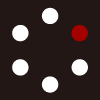
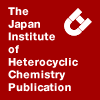
HETEROCYCLES
An International Journal for Reviews and Communications in Heterocyclic ChemistryWeb Edition ISSN: 1881-0942
Published online by The Japan Institute of Heterocyclic Chemistry
e-Journal
Full Text HTML
Received, 17th July, 2010, Accepted, 21st October, 2010, Published online, 29th October, 2010.
DOI: 10.3987/COM-10-12022
■ Regio- and Stereoselective 1,3-Dipolar Cycloaddition of C-Aryl-N-phenylnitrones over (E)-Arylidene-(2H)-indan-1-ones: Synthesis of Highly Substituted Novel Spiro-isoxazolidines
Nadia Wannassi, Mohamed Mehdi Rammah, Sarra Boudriga, Mohamed El Baker Rammah,* Karin Monnier-Jobé,* Kabula Ciamala, Michael Knorr, Mironel Enescu, Yoann Rousselin, and Marek M. Kubicki
Department of Chemistry, Laboratory of Heterocyclic Organic Chemistry, Faculty of Sciences, University of Monastir, Avenue Ali Bourguiba, Skanès
5000 Monastir, Tunisia
Abstract
Synthesis of a series of novel spiro-isoxazolidines has been accomplished in good yields by regio- and diastereoselective 1,3-dipolar cycloaddition of (E)-2-arylidene-(2H)-indan-1-ones 1a-d to C-aryl-N-phenylnitrones 2e-h. The structure of the spiro-adducts 3ae-dh was elucidated by 1H and 13C NMR spectroscopy. The proposed regio- and stereochemistry of spiranic compounds 3ae and 3be has been corroborated by two single crystal X-ray crystallographic analyses and by means of DFT calculations.INTRODUCTION
The 1,3-dipolar cycloaddition reaction between nitrones and unsaturated systems is an efficient method for the organic synthesis of a wide variety of new heterocyclic derivatives structurally related to lactams, indolizidines, alkaloids1 which have found application in the preparation of complex molecules with useful biological activities such as antibiotics and glycosidase inhibitors.2 For example Januário-Charmier et al. 3–5 demonstrated that by conventional organic methods, chiral heterocycles of potential biological activity, can be synthesized, exploring the fact that addition of nucleophiles to a C=C double bond offers an attractive route for the creation of novel C–C, N–O and C–O bonds and for the generation of new isoxazolidine derivatives. These compounds have been for many products syntheses and can be converted to efficient precursors for many synthetic intermediates including β-amino alcohols,6 β-amino acids7 and β-lactams,8 which are useful chiral building blocks for the synthesis of biologically active compounds. Although numerous reports are available for the synthesis of isoxazolidine derivatives,9,10 relatively few work on spiro-isoxazolidine derivatives has been published.
Further, many more indanone derivatives have been used as versatile intermediates for many natural and pharmaceutical products.11-13 The high synthetic utility and pharmacological importance have prompted us to synthesize some spiro-isoxazolidine derivatives which are potentially interesting in pharmaceutical industry.
Since several years, we have focused our studies on the reactivity of dipolarophiles bearing an exocyclic carbon-carbon double bond towards several 1,3-dipole such as nitrile oxides,14 diazoalkanes15 and diarylnitrilimines.16 We particularly paid attention to the role played by the substituents of the dipolar entities on both chemical reactivity and stereochemistry.
In continuation of our interest in the area of cycloaddition reactions, we present here the first examples where C-aryl-N-phenylnitrones 2e-h reacted with (E)-2-arylidene-(2H)-indan-1-ones 1a-d in regio selective manner. Previous to our investigation, to the best of our knowledge, there was only one report of nitrone cycloaddition to trisubstituted olefins where the dipolarophiles had the reacting double bond conjugated with an aryl group and a carbonyl group.17 Note that in the latter work, formation of regioisomers has been observed.
RESULTS AND DISCUSSION
The synthetic route to the targeted spiro-isoxazolidines 3ae-dh is outlined in Scheme 1. Dipolarophiles 1a-d were obtained by the condensation of aromatic aldehydes with indanone.18,19 C-aryl-N-phenylnitrones 2e-h were easily generated by warming aryl aldehydes with N-phenylhydroxylamine in ethanolic solution following a known procedure.20
The initial investigation on the 1,3-dipolar cycloaddition of (E)-2-arylidene-(2H)-indan-1-ones 1a-d with C-aryl-N-phenylnitrones 2e-h involved the reaction of 1b with 2e at 110 °C in toluene solution for 24 h, compound 3be was obtained in only 20% yield. Heating the same mixture at 80 °C for 24 h, afforded the product 3be in 35% yield. Finally, the reaction of 1b with nitrone 2e in toluene at 80 °C for 6 days yielded 3be (65%). Increase in reaction time from 6 days to 10 days did not allow improving the yield further. On the basis of these results, we performed all cycloaddition reactions of dipolarophiles 1a-d with C-aryl-N-phenylnitrones 2e-h at 80 °C in toluene for 6 days (Scheme 1). Yields of cycloadducts 3ae-dh range between 45-70% (Table 1).
