desired product 3 was erroneously assigned to succinic acid. Iodatometric titration of the reaction mixture after synthesis according to the described procedure revealed that the conversion of aminoguanidine was only 2-4%, i.e., the reaction rate was very low. In accordance with kinetic investigations,10a the mechanism of an analogous reaction between aminoguanidine and malonic acid is similar to that of the acylation of amines by carboxylic acids via acid catalysis,12 but monoprotonated aminoguanidine (AG) is the nucleophile (Scheme 1).
The reaction rate is in direct proportion to the concentration of H3O+ and can be expressed by equation (1),10a
where k1 and k-1 are the rate constants of the forward and reverse reactions, respectively; cAG is the concentration of aminoguanidine cation (AG); cRCOOH is the concentration of the unionized form of carboxylic acid; and cGH is the concentration of 2-guanylhydrazide.
It seems that the reaction for the formation of succinic acid 2-guanylhydrazide follows the same mechanism. We previously determined that an acceptable reaction rate is reached at a pH ≤ 2.10b However, because succinic acid is a weak acid (pKa = 4.21),13 the pH of the aminoguanidine succinate solution, which forms at the dissolution of aminoguanidine hydrogen carbonate and succinic acid in water, is only 4.2-4.3 even with 15% excess succinic acid. Therefore, the insufficient acidity of the reaction mixture is responsible for the very low reaction rate and failure of the synthesis according to the described procedure.11
To accelerate the synthesis of succinic acid 2-guanylhydrazide, we used an approach analogous to that described in our previous work.10b Hydrochloric acid was employed as a catalyst and the reaction was carried out in highly concentrated solutions to enhance the equilibrium yield. Analysis of the reaction mixtures revealed that equilibrium was reached within 70-90 min and that 86-87% of aminoguanidine was converted. Similar to the reaction between aminoguanidine and malonic acid,10 a mixture of compounds 7 and 8 formed, with yields of 65% and 22%, respectively, according to HPLC analysis (Scheme 2).
A mixture containing ~ 69% of compound 3 (yield ~45%) and ~ 19% of compound 5 (yield ~20%) was obtained after treatment of the guanylhydrazides 7 and 8 solution with NaOH and subsequent acidification. It is preferable to isolate compounds 3 and 5 at pH 4-5, this interval is limited by the ionization constants of compound 3. We determined by potentiometric titration that compound 3 has a pKa of 5.19±0.04 for the elimination of a proton and pKa of 3.67±0.05 for the addition of a proton in water at 20 °C. Considerable losses of compounds 3 and 5 were observed during their separation by crystallization from water due to comparable solubility (Table 1). However, at pH ~7, acid 3 formed a readily soluble sodium salt, while compound 5 was non-ionizable and almost insoluble at 0-5 °C and thus was separated by filtration. Unfortunately, due to losses within separation and crystallization, the pure compounds 3 and 5 were obtained in yields of only 27-32% and 8-11%, respectively.
Because the reaction between aminoguanidine and succinic acid did not produce compound 3 in satisfactory yields, we needed a more selective method for preparation of compound 3.
For the selective synthesis of compound 3 from aminoguanidine, the guanylhydrazide 7 (or equivalent compound) must be a main product at the first stage. We hypothesized that it is possible in the reaction between aminoguanidine and succinic anhydride (SAH), which should be a more selective acylating agent than succinic acid. Successful synthesis of 2-guanylhydrazide of benzoic acid by fusion of aminoguanidine hydrochloride with benzoic anhydride has been described in the literature.9b Therefore, we investigated a reaction between aminoguanidine hydrochloride (AGH) and SAH in melt.
We established that the hydrochloride of 2-guanylhydrazide of succinic acid (7) is formed selectively upon fusion of the reagents at 110-130 °C (Scheme 3). Compound 7 crystallizes from a melt during its formation, preventing the development of a side reaction for the formation of compound 8. Our attempts to isolate pure compound 7 were unsuccessful, because of decomposition during recrystallization, instead yielding compound 8 and succinic acid according to the NMR 1H and HPLC analyses. However, free guanylhydrazide 9 was successfully obtained by neutralization of an aqueous solution of the reaction mixture with NaHCO3 at room temperature (Scheme 3). Picrate 10 was prepared by rapid interaction of compound 9 with picric acid.
After fusion of AGH with SAH at 150 °C and higher or by heating the reaction mixture obtained at 110-130 °C to 150-155 °C, a highly exothermic reaction began and hydrochloride of 2-(2,5-dioxopyrrolidin-1-yl)guanidine (11) was formed in high yields (Scheme 3). Notably, the observed reaction is different from the similar reaction of the amidrazones of benzoic and pyridinecarboxylic acids with anhydrides of maleic and cis-1,2-cyclohexanedicarboxylic acids, at which substituted carboxyalkyl-1,2,4-triazoles are formed.14a,b In our case, the cyclization of compound 7 into compound 3 does not occur because the guanidine fragment of compound 7 is protonated and its nucleophilicity is very low.
Compound 9 cyclizes rapidly under heating in an alkaline water solution to produce triazole 3 in quantitative yield. The cyclization also takes place by refluxing 3 in water in the absence of an alkali, according to the recently reported procedure;15 however, the reaction occurs very slowly and is accompanied by the formation of sparingly soluble unidentified compounds. The reaction proceeds slowly because of the low nucleophilicity of the protonated guanidine fragment of compound 9, whose predominant tautomer probably has a zwitterionic structure (Scheme 3). Sparingly soluble by-products can result from side reactions of oligomerization due to the presence of a reactive carboxylic group in compound 9.
Compound 11 is sufficiently stable in water. Free 2-(2,5-dioxopyrrolidin-1-yl)guanidine (12) is formed with the addition of an equimolar amount of NaHCO3 to an aqueous solution of 11. Both compounds 11 and 12 can be converted quantitatively into the triazole 3 by short-term heating in an alkaline water solution (Scheme 4).
The observed rearrangement follows the ANRORC mechanism. The pyrrolidine-2,5-dione ring opens when exposed to hydroxide ions to give guanylhydrazide 9, which then cyclizes into triazole 3 (Scheme 5). This mechanism was corroborated by guanylhydrazide 9 being obtained by refluxing of compound 12 for 1 h in water (Scheme 4). Apparently, the hydroxide ion acts as a nucleophile instead of a water molecule, even in the absence of alkali. Because compound 12 is a weak base (pKa = 5.75±0.03 at 20 °C), its water solution has a slightly alkaline pH (8-9), thus providing the necessary concentration of hydroxyl ions. If a water molecule served as a nucleophile, the splitting of the pyrrolidine-2,5-dione ring would occur faster upon refluxing of compound 11 instead of 12 due to the negative inductive effect of the protonated guanidine fragment. However, compound 11 is hydrolyzed slowly in boiling water.