The reaction yielded exclusively in a regioselective manner, a series of the spiro-[3,5-diaryl-2-isoxazolidine-4:2’-indan-1’-ones] 3ae-dh. The structure of each product was established on the basis of spectroscopic and crystallographic analysis. In each case, the presence of traces of starting reactants was also evidenced by TLC and 1H NMR examination of the crude reaction mixture. These findings indicate that the reaction reaches an equilibrium state after 6 days. We suggest that the selectivity should be, at least in part, thermodynamically controlled.
The reaction is: (i) regioselective, since 3ae-dh with the oxygen of the nitrone added to the β-carbon of the dipolarophile and (ii) stereoselective, as the two aryl of isoxazolidine ring of 3ae-dh are cis-arranged. The regioselectivity is not unexpected since many examples have been reported of nitrone additions to electron deficient alkenes to afford isoxazolidines with the oxygen in β-position to the electron-withdrawing group.21-23
The 1H NMR spectra (300 MHz) exhibits a singlet between 5.40 ppm and 5.65 ppm assigned to the 3-H proton. This excludes the presence of the inverse regioisomer, for which the NMR spectrum would exhibit a doublet for 3-H. This was confirmed by the 13C NMR chemical shift of the spirocarbon (C-4,2’) atoms of all adducts, which have been found between 68.69 ppm and 70.31 ppm. This range is in agreement with the remoteness from the isoxazolidinic oxygen atom. 24,25 In the case of the hypothetic isomeric structures 3’ae-dh, the chemical shift values of spiro carbon atoms (C-5,2’) should be around 82-84 ppm.26-29 The cycloaddition of (E)-2-arylidene-(2H)-indan-1-ones 1a-d with C-aryl-N-phenylnitrones 2e-h led to cycloadducts 3ae-dh with three new chiral centers, i.e. the quaternary spiroatom C-4,2’; C-3 and the C-5 of isoxazolidine ring (Scheme 1). The relative stereochemistry of these carbon [rel-(3S, 4,2’R, 5R)] results from (i) preservation of the (E) configuration of the initial olefin and (ii) concerted reaction of (Z)-nitrones 2e-h over 1a-d (Scheme 1). The formation of diastereoisomeric adducts has never been caused by any Z/E interconversion of nitrones, 27-29 the relative configuration (Z) of the dipole always being preserved in spiro-compounds (Figure 2, Scheme 1).
Furthermore, the suggested regio- and stereochemistry of 3ae-dh are supported by X-ray analyses carried out on 3ae (Figure 2) and 3be (Figure 3). Before commenting these structures, one may consider different approches during the course of the cycloaddition.
The two approach modes (endo-C=O and exo-C=O) of the C-aryl-N-phenylnitrones 2e-h towards dipolarophiles 1a-d are depicted in Scheme 2.
In order to clarify the origin of the selective formation of the endo –C=O product, we performed density functional theory (DFT) optimisations of the complex of reactants in toluene at the B3LYP/6-31G(d) level of theory. In these calculations the solvent was treated using the polarisable continuum dielectric model of Cossi et al.30 The calculations were performed with the Gaussian 09 package. 31 We found that the reactants form a site-to-site complex of endo –C=O type (Figure 1) that is very likely the precursor of an endo –C=O transition state. It is well known that the site-to-site configuration is characteristic to complexes mainly stabilized by electrostatic interactions. In the present case the complex appears to be stabilized by hydrogen bonds involving the oxygen atoms carried by the two reactants. On the other hand, an analogous conformation of the complex of the exo –C=O type was not identified in the calculations. We conclude that the endo –C=O selectivity is due to a favourable electrostatic interaction between the two reactants.
Only diastereoisomers 3ae and 3be resulting from the cycloaddition of (E)-2-(benzylidene)-(2H)-indan-1-one 1a and (E)-2-(p-methylbenzylidene)-(2H)-indan-1-ones 1b with C,N-diphenylnitrone 2b gave crystals of X-ray quality.
Because the space groups of the solid-state structures of both compounds contain the symmetry centres, the diastereoisomers R(C10)S(C23) and S(C10)R(C23) (X-ray ORTEP label C10 corresponds to C3, whereas C23 corresponds to C5 of Scheme 1) coexist in their racemic crystals. The H10 (3-H) and H23 (5-H) hydrogen atoms as well as the O2 one of carbonyl indanone ring point towards the same side of isoxazolidine ring. A resulting endo (or cis) type geometry is more favourable since repulsion between the O2 atom of indanone and the O1 and N1 atoms of central ring is thus avoided.