The transformation of compound 12 into triazole 3 represents a new rearrangement in the series of 2,5-dioxopyrrolidine. According to the Babaev-Zefirov ring-bond-redistribution (RBR) graphs classification of heterocyclic ring transformations (Figure 2), this rearrangement relates to the relatively rare (a)(d)-class of the simple heterocyclic ring transformations and has a code 552-(a)(d).16 The majority of other rearrangements of five-membered heterocycles involving a side chain of three atoms have the code 552-(a)(a), i.e. fission of an old bond and formation of a new bond occur at one pivotal atom, instead of at two, as in the case of the observed rearrangement.16,17 This rearrangement is topologically similar to the rearrangement of the methyl ester of 2-(2-(2,5-dioxopyrrolidin-1-yl)phenyl)acetic acid into 3-(3-(methoxycarbonyl)-1H-indol-2-yl)propanoic acid under the action of bases,18 which has the same type, class and sort, but relates to another family.16
Thus, two approaches for the preparation of compound 3 from AGH and SAH, which differ in the first step, are possible. The first method implies the synthesis of guanylhydrazide 7 at 110-130 °C, while the second includes the formation of compound 11 at temperatures higher than 150 °C. At the second step of both approaches, the intermediate product 7 or 11 is readily converted into compound 3 by heating in an alkaline water solution and subsequent acidification. Therefore, both approaches can be used for one-pot synthesis, i.e., without isolation of intermediate compound 7 or 11.
As determined by iodatometric titration, the conversion of AGH at 115-120 °C increased to ~ 60% within 15-20 min. Then, the reaction mixture began to crystallize and the reaction proceeded slowly due to diffusion limitation, reaching 93% conversion of AGH within only 2 h. At 160-170 °С the reaction was completed within 10 min with 96-97% conversion of AGH. Thus, the second approach for the preparation of compound 3 (through the intermediate compound 11) is more advantageous because it requires less time and provides greater conversion of the starting compounds. According to HPLC analysis, a small amount of diguanylhydrazide 8, which subsequently cyclizes to give by-product 5, is still formed with an equimolar ratio of reagents in the first stage of the synthesis. However, the use of a small excess (20-30%) of SAH relative to AGH allows for almost complete suppression of by-product formation (Table 2) and for obtaining pure compound 3 in 85% to 90% yield after one recrystallization from water.
Because we needed esters of compound 3 to investigate the tautomerism, methyl (13a) and ethyl (13b) esters were obtained by esterification of compound 3 with alcohols in the presence of thionyl chloride according to the previously described procedure for the preparation of the esters of acids 1 and 2.19 However, due to very high solubility in water and polar organic solvents, compounds 13a,b could not be isolated from the reaction mixtures by common methods with reasonable yields. Therefore, the esters were isolated in the form of acylated derivatives 14a,b, which were sparingly soluble in cold water and easily crystallized. Compounds 13a,b were obtained by deacetylation of 14a,b with pyrrolidine in benzene (Scheme 6). The picrate of the methyl ester of 3-(5-amino-1H-1,2,4-triazol-3-yl)propanoic acid (15), which is required for investigation of the tautomerism of compound 3, was obtained by rapid mixing of solutions of compound 13a and picric acid in acetonitrile (Scheme 6).
The structures of the synthesized compounds were determined by elemental analysis, MS, IR, NMR spectra and finally corroborated by X-ray diffraction studies of compounds 3 and 11. Signal assignments in the NMR 13C spectra were performed based on proton-coupled 13C spectra and 1H-13C HMBC spectra.
Compound 3 can presumably exist in the tautomeric forms A-F (Figure 3). Imino forms are not considered because they are recognized as being thermodynamically unstable compared to the amino forms and have not been detected so far.20 The form F was also excluded, because according to the quantum chemical calculations for protonated 3-amino-1,2,4-triazole and its N-methyl derivatives, the tautomer analogous to the form F is 8-9 kcal/mol less stable than the tautomers corresponding to the D and E forms.21
NMR spectroscopy is one of the most informative methods for investigation of the tautomerism of C-amino-1,2,4-triazoles in solutions.15,22 The 1H and 13C NMR spectra of compound 3 did not show doubling of signals characteristic for 3(5)-substituted 5(3)amino-1,2,4-triazoles,15 which could be accounted for either by the coalescence of signals of different tautomers or the predominance of one tautomer and a very low concentration (or absence) of the other tautomers.22 Comparison of the 1H and 13C NMR spectra of compound 3 with the spectra of esters 13a,b, picrate 15 and literature data for tautomeric forms A-C1,15,21,23 and salts D,E1,21,24 (Figures 4 and 5) suggests that compound 3 in DMSO exists predominately in the A and B tautomeric forms, but with the prevalence of tautomer A. Other tautomers are absent or present in low concentrations insufficient to be detected by NMR.
In the presence of tautomers D or E (Figure 3) in significant concentrations, the signals of NH2 protons should be observed at lower field (as for picrate 15), and the signals of the triazole carbons would be at higher field (Figures 4 and 5). In tautomer C, the carbon connected to the carboxyethyl group should give a signal at a considerably higher field, such as in 3-amino-4,5-dimethyl-1,2,4-triazole and 3-amino-4-methyl-5-phenyl-1,2,4-triazole (151.3 and 152.1 ppm, respectively).23b The proton spectra of the esters 13a,b at 0.1 M concentration show two sets of signals corresponding to tautomers A and В (Figures 4 and 5). However, the 13C NMR spectra of these compounds at 0.4 M concentration exhibit only one set of signals, apparently due to coalescence. The ratio of the integral intensities of NH2 protons of tautomers A and B gives KT = [A]/[B] ~ 3.2 at 27 °C. The NH2 protons of compound 3 exhibit an average shift between the corresponding signals of tautomers A and B of esters 13a,b. In addition, the 13C NMR spectrum of compound 3 is very similar to that of esters 13a,b, in which coalescence of the signals of tautomers A and B is observed. Therefore, we concluded that compound 3 in DMSO exists predominately as both tautomers A and B. The averaging of the NMR chemical shifts of the tautomers arises from coalescence due to accelerated proton exchange (in comparison with esters 13a,b) catalyzed by carboxyl group. Taking over as model signals the chemical shifts of NH2 protons of tautomers A and B of compound 13a we calculated by interpolation22 KT = 3.3 for compound 3. This value is very close to the KT of the esters 13a,b.
To estimate the tautomeric equilibrium of compound 3 in water and detect the existence13 of zwitterionic forms, we compared its acid-base properties with those of ester 13b. By potentiometric titration of 13b with 0.1 M hydrochloric acid at 20 °C, we determined a pKa of 4.32±0.05, which is an approximately average value between the pKa obtained by titration of compound 3 with acid (3.67±0.05) and base (5.19±0.04). Therefore, we concluded that an equilibrium exists between the neutral (apparently predominately A) and zwitterionic (probably D) forms of compound 3 and determined a ratio, R = [zwitterion]/[neutral molecule] calculated from the Ebert formula,13 of 3.47.
The structure of 3 in the crystal was investigated by FTIR spectroscopy and X-ray analysis. The FTIR spectrum of the sample obtained by crystallization of compound 3 from water contains a very broad band of the OH stretching absorption in the region of 3300-2500 cm-1, which is typical for carboxylic acids.25 An intensive band of a C=O stretching at 1674 cm-1 indicates the presence of an unionized tautomer, most likely tautomer A, based on the literature data for other 3-substituted 5-amino-1,2,4-triazoles.1,15,20,23h The stretching vibrations of the zwitterionic tautomers D and E, which have ionized carboxyl groups, should be observed in the region of 1610-1550 cm-1.25 Although the spectrum shows an intensive band at 1553 cm-1, this band can also be assigned to the valence vibrations of C=N of the triazole ring.20c For example, the analogous band presents in the FTIR spectra of compounds 14a,b. Because it was difficult to draw conclusions about the presence or absence of the zwitterionic forms of compound 3 based on the FTIR spectrum, we conducted X-ray crystallography.
A single crystal for X-ray analysis was obtained by crystallization of compound 3 from DMF. In the crystal, compound 3 exists as a solvate with DMF with a composition of 1:1. For subsequent discussion of the structure, the crystallographic numbering system will be used (Figure 6). The carboxyl group of 3 is partially deprotonated and a proton is disordered between O1 and N4 (site occupancy factors are 0.72 and 0.28, correspondingly). These observations likely result because the crystal consists of 72% of neutral (tautomer A) and 28% of zwitterionic (tautomer D) forms of compound 3. The bond lengths and angles in the aminotriazole fragment are close to those reported for other 5-amino-1,2,4-triazoles.1b,15a,23g,h The triazole fragment of the molecule is planar, with maximal deviations of atoms from the mean-square plane not exceeding 0.011(8) Å. A planar amino group is almost coplanar to the triazole ring (dihedral angle between the NH2 and triazole planes is 4(2)°). Conjugation between the unshared electron pair of N5 and the π system of the triazole cycle leads to shortening of the C5—N5 bond (1.342(1) Å) relative to the standard length of a purely single Nsp2—Csp2 bond (1.43-1.45 Å).26 The C5—N1 and C5—N4 bonds have almost equal lengths of 1.336(1) and 1.337(1) Å, respectively. In DMF, one of the methyl group (C11) is disordered in two positions, which differ in rotation about the C11—N6 bond. In the crystal, the molecules of 3 are linked together by hydrogen bonds: N5—H5A···O2 [N ···O 2.937(1) Å, O···H 2.08(2) Å, N—H 0.89(2) Å, angle NHO 162(1)°], O1—H1o···N4 [O···N 2.574(1) Å, H···N 1.71(2) Å, O—H 0.86(2) Å, angle OHN 173(1)°] and N4—H4···O1 [H···O 1.78(8) Å, N—H 0.80(6) Å, angle NHO 173(3)°] (Figure 6). Molecules of DMF are hydrogen-bonded to 3 via N1—H1···O3 [N···O 2.802(1) Å, H···O 1.95(2) Å, N—H 0.86(2) Å, angle NHO 168(1)°] and N5—H5B···O3 [N···O 2.896(1) Å, H···O 2.07(2) Å, N—H 0.84(2) Å, angle NHO 170(1)°].
The 1H NMR spectrum of compound 9 shows the broadening of NH signals and the appearance of an additional very broad peak at 7.03 ppm, indicating the presence of at least two different tautomers in DMSO. The structure and purity of 9 were also confirmed by elemental analysis, which is in good agreement with the chemical formula proposed for the monohydrate, and by the 13C NMR spectrum, which exhibits only one set of coalesced signals. In addition, the 1H NMR spectrum of picrate 10 is analogous to spectra of other salts of 2-guanylhydrazides of carboxylic acids.27 The pKa, determined by the potentiometric titration of 9 with 0.1 M hydrochloric acid in water and corresponding to the addition of a proton, is 3.95±0.02 (at 27 °C), which is considerably lower than the corresponding pKa of 2-guanylhydrazide of 4-chlorobenzoic acid (7.85±0.04 at 24 °C). By contrast, the pKa, determined by the titration of 9 with KOH and corresponding to the elimination of a proton, is 9.23±0.03 (at 30 °C). These data indicate that compound 9 in water exists in the zwitterionic form. The FTIR spectrum of 9 also indicates the presence of an ionized carboxyl group showing bands at 1568 and 1591 cm-1. Based on these data, the zwitterionic structure in Scheme 5 was assigned to compound 9, though its structural features and tautomerism demand more thorough investigation.
The proposed structures of compounds 11 and 12 are in good agreement with spectral data and were confirmed unambiguously by X-ray crystal analysis of compound 11. A single crystal for X-ray investigation was obtained by crystallization of 11 from 80% ethanol.
The structure of compound 11 (Figure 7) is somewhat similar to that of 2-guanylhydrazides of carboxylic acids and aminoguanidinium salts.28 The pyrrolidine-2,5-dione ring and N2N3N4C5 fragment of the molecule are planar and nearly perpendicular to each other, with a dihedral angle of 81.95(4)°. The bonds C5—N3 and C5—N4 have lengths of 1.313(1) and 1.329(1) Å, respectively, close to the analogous bonds in the aminoguanidine cation, though C5—N2 bond is somewhat longer (1.355(1) Å instead of 1.325-1.341 Å).28 All nitrogen atoms have a trigonal pyramidal configuration (sums of valence angles are 358.6 at N(1), 353.9 at N(2), 356.6 at N(3) and 357.3 at N(4)). The bond lengths and angles in pyrrolidine-2,5-dione cycle are in agreement with those reported for similar compounds.29
The spectra of compounds 14a,b are analogous to the ones described for other 1-acyl-5-amino-1,2,4-triazoles.1a,8,23f
In conclusion, we described the selective one-pot synthesis of compound 3 by fusion of aminoguanidine hydrochloride with succinic anhydride and subsequent rearrangement of the produced hydrochloride of 2-(2,5-dioxopyrrolidin-1-yl)guanidine (11) in an aqueous alkaline solution. The rearrangement is new for the 2,5-dioxopyrrolidine series and is related to a relatively rare (a)(d)-class of simple heterocyclic ring transformations. Compound 3 exhibits tautomerism and exists as tautomers A, B and zwitterion D depending on the conditions. Further analysis is needed to elucidate the structural features of compound 9.