Because of the presence of three adjacent sp3 carbon atoms (C10, C1 and C23), the isoxazolidine rings deviate strongly from planarity. The C10/N1/O1/C23 fragment is planar within 0.05 and 0.10 Å in 3ae and 3be, respectively. The dihedral angles with the C10/C1/C23 plane are equal to 42.4(9) and 40.1(1)° in 3ae and 3be, respectively. Contrary to the isoxazolidine ring, the five-membered indanone ring (one sp3 carbon) fits very well the best least-square plane with highest deviations from reaching only 0.01 and 0.009 Å, respectively. The spiranic dihedral angles of 89.5(1) 3ae and 87.8(1)° 3be are close to the ideal value of 90° for regular tetrahedral Td spirane. The metric parameters are normal in both structures.
CONCLUSION
In conclusion, we have shown that cycloaddition reaction of (E)-2-arylidene-(2H)-indan-1-ones 1a-d with nitrones 2e-h leads regio- and diastereoselectively (100%) to spiro-[3,5-diaryl-2-isoxazolidine-4:2’-indan-1’-ones]. The regiochemistry of the reaction is independent to the electronic nature of the substituents at the para-position on the dipolarophile as well as on the dipole. The exclusive stereoselectivity of the reaction has been rationalised to be a consequence of combined electronic and steric interactions of the reagents during their approaches. The diastereoisomers 3ae-dh are the result of the preferential approach directing the indanone carbonyl group in the endo position relative to the dipolar linkage. Apart from the regioselectivity aspect, the novel spiro-isoxazolidines presented in this work should be of interest as precursors for the synthesis of a variety of amino-alcohols derivatives.
EXPERIMENTAL
General Remarks: Reactions were carried out under an atmosphere of dry N2. Solvents were purified by standard methods and freshly distilled under nitrogen and dried before use.
Melting points were determined on a Kofler bank. IR spectra were recorded from KBr on a Perkin-Elmer 197 spectrometer; only structurally significant bands are reported. NMR spectra were recorded with a Bruker-Spectrospin AC 300 spectrometer operating at 300 MHz for 1H and 75.5 MHz for 13C using tetramethylsilane (TMS) as the internal standard (0.00 ppm) in CDCl3 as solvent. Chemical shifts were reported in ppm (δ) downfield from TMS. The following abbreviations were used to explain the multiplicities: s =singlet, d = doublet, t = triplet, m = multiplet.
Elemental analyses (C, H, N) were conducted on a Leco Elemental CHN 900; values were in satisfactory agreement with the calculated ones (0.30%). Materials: thin-layer chromatography (TLC): TLC plates (Merck, silica gel 60 F254 0.2 mm 200×200 nm); substances were detected using UV light at 254 nm.
(E)-2-Arylidene-(2H)-indan-1-ones 1a-d were obtained by condensation of aldehydes ArCHO with respectively indanone according to reported methods.28,29,32 C-Aryl-N-phenylnitrones 2e-h was synthesized as reported by condensation of aldehydes Ar’CHO with phenylhydroxylamine.33
General procedure for the preparation of the cycloadducts (3ae-dh)
A solution of dipolarophiles 1a-d (3 mmol) and C-aryl-N-phenylnitrones (3 mmol) 2e-h in dry toluene (10 mL), was stirred at 80 °C for 6 days. The solvent was evaporated under reduced pressure and the residue was purified by column chromatography on silica gel eluted with hexane/EtOAc (20:80) to afford compounds 3ae-dh.
Spiro[3,5-diphenyl -2-isoxazolidine-4:2’-indan-1’-ones] (3ae)
Yield 0.56 g (45%); white solid; mp 122 °C; IR (KBr, cm-1): 1751, 1615; 1H NMR (CDCl3): δ 2.75 (d, J= 18 Hz, 3’-H); 2.85 (d, J= 18 Hz, 3’-H); 5.45 (s, 3-H); 5.60 (s, 5-H); 6.78-8.30 (m, aromatic H); 13C NMR (CDCl3): δ 30.57 (C-3’); 70.31 (C-4.2’); 79.20 (C-3); 86.64 (C-5); 114.95-154.24 (aromatic C); 204.36 (C-1’); Anal. Calcd for C29H23NO2: C, 83.43; H, 5.55; N, 3.35. Found: C, 83.25; H, 5.40; N, 3.21.
Spiro[3-phenyl-5-(p-tolyl)-2-isoxazolidine-4:2’-indan-1’-one] (3af)
Yield 0.82 g (64%); yellow solid; mp 126 °C; IR (KBr, cm-1): 1740, 1613; 1H NMR (CDCl3): δ 2.20 (s, CH3); 2.75 (d, J= 18 Hz, 3’-H); 2.85 (d, J= 18 Hz, 3’-H); 3’-H); 5.40 (s, 3-H); 5.60 (s, 5-H); 6.85-7.75 (m, aromatic H); 13C NMR (CDCl3): δ 21.44 (CH3); 30.53 (C-3’); 70.25 (C-4,2’); 79.02 (C-3); 86.62 (C-5); 114.96-154.32 (aromatic C); 204.40 (C-1’). Anal. Calcd for C30H25NO2: C, 83.50; H, 5.84; N, 3.25. Found: C, 83.29; H, 5.96; N, 3.34.