EXPERIMENTAL
General. The melting points were determined in open capillary tubes in a Thiele apparatus and are uncorrected. The IR spectra were recorded on a Varian Excalibur 3100 FT-IR spectrometer using a single reflection ATR system as a sampling accessory. 1H and 13C NMR spectra were recorded on a Bruker DRX 500 instrument at 500 MHz and 125 MHz, respectively, and a Bruker Avance 600 instrument at 600 MHz (150 MHz for 13C) or Varian Unity 300 at 300 MHz in DMSO-d6 using TMS as an internal standard. Mass spectra were recorded in the form of m/z (intensity relative to base 100) on a Finnigan MAT INCOS 50 instrument using electron impact ionization. Elemental analyses were determined with a Perkin-Elmer 2400 Elemental Analyzer. HPLC analyses were performed on a Milichrom-5 chromatograph equipped with a Separon-C18 column (0.025 M solution of KH2PO4 as the mobile phase) and a UV detector (220 nm) according to a described procedure.10a The concentration of aminoguanidine in solutions was analyzed by iodatometric titration.10a The pKa values of compounds 3, 9, 12 and 13b were determined by potentiometric titration13 in water using a Mettler Toledo S40-KS instrument equipped with an InLab®Expert Pro combined electrode.
Single-crystal X-ray diffraction experiments were carried out with a Bruker SMART 1000 CCD area detector (for compound 3) and Bruker APEX II CCD area detector (for compound 11) using graphite monochromated Mo Kα radiation at 120 and 100 K, respectively. The structures were solved by the direct method and refined by a full-matrix least-squares method against F2 in anisotropic (for no-hydrogen atoms) approximation. For compound 3, all H(N) and H(O) hydrogen atoms were located from the difference Fourier syntheses and were refined in isotropic approximation. The H(C) atoms were placed in geometrically calculated positions and were refined in isotropic approximation in riding model with the Uiso(H) parameters equal to 1.2 Ueq(Ci), where U(Ci) are the equivalent thermal parameters of the atoms to which corresponding H atoms are bonded. For compound 11, the H(N) hydrogen atoms were located from the difference Fourier syntheses, and the H(C) atoms were placed in geometrically calculated positions. All hydrogen atoms were refined in isotropic approximation in a riding model with the Uiso(H) parameters equal to 1.2 Ueq(Ci), where U(Ci) are the equivalent thermal parameters of the atoms to which the corresponding H atoms are bonded. All calculations were performed on an IBM PC/AT using SHELXTL (G. M. Sheldrick, SHELXTL-97, Version 5.10, Bruker AXS Inc., Madison, WI-53719, USA). Atomic coordinates, bond lengths, bond angles and thermal parameters have been deposited at the Cambridge Crystallographic Data Centre (CCDC), with deposition numbers 783902 and 783901 for (3) and (11), respectively.
3-(5-Amino-1H-1,2,4-triazol-3-yl)propanoic acid (3) and 3,3'-(ethane-1,2-diyl)bis(1H-1,2,4-triazol-5-amine) (5). A mixture of succinic acid (4.31 g, 37 mmol), aminoguanidine hydrogen carbonate (5.00 g, 37 mmol) and 36% aqueous solution of hydrochloric acid (4.31 g, 43 mmol) was heated at 97 °C for 1.5 h. Then 30 mL (183 mmol) of 6.1 molar aqueous solution of NaOH was added and the reaction mixture was additionally heated at 97 °C for 1 h, acidified to pH 4-5 with conc. HCl, cooled to 3-5 °C and stored at this temperature for one day. The precipitate formed was filtered off and dried at 120 °C to give 3.77 g of a mixture, which, according to HPLC, contained 69% of compound 3 (45% yield) and 19% of compound 5 (20% yield). The mixture obtained was slurred in water (7 mL) and alkalified to pH 7-8 with 20% aqueous solution of NaOH. The slurry was heated at stirring to boiling and then cooled to 3-5 °C and kept at this temperature for one day. A precipitate formed was filtered off and dried to give 0.68 g (19% yield) of crude compound 5 as white solid. After one recrystallization from water the yield of pure compound 5 amounted to 0.4 g (11%), mp 310 °C (mp 310-312 °C30); 1H NMR, δ: 2.68 (br s, 4H, 2CH2), 5.00 (br s, 4H, 2NH2 of tautomer B), 5.77 (br s, 4H, 2NH2 of tautomer A), 11.54 (br s, 2H, 2NH of tautomer A), 12.32 (br s, 2H, 2NH of tautomer B); MS, m/z: 194 (M+, 72), 136 (12), 111 (20), 98 (100), 69 (10), 57 (27), 43 (86). Anal. Calcd for C6H10N8: C, 37.11; H, 5.19; N, 57.70. Found: C, 37.00; H, 5.31; N, 58.01.
The filtrate after separation of compound 5 was acidified with 20% HCl to pH 4.4-4.7 and cooled to 3-5 °C. A precipitate formed was filtered off to give 2.1 g (36% yield) of crude compound 3 as white solid. After one recrystallization from water the yield of pure compound 3 amounted to 1.56 g (27%), mp 219-220 °C; 1H NMR, δ: 2.54 (t, J = 7.4 Hz, 2H, CH2COOH), 2.62 (t, J = 7.4 Hz, 2H, CH2), 5.60 (s, 2H, NH2), 11.89 (br s, 2H, NH and COOH); 13C NMR, δ: 23.01 (CH2CH2COOH), 31.82 (CH2CH2COOH), 158.05 (С3 of triazole), 158.36 (C5 of triazole), 174.10 (COOH); IR (ATR), ν (cm-1): 3224, 3107, 2933, 2730, 2500, 1674 (CO), 1553, 1407; MS, m/z: 156 (M+, 23), 111 (100), 69 (10), 57 (40), 43 (48). Anal. Calcd for C5H8N4O2: C, 38.46; H, 5.16; N, 35.88. Found: C, 38.50; H, 5.18; N, 35.69.
Selective one-pot synthesis of 3-(5-amino-1H-1,2,4-triazol-3-yl)propanoic acid (3). A magnetically stirred mixture of finely ground aminoguanidine hydrochloride (30 g, 0.271 mol) and succinic anhydride (30 g, 0.300 mol) was gradually heated in an oil bath at stirring. The mixture melted at 95-100 °C and an exothermal reaction began at about 150-155 °C. At this point temperature of the reaction mixture rose to 160-170 °C and the melt solidified. The mixture was heated at 160-170 °C for 10 min and then cooled to 40-50 °C. A solution of NaOH (32 g, 0.8 mol) in water (80 mL) was added gradually at stirring. The resulted mixture was heated at 95-100 °C and stirring to complete homogenization and then additionally for 30 min, then cooled to 50-60 °C and acidified with 20% HCl to pH 4.4-4.7, causing precipitation of compound 3. The resulted slurry was cooled to 3-5 °C and kept at this temperature for 1 h. The precipitate formed was filtered off, washed with cold water and dried in vacuum at 120 °C to give 43.6-44.5 g of white solid, which contained, according to HPLC, 95-97% of compound 3 (yield ~ 100%). This product was crystallized from water to give 36.5-37.2 g (86-88% yield) of pure compound 3. Its mp, MS and 1H NMR spectra were identical with those prepared by the method, described above.
4-[2-(Diaminomethylidene)hydrazinyl]-4-oxobutanoic acid hydrate (9).
Method A. A mixture of finely ground aminoguanidine hydrochloride (6.00 g, 54.3 mmol) and succinic anhydride (5.43 g, 54.3 mmol) was heated in an oil bath at 120-130 °C and stirring for 20 min, then cooled to 20 °C and dissolved in 20 mL of water. Sodium hydrogen carbonate (4.56 g, 54.3 mmol) was added in a few portions to the resulted solution at stirring. The mixture was stirred for 20 min at 20 °C then cooled to 5 °C. The precipitate formed was filtered off, washed with ice-cold water and dried at 100 °C to give 5.96 g (57% yield) of title compound. White solid (from water), mp 181 °C (dec.); 1H NMR, δ: 2.08-2.11 (m, 2H, CH2), 2.31 (m, 2H, CH2), 7.03 (br s, 1H, NH), 7.67 (br s, 1H, NH), 9.31 (br s, 1H, NH), 9.98 (br s, 1H, NH), 10.47 (br s, 1H, NH); 13C NMR, δ: 29.42 (CH2), 32.45 (CH2), 159.52, 173.25 (CO), 177.17 (CO); IR (ATR), ν (cm-1): 3465, 3324, 3125, 2935, 2623, 1693, 1656, 1591, 1568, 1497; MS, m/z: 156 ([C5H10N4O3 – H2O]+, 20), 111 (20), 55 (22), 43 (100). Anal. Calcd for C5H12N4O4: C, 31.25; H, 6.29; N, 29.15. Found: C, 31.30; H, 6.39; N, 29.38.
Method B. A solution of compound 12 (1.00 g, 6.4 mmol) in 6 mL of water was refluxed for 1 h, then cooled to 5 °C. The precipitate formed was filtered off, washed sequentially with cold water and EtOH, then dried at 100 °C to give 0.84 g (68% yield) of title compound. Its mp, 1H NMR and IR spectra were identical with those prepared by method A.
4-[2-(Diaminomethylidene)hydrazinyl]-4-oxobutanoic acid picrate (10). A warm solution of picric acid (474 mg, 2.06 mmol) in EtOH (2 mL) was added at stirring to a warm solution of compound 9 (300 mg, 1.72 mmol) in water (1 mL). After cooling to room temperature, the precipitate formed was filtered off, washed with acetonitrile and dried in vacuum at 120 °C to give 395 mg (57% yield) of title compound. Yellow filaments, mp 180 °C (dec.); 1H NMR, δ: 2.41-2.43 (m, 2H, CH2), 2.47-2.49 (m, 2H, CH2), 7.39 (br s, 4H, 2NH2), 8.58 (s, 2H, arom.), 9.44 (s, 1H, NH), 10.04 (s, 1H, NH), 12.25 (s, 1H, OH); 13C NMR, δ: 27.16 (CH2), 28.64 (CH2), 124.57 (C4 of PkO-), 125.33 (C3 of PkO-), 141.82 (C2 of PkO-), 158.49, 160.95 (C1 of PkO-), 171.85 (CO), 173.88 (CO); IR (ATR), ν (cm-1): 3367, 3305, 3221, 3117, 1720, 1693, 1674, 1611, 1565, 1548, 1519. Anal. Calcd for C11H13N7O10: C, 32.76; H, 3.25; N, 24.31. Found: C, 32.40; H, 3.39; N, 24.68.
2-(2,5-Dioxopyrrolidin-1-yl)guanidine hydrochloride (11). A mixture of finely ground aminoguanidine hydrochloride (10 g, 90.5 mmol) and succinic anhydride (10 g, 100.0 mmol) was gradually heated in an oil bath at stirring. The mixture melted at 95-100 °C and an exothermal reaction began at about 150-155 °C. At this point temperature of the reaction mixture rose to 160-170 °C and the melt solidified. The mixture was heated at 160-170 °C for 30 min, cooled to room temperature and then dissolved in 15 mL of hot water. The solution obtained was diluted with EtOH (40 mL) and cooled to 3-5 °C. The precipitate formed was filtered off, recrystallized from water-EtOH mixture and dried at 120 °C to give a white solid (15.2 g, 87%). Mp 270-272 °C; 1H NMR, δ: 2.70 (s, 4H, 2CH2), 7.89 (br s, 2H, NH2), 8.09 (br s, 2H, NH2), 10.06 (br s, 1H, NH); 13C NMR, δ: 27.14 (2CH2), 157.97, 174.65 (2CO); IR (ATR), ν (cm-1): 3409, 3325, 3107, 2949, 1735, 1677, 1617, 1586; MS, m/z: 156 (C5H8N4O2+, 47), 114 (48), 101 (16), 85 (22), 55 (25), 43 (100). Anal. Calcd for C5H9ClN4O2: C, 31.18; H, 4.71; N, 18.41. Found: C, 31.16; H, 4.28; N, 18.55.
2-(2,5-Dioxopyrrolidin-1-yl)guanidine hydrate (12). Sodium hydrocarbonate (4.38 g, 52.1 mmol) was added gradually to a solution of compound 11 (10.00 g, 52.1 mmol) in 15 mL of water at 30-40 °C and stirring. Resulted mixture was cooled to 15-20 °C and stirred at this temperature for 20 min. The precipitate formed was filtered off, washed with cold water (10 mL) and dried at 100 °C to give 10.34 g (85% yield) of compound 12, pure enough for further synthesis. Analytical sample was obtained by crystallization of crude 12 from water. White plates, mp > 300 °C; 1H NMR, δ: 2.50 (s, 4H, 2CH2), 5.23 (s, 2H, NH2), 5.57 (s, 2H, NH2); 13C NMR: δ 26.90 (2CH2), 160.03, 175.64 (2CO); IR (ATR), ν (cm-1): 3433, 3344, 3167, 1683, 1667, 1644, 1569, 1498; MS, m/z: 156 (M+, 76), 114 (82), 101 (23), 85 (29), 43 (100). Anal. Calcd for C5H10N4O3: C, 34.48; H, 5.79; N, 32.17;. Found: C, 34.65; H, 5.55; N, 32.33.