Spiro[5-(p-anisyl)-3-phenyl-2-isoxazolidine-4:2’-indan-1’-one] (3ag)
Yield 0.80 g (60%); yellow solid; mp 165 °C; IR (KBr, cm-1): 1742, 1612; 1H NMR (CDCl3): δ 2.83 (d, J= 18 Hz, 3’-H); 2.93 (d, J= 18 Hz, 3’-H); 3.75 (s, OCH3); 5.47 (s, 3-H); 5.50 (s, 5-H); 6.75 -7.70 (m, aromatic H);13C NMR (CDCl3): δ 30.19 (C-3’); 55.30 (s, OCH3); 69.90 (C-4,2’); 78.45 (C-3); 86.57 (C-5); 113.40-159.20 (aromatic C); 204.28 (C-1’). Anal. Calcd for C30H25NO3: C, 80.51; H, 5.63; N, 3.13. Found: C, 80.25; H, 5.35; N, 2.97.
Spiro[5-(p-chlorophenyl)-3-phenyl-2-isoxazolidine-4:2’-indan-1’-one] (3ah)
Yield 0.67g (50%); white solid; mp 149 °C; IR (KBr, cm-1): 1753, 1613; 1H NMR (CDCl3): δ 2.90 (d, J= 18 Hz, 3’-H); 3.00 (d, J= 18 Hz, 3’-H); 5.63 (s, 3-H); 5.72 (s, 5-H); 7.03-7.85 (m, aromatic H); 13C NMR (CDCl3): δ 29.06 (C-3’); 68.69 (C-4,2’); 77.72 (C-3); 83.95 (C-5); 113.60-152.64 (aromatic C); 202.67 (C-1’). Anal. Calcd for C29H22NO2Cl: C, 77.07; H, 4.91; N, 3.10. Found: C, 76.89; H, 4.72; N, 3.33.
Spiro[5-phenyl-3-(p-tolyl)- 2-isoxazolidine-4:2’-indan-1’-one] (3be)
Yield 0.84g (65%); yellow solid; mp 126 °C; IR (KBr, cm-1): 1755, 1613; 1H NMR (CDCl3): δ 2.27 (s, CH3); 2.84 (d, J= 18 Hz, 3’-H); 2.94 (d, J= 18 Hz, 3’-H); 5.54 (s, 3-H); 5.63 (s, 5-H); 6.91-7.76 (m, aromatic H); 13C NMR (CDCl3): δ 21.19 (CH3); 30.16 (C-3’); 69.86 (C-4,2’); 78.69 (C-3); 86.31 (C-5); 114.57-154.01 (aromatic C); 204.14 (C-1’). Anal. Calcd for C30H25NO2: C, 83.50; H, 5.84; N, 3.25. Found: C, 83.33; H, 5.58; N, 3.06.
Spiro[3,5-di(p-tolyl)-2-isoxazolidine-4:2’-indan-1’-one] (3bf)
Yield 0.80 g (60%); yellow solid; mp 195 °C; IR (KBr, cm-1): 1757, 1614; 1H NMR (CDCl3): δ 2.40 (s, CH3); 2.42 (s, CH3); 2.80 (d, J= 18 Hz, 3’-H); 2.90 (d, J= 18 Hz, 3’-H); 5.45 (s, 3-H); 5.59 (s, 5-H); 6.87-7.77 (m, aromatic H); 13C NMR (CDCl3): δ 21.30 (s, CH3); 21.35 (s, CH3); 30.33 (C-3’); 70.14 (C-4,2’); 78.74 (C-3); 86.42 (C-5); 114.62-154.24 (aromatic C); 204.26 (C-1’). Anal. Calcd for C31H27NO2: C, 83.57; H, 6.11; N, 3.14. Found: C, 83.75; H, 6.25; N, 2.97.
Spiro[5-(p-anisyl)-3-(p-tolyl)-2-isoxazolidine-4:2’-indan-1’-one] (3bg)
Yield 0.96 g (70%); yellow solid; mp 190 °C; IR (KBr, cm-1): 1754, 1614; 1H NMR (CDCl3): δ 2.40 (s, CH3); 2.96 (d, J= 18 Hz, 3’-H); 3.06 (d, J= 18 Hz, 3’-H); 3.80 (s, OCH3); 5.46 (s, 3-H); 5.63 (s, 5-H); 6.70-7.75 (m, aromatic H); 13C NMR (CDCl3): δ 21.51 (s, CH3); 30.35 (C-3’); 55.61 (s, OCH3); 70.10 (C-4,2’); 78.35 (C-3); 85.98 (C-5); 113.62-158.54 (aromatic C); 204.26 (C-1’). Anal. Calcd for C31H27NO3: C, 80.67; H, 5.90; N, 3.03. Found: C, 80.82; H, 5.62; N, 2.91.
Spiro[5-(p-chlorophenyl)-3-(p-tolyl)- 2-isoxazolidine-4:2’-indan-1’-one] (3bh)
Yield 0.72 g (52%); white solid; mp 175 °C; IR (KBr, cm-1): 1735, 1612; 1H NMR (CDCl3): δ 2.45 (s, CH3); 3.07 (d, J= 18 Hz, 3’-H); 3.17 (d, J= 18 Hz, 3’-H); 5.61 (s, 3-H); 5.73 (s, 5-H); 7.26-7.85 (m, aromatic H); 13C NMR (CDCl3): δ 22.40 (CH3); 30.40 (C-3’); 68.77 (C-4,2’); 77.73 (C-3); 85.45 (C-5); 115.64-153.30 (aromatic C); 204.20 (C-1’). Anal. Calcd for C30H24NO2Cl: C, 77.33; H, 5.19; N, 3.01. Found: C, 77.13; H, 5.04; N, 3.20.
Spiro[3-(p-anisyl)-5-phenyl-2-isoxazolidine-4:2’-indan-1’-one] (3ce)
Yield 0.67 g (50%); yellow solid; mp 186 °C; IR (KBr, cm-1): 1732, 1615; 1H NMR (CDCl3): δ 2.84 (d, J= 18 Hz, 3’-H); 2.94 (d, J= 18 Hz, 3’-H); 3.77 (s, OCH3); 5.48 (s, 3-H); 5.52 (s, 5-H); 6.73 -7.85 (m, aromatic H); 13C NMR (CDCl3): δ 30.25 (C-3’); 55.31 (s, OCH3); 69.95 (C-4,2’); 78.46 (C-3); 86.60 (C-5); 113.45-159.25 (aromatic C); 204.30 (C-1’). Anal. Calcd for C30H25NO3: C, 80.51; H, 5.63; N, 3.13. Found: C, 80.34; H, 5.37; N, 2.94.
Spiro[3-(p-anisyl)-5-(p-tolyl)-2-isoxazolidine-4:2’-indan-1’-one] (3cf)
Yield 0.69 g (50%); yellow solid; mp 180 °C; IR (KBr, cm-1): 1754, 1612; 1H NMR (CDCl3): δ 2.39 (s, CH3); 2.92 (d, J= 18 Hz, 3’-H); 3.02 (d, J= 18 Hz, 3’-H); 3.85 (s, OCH3); 5.48 (s, 3-H); 5.60 (s, 5-H); 6.70-7.75 (m, aromatic H); 13C NMR (CDCl3): δ 21.50 (s, CH3); 30.89 (C-3’); 55.65 (s, OCH3); 70.12 (C-4,2’); 78.40 (C-3); 85.97 (C-5); 113.62-158.55 (aromatic C); 204.27 (C-1’). Anal. Calcd for C31H27NO3: C, 80.67; H, 5.90; N, 3.03. Found: C, 80.79; H, 6.03; N, 2.88.
Spiro[3,5-di(p-anisyl)-2-isoxazolidine-4:2’-indan-1’-one] (3cg)
Yield 0.70 g (49%); yellow solid; mp 138 °C; IR (KBr, cm-1): 1752, 1613; 1H NMR (CDCl3): δ 2.84 (d, J= 18 Hz, 3’-H); 2.94 (d, J= 18 Hz, 3’-H); 3.70 (s, OCH3); 3.74 (s, OCH3); 5.47 (s, 3-H); 5.59 (s, 5-H); 6.68-7.73 (m, aromatic H); 13C NMR (CDCl3): δ 30.04 (C-3’); 55.23 (OCH3); 55.28 (OCH3); 69.97 (C-4,2’); 78.52 (C-3); 86.14 (C-5); 113.54-159.31 (aromatic C); 204.29 (C-1’). Anal. Calcd for C31H27NO4: C, 77.97; H, 5.70; N, 2.93. Found: C, 78.14; H, 5.44; N, 2.67.
Spiro[3-(p-anisyl)-5-(p-chlorophenyl)-2-isoxazolidine-4:2’-indan-1’-one] (3ch)
Yield 0.79 g (55%); white solid; mp 196 °C; IR (KBr, cm-1): 1753, 1613; 1H NMR (CDCl3): δ 2.85 (d, J= 18 Hz, 3’-H); 2.95 (d, J= 18 Hz, 3’-H); 3.84 (s, OCH3); 5.49 (s, 3-H); 5.61 (s, 5-H); 6.72-7.75 (m, aromatic H); 13C NMR (CDCl3): δ 30.42 (C-3’); 55.25 (OCH3); 70.12 (C-4,2’); 78.55 (C-3); 85.30 (C-5); 113.62-160.40 (aromatic C); 204.25 (C-1’). Anal. Calcd for C30H24NO3Cl: C, 74.76; H, 5.02; N, 2.91. Found: C, 74.63; H, 5.23; N, 2.67.