Methyl 3-(1-acetyl-5-amino-1H-1,2,4-triazol-3-yl)propanoate (14a). Thionyl chloride (5.59 g, 47 mmol) was added drop by drop within 10 min to a stirred suspension of compound 3 (7.34 g, 47 mmol) in 25 mL of absolute MeOH. The resulted mixture was refluxed for 1 h. Then 5.59 g (47 mmol) of thionyl chloride was additionally added drop by drop and the reaction mixture was refluxed for 3 h. The excess of MeOH was distilled off in vacuum. The semisolid residue was cooled to 3-5 °C and neutralized to pH 5-6 by addition of cold saturated aqueous solution of sodium acetate at stirring. Then acetic anhydride 5.8 g (57 mmol) was added drop by drop to the neutralized solution and the reaction mixture was stirred for 20 min at 10-15 °C. The precipitate formed was filtered off, washed with cold water and dried to give 6.69 g (67% yield) of white solid. Mp 157-158 °C (from MeOH); 1H NMR, δ: 2.48 (s, 3H, CH3CO), 2.65-2.68 (m, 2H, CH2COOMe), 2.70-2.74 (m, 2H, CH2), 3.60 (s, 3H, CH3O), 7.43 (s, 2H, NH2); 13C NMR, δ: 23.04 (CH3CO), 23.30 (CH2), 30.58 (CH2), 51.42 (MeO), 157.07 (C5 of triazole), 161.65 (C3 of triazole), 171.14 (CH3CO), 172.48 (COOMe); IR (ATR), ν (cm-1): 3395, 3303, 3211, 3140, 2940, 1737 (CO), 1715 (CO), 1653, 1553; MS, m/z: 212 (M+, 11), 170 (47), 139 (23), 111 (100), 43 (45). Anal. Calcd for C8H12N4O3: C, 45.28; H, 5.70; N, 26.40. Found: C, 45.18; H, 6.00; N, 26.22.
Ethyl 3-(1-acetyl-5-amino-1H-1,2,4-triazol-3-yl)propanoate (14b). This compound was prepared analogously to compound 13a, yield 8.29 g (78% yield), white solid. Mp 130-131 °C (from water); 1H NMR, δ: 1.16 (t, J = 7.1 Hz, 3H, CH3CH2O), 2.47 (s, 3H, CH3CO), 2.65 (m, 2H, CH2), 2.71 (m, 2H, CH2), 4.07 (q, J = 7.1 Hz, 2H, CH3CH2O), 7.39 (s, 2H, NH2); 13C NMR, δ: 14.11 (CH3CH2O), 22.99 (CH3CO), 23.35 (CH2), 30.81 (CH2), 59.91 (CH3CH2O), 157.07 (C5 of triazole), 161.71 (C3 of triazole), 171.13 (CH3CO), 171.96 (COOEt); IR (ATR), ν (cm-1): 3391, 3301, 3211, 3142, 2960, 2940, 1737 (CO), 1718 (CO), 1652, 1550; MS, m/z: 226 (M+, 13), 184 (55), 139 (45), 111 (100), 54 (17), 43 (99). Anal. Calcd for C9H14N4O3: C, 47.78; H, 6.24; N, 24.77. Found: C, 48.01; H, 6.02; N, 24.51.
Methyl 3-(5-amino-1H-1,2,4-triazol-3-yl)propanoate (13a). Pyrrolidine (0.40 g, 5.6 mmol) was added to a suspension of compound 14a (1.00 g, 4.7 mmol) in benzene (3 mL) and the resulted mixture was refluxed for 30 min. After cooling to room temperature the precipitate formed was filtered off, crystallized from MeCN and dried. Yield 0.68 g (85%), white solid, mp 137-138 °C (from MeCN). 1H NMR, δ: 2.61-2.63 (m, 2H, CH2COOMe), 2.66-2.68 (m, 2H, CH2), 3.56 (s, 3H, CH3O), 5.02 (s, 2H, NH2 of tautomer B), 5.78 (s, 2H, NH2 of tautomer A), 11.56 and 12.30 (both br s, 1H, NH of tautomers A and B, accordingly); 13C NMR, δ: 22.97 (CH2CH2COOMe), 31.30 (CH2CH2COOMe), 51.27 (CH3O), 157.59 (C3 and C5 of triazole), 172.68 (CO); IR (ATR), ν (cm-1): 3383, 3108, 2956, 2930, 1722 (CO), 1659, 1591, 1533; MS, m/z: 170 (M+, 21), 139 (16), 111 (100), 57 (12), 43 (13). Anal. Calcd for C6H10N4O2: C, 42.35; H, 5.92; N, 32.92. Found: C, 42.56; H, 6.08; N, 32.76.
Ethyl 3-(5-amino-1H-1,2,4-triazol-3-yl)propanoate (13b). This compound was prepared from 14b analogously to compound 13a. Yield 0.71 g (87%), white solid, mp 104-105 °C (from EtOAc); 1H NMR, δ: 1.15 (t, J = 7.1 Hz, 3H, CH3CH2O), 2.59-2.66 (m, 4H, 2CH2), 4.03 (q, J = 7.1 Hz, 2H, CH3CH2O), 5.01 (s, 2H, NH2 of tautomer B), 5.78 (s, 2H, NH2 of tautomer A), 11.55 and 12.30 (both br s, 1H, NH of tautomers A and B, accordingly); 13C NMR: δ 14.01 (CH3CH2O), 23.03 (CH2CH2COOEt), 31.52 (CH2CH2COOEt), 59.74 (CH3CH2O), 156.96 and 158.96 (carbons of triazole), 172.13 (CO); IR (ATR), ν (cm-1): 3387, 3310, 3150, 2987, 2920, 1719 (CO), 1655, 1585, 1532; MS, m/z: 184 (M+, 21), 139 (31), 111 (100), 78 (10), 57 (14), 43 (21). Anal. Calcd for C7H12N4O2: C, 45.65; H, 6.57; N, 30.42. Found: C, 45.71; H, 6.42; N, 30.57.