Spiro[3-(p-chlorophenyl)-5-phenyl-2-isoxazolidine-4:2’-indan-1’-one] (3de)
Yield 0.81 g (60%); yellow solid; mp 176 °C; IR (KBr, cm-1): 1755, 1614; 1H NMR (CDCl3): δ 2.84 (d, J= 18 Hz, 3’-H); 2.94 (d, J= 18 Hz, 3’-H); 5.65 (s, 3-H); 5.69 (s, 5-H); 6.99-7.80 (m, aromatic H); 13C NMR (CDCl3): δ 29.26 (C-3’); 68.73 (C-4,2’); 77.68 (C-3); 83.98 (C-5); 114.30-155.25 (aromatic C); 203.99 (C-1’). Anal. Calcd for C29H22NO2Cl: C, 77.07; H, 4.91; N, 3.10. Found: C, 76.90; H, 4.73; N, 3.30.
Spiro[3-(p-chlorophenyl)-5-(p-tolyl)-2-isoxazolidine-4:2’-indan-1’-one] (3df)
Yield 0.62 g (45%); yellow solid; mp 230 °C; IR (KBr, cm-1): 1753, 1614 cm-1; 1H NMR (CDCl3): δ 2.40 (s, CH3); 3.09 (d, J= 18 Hz, 3’-H); 3.19 (d, J= 18 Hz, 3’-H); 5.63 (s, 3-H); 5.70 (s, 5-H); 7.26-7.85 (m, aromatic H); 13C NMR (CDCl3): δ 22.39 (CH3); 30.36 (C-3’); 68.75 (C-4,2’); 77.70 (C-3); 85.41 (C-5); 115.63-155.27 (aromatic C); 202.71 (C-1’). Anal. Calcd for C30H24NO2Cl: C, 77.33; H, 5.19; N, 3.01. Found: C, 77.10; H, 5.06; N, 3.19.
Spiro[5-(p-anisyl)-3-(p-chlorophenyl)-2-isoxazolidine-4:2’-indan-1’-one] (3dg)
Yield 0.72 g (50%); yellow solid; mp 188 °C; IR (KBr, cm-1): 1752, 1615 cm-1; 1H NMR (CDCl3): δ 2.87 (d, J= 18 Hz, 3’-H); 2.97 (d, J= 18 Hz, 3’-H); 3.82 (s, OCH3); 5.50 (s, 3-H); 5.62 (s, 5-H); 7.71-7.77 (m, aromatic H); 13C NMR (CDCl3): δ 30.140 (C-3’); 55.26 (OCH3); 70.10 (C-4,2’); 78.48 (C-3); 85.37 (C-5); 113.60-160.43 (aromatic C); 203.98 (C-1’). Anal. Calcd for C30H24NO3Cl: C, 74.76; H, 5.02; N, 2.91. Found: C, 74.62; H, 5.25; N, 2.68.
Spiro[3,5-di(p-chlorophenyl)-2-isoxazolidine-4:2’-indan-1’-one] (3dh)
Yield 0.72 g (50%); white solid; mp 170 °C; IR (KBr, cm-1): 1751, 1615; 1H NMR (CDCl3): δ 2.90 (d, J= 18 Hz, 3’-H); 3.00 (d, J= 18 Hz, 3’-H); 5.62 (s, 3-H); 5.71 (s, 5-H); 7.69-7.79 (m, aromatic H); 13C NMR (CDCl3): δ 30.15 (C-3’); 70.16 (C-4,2’); 78.52 (C-3); 85.91 (C-5); 113.58-160.40 (aromatic C); 204.18 (C-1’). Anal. Calcd for C29H21NO2Cl2: C, 71.61; H, 4.35; N, 2.88. Found: C, 71.43; H, 4.44; N, 2.76.
X-Ray structure analyses
X-Ray quality colorless crystals of 3ae (0.42x0.20x0.20 mm) and 3be (0.30x0.25x0.07 mm), grown from ethanol solutions, were mounted on a Nonius KappaCCD diffractometer. Intensity data were collected at 115 K with Mo-Kα radiation. The crystal structures were solved by direct methods34 and refined with full-matrix least squares on F2.35 Anisotropic thermal parameters were applied for non-hydrogen atoms. All H atoms were placed in calculated positions and included in final refinements in a riding model with isotropic temperature factors related to the carbon atoms bearing them: Uiso(H) = 1.2Ueq(C). There is a slight disorder in both structures. It concernes the four aromatic CH groups of indanone ring in 3ae (refined occupancies are 0.55/0.45), whereas in 3be the disorder is observed for phenyl group bound to C10 atom of the central ring with occupancies 0.61/0.39. These disorders are certainly due to the crystal packing.
X-Ray crystal structure analyses of compounds (3ae) and (3be):
For C29H23NO2 : M = 417.48, monoclinic, space group P21/c, a = 10.2526(1) Å, b = 19.8492(3) Å, c = 11.1911(2) Å, β = 104.151(1)°, V = 2208.34(6) Å3, Z = 4, Dc = 1.256 Mg/m3, λ(Mo-Kα) = 0.71073 Å, F(000) = 880, µ(Mo-Kα) = 0.078 mm-3, T = 115(2) K. 9878 reflections collected, 5022 unique and 3881 with I>2σ(I). Final residuals ρmax = 0.247, ρmin = -0.192 e-/Å3. Final agreement factors: R(F) = 0.0555 and 0.0757, wR(F2) = 0.1259 and 0.1325 for I>2σ(I) and all data, respectively, GOF = 1.143.
For C30H25NO2 : M = 431.51 triclinic, space group P-1, a = 8.3336(2) Å, b = 9.5701(3) Å, c = 15.3515(4) Å, α = 81.393(2)°, β = 79.535(2)°, γ = 68.349(1)°, V = 1114.44(5) Å3, Z = 2, Dc = 1.286 Mg/m3, λ(MoKα) = 0.71073 Å, F(000) = 456, µ(Mo-Kα) = 0.080 mm-3, T = 115(2) K. 9798 reflections collected, 5101 unique and 3448 with I>2σ(I). Final residuals ρmax = 0.328, ρmin = -0.298 e-/Å3. Final agreement factors: R(F) = 0.0496 and 0.0845, wR(F2) = 0.1195 and 0.1360 for I>2σ(I) and all data, respectively, GOF = 1.050.
Crystallographic data for the structures of 3ae and 3be have been deposited with the Cambridge Crystallographic Data Centre as supplementary publication number 782615 and 782616. Copy of these data can be obtained, free of charge, on application to CCDC, 12 Union Road, Cambridge CB2 IEZ, UK, fax: 144-(0)1223-336033 or e-mail: deposit@ccdc.cam.ac.uk.
References
1. G. A. Schiehser, J. D. White, G. Matsumoto, J. O. Pezzanite, and J. Clardy, Tetrahedron Lett., 1986, 27, 5587; CrossRef A. E. McCaig and R. H. Wightman, Tetrahedron Lett., 1993, 34, 3939; CrossRef A. Goti, F. Cardona, A. Brandi, S. Picasso, and P. Vogel, Tetrahedron: Asymmetry, 1996, 7, 1659. CrossRef
2. Z. Guo and P. J. Sadler, Adv. Inorg. Chem., 1999, 49, 183; CrossRef Z. Guo and P. J. Sadler, Angew. Chem. Int. Ed., 1999, 38, 1512; CrossRef K. V. Gothelf and K. A. Jørgensen, Chem. Rev., 1998, 98, 863. CrossRef
3. M. O. Januário-Charmier, N. Moussalli, J. Chanet, and S. Chou, J. Chem. Res. (S), 1999, 566.
4. M. T. Barros, M. A. Januário-Charmier, C. D. Maycock, and T. Michaud, Tetrahedron, 2002, 58, 1519. CrossRef
5. J. Chanet, M. O. Januário-Charmier, R. Vessière, and M. F. Zuccarelli, J. Heterocycl. Chem., 1994, 31, 1667. CrossRef
6. A. Vasella and R. Voeffray, J. Chem. Soc., Chem. Commun., 1981, 97; CrossRef A. Vasella and R. Voeffray, Helv. Chim. Acta, 1982, 65, 1134. CrossRef
7. D. Kiers, D. Moffat, and K. Overton, J. Chem. Soc., Chem. Commun., 1988, 654; CrossRef D. Kiers, D. Moffat, K. Overton, and R. Tomanek, J. Chem. Soc., Perkin Trans. 1, 1991, 1041; CrossRef C. M. Tice and B. Ganem, J. Org. Chem., 1983, 48, 5048. CrossRef
8. I. Panfil, C. Belzecki, Z. Urbanczyk-Lipkowska, and M. Chmielewski, Tetrahedron, 1991, 47, 10087; CrossRef I. Panfil, C. Belzecki, and M. Chmielewski, J. Carbohydr. Chem., 1987, 6, 463; CrossRef I. Panfil, C. Belzecki, M. Chmielewski, and K. Suwinska, Tetrahedron, 1989, 45, 233; CrossRef I. Panfil, M. Chmielewski, and C. Belzecki, Heterocycles, 1986, 24, 1609. CrossRef
9. R. S. Kumar, S. Perumal, K. A. Shetty, Y. Perumal, and D. Sriram, Eur. J. Med. Chem., 2010, 45, 124. CrossRef
10. J. Revuelta, S. Cicchi, A. De Meijere, and A. Brandi, Eur. J. Org. Chem., 2008, 6, 1085. CrossRef
11. H. Sugimoto, Pure Appl. Chem., 1999, 71, 2031. CrossRef
12. W. M. Welch, A. R. Kraska, R. Sarges, and B. K. Kow, J. Med. Chem., 1984, 27, 1508. CrossRef
13. R. A. Stalker, T. E. Munsch, J. D. Tran, X. Nie, R. Warmuth, A. Beatty, and C. B. Aakeroy, Tetrahedron, 2002, 58, 4837. CrossRef