Picrate of methyl 3-(5-amino-1H-1,2,4-triazol-3-yl)propanoate (15). A solution of 444 mg (1.94 mmol) of picric acid in MeCN (2 mL) was added at stirring to a hot solution of compound 13a (300 mg, 1.76 mmol) in MeCN (2 mL). The resulted mixture was cooled to 20 °C and precipitate formed was filtered off, washed with cold MeCN and dried at 100 °C. Yield 569 mg (71%), yellow solid, mp 177-178 °C (from MeCN); 1H NMR, δ: 2.71 (t, J = 6.8 Hz, 2H, CH2), 2.84 (t, J = 6.8 Hz, 2H, CH2), 3.59 (s, 3H, CH3O), 7.99 (s, 2H, NH2), 8.58 (s, 2H, arom.), 13.26 (br s, 2H, 2NH); 13C NMR: δ 20.60 (CH2), 29.59 (CH2), 51.60 (OCH3), 124.52 (C4 of PkO-), 125.31 (C3 of PkO-), 141.83 (C2 of PkO-), 150.10 (C3 of triazole), 151.17 (C5 of triazole), 160.95 (C1 of PkO-), 171.95 (CO); IR (ATR), ν (cm-1): 3393, 3195, 3081, 2961, 2831, 1710, 1689, 1642, 1567, 1546, 1501. Anal. Calcd for C12H13N7O9: C, 36.10; H, 3.28; N, 24.56. Found: C, 36.29; H, 3.17; N, 24.79.
ACKNOWLEDGEMENTS
The authors thank the Federal Agency for Education of Russia for the financial support of this work through the Federal Target Program “Research and Educational Personnel of Innovative Russia at 2009-2013 Years”, State contract P1472, project NK-186P/3.
References
1. (a) A. Dżygiel, B. Rzeszotarska, E. Masiukiewicz, P. Cmoch, and B. Kamieński, Chem. Pharm. Bull., 2004, 52, 192; CrossRef (b) E. Masiukiewicz, B. Rzeszotarska, I. Wawrzycka-Gorczyca, and E. Kołodziejczyk, Synth. Commun., 2007, 37, 1917. CrossRef
2. (a) H.-Ch. Gallmeier and B. König, Eur. J. Org. Chem., 2003, 3473; CrossRef (b) P. G. Baraldi, D. Preti, F. Fruttarolo, M. A. Tabrizi, and R. Romagnoli, Bioorg. Med. Chem., 2007, 15, 17; CrossRef (c) G. Kashiwazaki, T. Bando, K. Shinohara, M. Minoshima, S. Nishijima, and H. Sugiyama, Bioorg. Med. Chem., 2009, 17, 1393. CrossRef
3. N. Fantò, G. Gallo, A. Ciacci, M. Semproni, D. Vignola, M. Quaglia, V. Bombardi, D. Mastroianni, M. P. Zibella, G. Basile, M. Sassano, V. Ruggiero, R. Santis, and P. Carminati, J. Med. Chem., 2008, 51, 1189. CrossRef
4. (a) M. V. Chudinov, I. D. Konstantinova, O. I. Ryzhova, R. S. Esipov, A. M. Yurkevich, V. I. Shvets, and A. I. Miroshnikov, Pharm. Chem. J., 2005, 39, 212; CrossRef (b) M. Uenaka, K. Kawata, M. Nagai, and T. Endoh, US Patent 6831177, 2004 (Chem. Abstr., 2005, 134, 29421); (c) Q. Wu, F. Qu, J. Wan, X. Zhu, Y. Xia, and L. Peng, Helv. Chim. Acta, 2004, 87, 811; CrossRef (d) J. Wan, Y. Xia, Y. Liu, M. Wang, P. Rocchi, J. Yao, F. Qu, J. Neyts, J. L. Iovanna, and L. Peng, J. Med. Chem., 2009, 52, 1144. CrossRef
5. H. Ohnishi, H. Kosuzume, M. Mizota, Y. Suzuki, and E. Mochida, Eur. Patent 150507A2, 1985 (Chem. Abstr., 1985, 104, 68678y).
6. (a) W. Shimizu, US Patent 7097700, 2006 (http://www.freepatentsonline.com/7097700.html); (b) G. Wright and P. Gregory, US Patent 7238228, 2007 (http://www.freepatentsonline.com/7238228.html).
7. H. Park, G. Krigsfeld, S. J. Teat, and J. B. Parise, Cryst. Growth. Des., 2007, 7, 1343. CrossRef
8. A. M. Abdel-Megeed, H. M. Abdel-Rahman, G.-E. S. Alkaramany, and M. A. El-Gendy, Eur. J. Med. Chem., 2009, 44, 117. CrossRef
9. (a) J. Thiele and W. Manchot, Liebigs Ann. Chem., 1898, 303, 33; CrossRef (b) G. Chipen and V. Grinstein, Latv. PSR Zinatnu Akad. Vestis Kim. Ser., 1965, 204 (Chem. Abstr., 1965, 63, 13243f).
10. (a) A. V. Chernysheva, V. M. Chernyshev, P. V. Korolenko, and V. A. Taranushich, Russ. J. Appl. Chem., 2008, 81, 1813; CrossRef (b) V. M. Chernyshev, A. V. Chernysheva, and V. A. Taranushich, Russ. J. Appl. Chem., 2009, 82, 276. CrossRef
11. V. A. Lopyrev, T. N. Vereschagina, G. G. Kononenko, V. V. Makarskii, and K. L. Krupin, USSR Patent 320496, 1971 (Chem. Abstr., 1972, 76, 126990k).
12. (a) R. S. Brown, A. J. Bennet, and H. Slebocka-Tilk, Acc. Chem. Res., 1992, 25, 481; CrossRef (b) A. M. Aman and R. S. Brown, J. Am. Chem. Soc., 1999, 121, 4598. CrossRef
13. A. Albert and E. P. Serjeant, 'The Determination of Ionization Constants. A laboratory manual,' 3rd ed., Chapman and Hall, London, 1984, p. 218.
14. (a) B. Modzelewska-Banachiewicz, J. Banachiewicz, A. Chodkowska, E. Jagiełło-Wójtowicz, and L. Mazur, Eur. J. Med. Chem., 2004, 39, 873; CrossRef (b) M. Ziegler-Borowska, M. Ucherek, J. Kutkowska, L. Mazur, B. Modzelewska-Banachiewicz, D. Kędziera, and A. Kaczmarek-Kędziera, Tetrahedron Lett., 2010, 51, 2951. CrossRef
15. (a) A. V. Dolzhenko, G. Pastorin, A. V. Dolzhenko, and W. K. Chui, Tetrahedron. Lett., 2009, 50, 2124; CrossRef (b) A. V. Dolzhenko, A. V. Dolzhenko, and W. K. Chui, Heterocycles, 2007, 71, 429. CrossRef
16. (a) E. V. Babaev and N. S. Zefirov, Chem. Heterocycl. Compd., 1992, 28, 671; CrossRef (b) E. V. Babaev and N. S. Zefirov, J. Math. Chem., 1992, 11, 65; CrossRef (c) E. V. Babaev, D. E. Lushnikov, and N. S. Zefirov, J. Am. Chem. Soc., 1993, 115, 2416; CrossRef (d) H. C. Van der Plas, 'Advances in Heterocyclic Chemistry: Degenerate Ring Transformations of Heterocyclic Compounds,' Vol. 74, ed. by A. R. Katritzky, Academic Press, Inc., London, 1999, pp. 1-9.