14. M. Askri, N. Jgham, M. Rammah, K. Ciamala, K. Monnier-Jobé, and J. Vebrel, Heterocycles, 2007, 71, 289. CrossRef
15. M. Askri, N. Ben Hamadi, M. Msadek, and M. E. Rammah, J. Soc. Chim. Tunisie, 2006, 8, 219.
16. S. Boudriga, M. Askri, R. Gharbi, M. Rammah, and K. Ciamala, J. Chem. Research (S), 2003, 204.
17. R. R. Kumar, S. Perumal, H. B. Kagan, and R. Guillot, Tetrahedron, 2006, 62, 12380. CrossRef
18. M. Msaddek, M. Rammah, K. Ciamala, J. Vebrel, and B. Laude, Bull. Soc. Chim. Belg., 1997, 106, 825.
19. N. Barbier, Heterocycles, 1988, 27, 955. CrossRef
20. S. R. Sandler and W. Karo, Organic Functional Group Preparations, 2nd ed, Academic Press, San Diego, 1989, 3, 351.
21. B. De Lange and B.L. Feringa, Tetrahedron Lett., 1988, 29, 5317. CrossRef
22. P. Cid, P. de March, M. Figueredo, J. Font, and S. Milán, Tetrahedron Lett., 1992, 33, 667; CrossRef M. Burdisso, A. Gamba, and R. Gandolfi, Tetrahedron, 1988, 44, 3735. CrossRef
23. R. Grigg, J. Markandu, T. Perrior, S. Surendrakumar, and W. J. Warnock, Tetrahedron, 1992, 48, 6929; CrossRef A. J. Blake, A. C. Forsyth, and R. M. Paton, J. Chem. Soc., Chem. Commun., 1988, 440; CrossRef Sk. A. Ali and H. P. Perzanowski, J. Chem. Res., 1992, 146.
24. Raunak, V. Kumar, S. Mukherjee, Poonam, A. K. Prasad, C. E. Olsen, S. J. C. Schäffer, S. K. Sharma, A. C. Watterson, W. Errington, and V. S. Parmar, Tetrahedron, 2005, 61, 5687. CrossRef
25. T. Ueda, M. Inada, I. Okamoto, N. Morita, and O. Tamura, Org. Lett., 2008, 10, 2043. CrossRef
26. C. Roussel, R. Fihi, K. Ciamala, J. Vebrel, T. Zair, and C. Riche, Org. Biomol. Chem., 2003, 1, 2689. CrossRef
27. S. Rigolet, J. M. Mélot, J. Vebrel, A. Chiaroni, and C. Riche, J. Chem., Perkin Trans., 1, 2000, 1095.
28. M. Cacciarini, F. M. Cordero, C. Faggi, and A. Goti, Molecules, 2000, 5, 637. CrossRef
29. C. Roussel, K. Ciamala, J. Vebrel, and C. Riche, Heterocycles, 2009, 78, 1977. CrossRef
30. M. Cossi, G. Scalmani, N. Rega, and V. Barone, J. Chem. Phys., 2002, 117, 43. CrossRef
31. Gaussian 09, Revision A.1, M. J. Frisch, G. W. Trucks, H. B. Schlegel, G. E. Scuseria, M. A. Robb, J. R. Cheeseman, G. Scalmani, V. Barone, B. Mennucci, G. A. Petersson, H. Nakatsuji, M. Caricato, X. Li, H. P. Hratchian, A. F. Izmaylov, J. Bloino, G. Zheng, J. L. Sonnenberg, M. Hada, M. Ehara, K. Toyota, R. Fukuda, J. Hasegawa, M. Ishida, T. Nakajima, Y. Honda, O. Kitao, H. Nakai, T. Vreven, J. A. Montgomery, Jr., J. E. Peralta, F. Ogliaro, M. Bearpark, J. J. Heyd, E. Brothers, K. N. Kudin, V. N. Staroverov, R. Kobayashi, J. Normand, K. Raghavachari, A. Rendell, J. C. Burant, S. S. Iyengar, J. Tomasi, M. Cossi, N. Rega, J. M. Millam, M. Klene, J. E. Knox, J. B. Cross, V. Bakken, C. Adamo, J. Jaramillo, R. Gomperts, R. E. Stratmann, O. Yazyev, A. J. Austin, R. Cammi, C. Pomelli, J. W. Ochterski, R. L. Martin, K. Morokuma, V. G. Zakrzewski, G. A. Voth, P. Salvador, J. J. Dannenberg, S. Dapprich, A. D. Daniels, Ö. Farkas, J. B. Foresman, J. V. Ortiz, J. Cioslowski, and D. J. Fox, Gaussian, Inc., Wallingford CT, 2009..
32. K. B. G. Torsell, in Nitrile Oxides, Nitrones, and nitronates in Organic Synthesis, ed. by H. Feuer, VCH Publishers, New York, 1988.
33. J. J. Tufariello, In 1,3-Dipolar Cycloaddition Chemistry; ed. by A. Padwa, John Wiley & Sons: New York, 1984.
34. SIR92 program, A. Altomare, G. Cascarano, C. Giacovazzo, and A. Guagliardi, J. Appl. Crystallogr., 1993, 32, 115. CrossRef
35. SHELXL-97, Program for the Refinement of Crystal Structures, Sheldrick, G. M., 1997, University of Göttingen, Göttingen, Germany.