17. (a) G. L’abbé, J. Heterocycl. Chem., 1984, 21, 627; CrossRef (b) N. Vivona, S. Buscemi, V. Frenna, and G. Cusmano, 'Advances in Heterocyclic Chemistry: Ring Transformations of Five-Membered Heterocycles,' Vol. 56, ed. by A. R. Katritzky, Academic Press, Inc., London, 1993, pp. 49-154; (c) F. D’Anna, V. Frenna, S. Guernelli, G. Z. Lanza, G. Macaluso, S. Marullo, and D. Spinelli, ARKIVOC, 2009, viii, 125.
18. G. Kim and G. Keum, Heterocycles, 1997, 45, 1979. CrossRef
19. V. M. Chernyshev, A. V. Chernysheva, and V. A. Taranushich, Russ. J. Appl. Chem., 2006, 79, 783. CrossRef
20. (a) V. I. Minkin, A. D. Garnovskii, J. Elguero, A. R. Katritzky, and O. V. Denisko, 'Advances in Heterocyclic Chemistry: The Tautomerism of Heterocycles: Five-Membered Rings with Two or More Heteroatoms,' Vol. 76, ed. by A. R. Katritzky, Academic Press, Inc., London, 2000, pp. 157-323; (b) G. Karpińska and J. Cz. Dobrowolski, J. Mol. Struct. THEOCHEM, 2008, 853, 7; (c) M. Pagacz-Kostrzewa, R. Bronisz, and M. Wierzejewska, Chem. Phys. Lett., 2009, 473, 238. CrossRef
21. E. Anders, K. Wermann, B. Wiedel, and J.-J. Vanden Eynde, Liebigs. Ann./Recueil, 1997, 745.
22. (a) J. Elguero, A. R. Katritzky, and O. V. Denisko, 'Advances in Heterocyclic Chemistry: Prototropic Tautomerism of Heterocycles: Heteroaromatic Tautomerism – General Overview and Methodology,' Vol. 76, ed. by A. R. Katritzky, Academic Press, Inc., London, 2000, pp. 1-84; (b) R. M. Claramunt, C. López, M. D. Santa María, D. Sanz, and J. Elguero, Prog. Nucl. Magn. Reson. Spectrosc., 2006, 49, 169. CrossRef
23. (a) R. N. Butler, T. McEvoy, E. Alcalde, R. M. Claramunt, and J. Elguero, J. Chem. Soc., Perkin Trans. 1, 1979, 2886; CrossRef (b) T. Winkler and H. Kristinsson, Helv. Chim. Acta, 1983, 66, 694; CrossRef (c) P. Dvortsák, J. Reiter, T. Somorai, and P. Sohár, Magn. Reson. Chem., 1985, 23, 194; CrossRef (d) J. Reiter, L. Pongó, T. Somorai, and P. Dvortsák, J. Heterocycl. Chem., 1986, 23, 401; CrossRef (e) L. Salazar, M. Espada, C. Avendaño, and J. Elguero, J. Heterocycl. Chem., 1990, 27, 1109; CrossRef (f) A. Dżygiel, E. Masiukiewicz, and B. Rzeszotarska, J. Agric. Food. Chem., 2002, 50, 1383; CrossRef (g) V. M. Chernyshev, V. A. Rakitov, V. V. Blinov, V. A. Taranushich, and Z. A. Starikova, Chem. Heterocycl. Compd., 2009, 45, 436; CrossRef (h) M. Bichay, J. W. Fronabarger, R. Gilardi, R. J. Butcher, W. B. Sanborn, M. E. Sitzmann, and M. D. Williams, Tetrahedron. Lett., 2006, 47, 6663. CrossRef
24. H. Xue, S. W. Arritt, B. Twamley, and J. M. Shreeve, Inorg. Chem., 2004, 43, 7972. CrossRef
25. (a) E. Pretsch, P. Bühlmann, and M. Badertscher, 'Structure Determination of Organic Compounds: Tables of Spectral Data,' 4th ed, Springer, Berlin, 2009, p. 436; (b) R. M. Silverstein, F. X. Webster, and D. J. Kiemle, 'Spectrometric identification of organic compounds,' 7th ed., Wiley, New York, 2005, p. 502.
26. (a) M. Burke-Laing and M. Laing, Acta. Cryst. B, 1976, 32, 3216; CrossRef (b) F. H. Allen, O. Kennard, D. G. Watson, L. Brammer, A. G. Orpen, and R. Taylor, J. Chem. Soc., Perkin Trans. 2, 1987, S1-S19. CrossRef
27. According to our unpublished results, for hydrochloride of 4-chlorobenzoic acid 2-guanylhydrazide, 1H NMR (300 MHz, DMSO-d6), δ: 7.57 (br s, 2Н, NH2), 7.64 (d, J = 8.6 Hz, 2Н, arom.), 7.87 (br s, 2Н, NH2), 7.97 (d, J = 8.6 Hz, 2Н, arom.), 9.75 (s, 1Н, NH), 10.78 (s, 1Н, NH); for hydrochloride of acetic acid 2-guanylhydrazide, 1H NMR (600 MHz, DMSO-d6), δ: 1.87 (s, 3H, CH3), 7.57 (br s, 4Н, 2NH2), 9.53 (s, 1Н, NH), 10.10 (s, 1Н, NH).
28. V. M. Chernyshev, A. V. Chernysheva, E. V. Tarasova, V. V. Ivanov, and Z. A. Starikova, Acta Cryst., 2010, E66, o1152.
29. (a) A. K. Tripathi, C. Bruhn, S. Flock, and H. Frauenrath, Acta Cryst., 2005, 61C, o705; (b) G.-L. Zhao, R. Rios, J. Vesely, L. Eriksson, and A. Córdova, Angew. Chem. Int. Ed., 2008, 47, 8468. CrossRef
30. R. N. Shreve and R. K. Charlesworth, US Patent 2744116, 1956 (Chem. Abstr., 1957, 51, 489h).