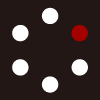
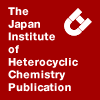
HETEROCYCLES
An International Journal for Reviews and Communications in Heterocyclic ChemistryWeb Edition ISSN: 1881-0942
Published online by The Japan Institute of Heterocyclic Chemistry
e-Journal
Full Text HTML
Received, 21st July, 2010, Accepted, 17th August, 2010, Published online, 18th August, 2010.
DOI: 10.3987/COM-10-12023
■ The Unexpected Formation and Structure of 4,6-Dimethylthieno[3,4-c]thiophene-1(3H)-thione
Nathan C. Tice,* Sarah M. Peak, and Sean Parkin
Department of Chemistry, Eastern Kentucky University, Richmond, KY, 40475-3102, U.S.A.
Abstract
The reaction of 2,5-dimethylthiophene-3,4-dicarboxaldehye (1) with excess Lawesson’s Reagent afforded the unexpected 5,5-fused ring thienothione derivative, 4,6-dimethylthieno[3,4-c]thiophene-1(3H)-thione (2), in good yield (79%). The desired non-classical thiophene, 1,3-dimethylthieno[3,4-c]thiophene (3), was observed by GC/MS as a minor product (approximately 5%) along with thione 2. The thienothione 2 was characterized spectroscopically and its structure was confirmed by X-ray crystallography. The formation of compound 2 is proposed to occur through a 1,3-hydride shift.Thiophenes and their fused-ring derivatives have long been of interest for use in next generation electronic materials due to their ease of production, synthetic versatility, and low cost compared to traditional inorganic materials (e.g., silicon).1,2 We are primarily interested in facile pathways towards novel thiophene derivatives that incorporate other heteroatoms into fully aromatic systems. The incorporation of heteroatoms like sulfur into electronic materials is thought to be advantageous due to their ability to stabilize positive charge in p-type semiconductors.3 One such class of heterocycles we have been particularly interested in is thienothiophenes (Figure 1). These can exist in either the “b” or “c” forms and have potential applications in a wide variety of optical and electronic systems.4-6 Previously, we reported upon the synthesis of a 5,6-fused thienopyridazine from its diformyl precursor.7 However, we wished to explore the analogous ring closure chemistry with thiating reagents and see whether stable sulfur products could be obtained. Herein we report upon the unexpected formation and characterization of 4,6-dimethylthieno[3,4-c]thiophene-1(3H)-thione (2) by thiation of 2,5-dimethylthiophene-3,4-dicarboxaldehye (1).
Although not as common as the “classic” or “b-type” thienothiophenes, “non-classical or “c-type” compounds have been observed (Figure 1).4 Syntheses of these types of fused-thiophenes has typically been accomplished from a 1,4-diketo thiophene precursor, with the reduced or dihydrothienothiophene as the initial ring closed species.8 Formation of the oxide followed by dehydration would afford the corresponding non-classical thienothiophene. Unsubstituted non-classical thiophenes have been found to be unstable,9,10 while those with electron withdrawing groups (EWGs) display high stability in the solid state.11, 12 For example, Cava and coworkers used 3,4-biscyanomethyl-2,5-methoxyesterthiophene and SOCl2 as the thiating regent to afford the desired thieno[3,4-c]thiophene in good yield (70%).12 Other more elaborate routes have employed substituted cyclopropenethiones and trialkyl- or triarylphosphines to give non-classical forms.13-16
We wished to investigate whether the 3,4-diformyl system previously employed with pyridazine derivatives7 would in fact afford a stable, non-classical thienothiophene or whether aryl or EWGs were truly necessary for environmental stability. The attempted formation of the non-classical thiophene 3 was initially attempted on the dialdehyde 1 with harsher thiating conditions (P4S10, CS2 reflux). However, this only afforded decomposition of the substrate. Milder conditions (Lawesson’s reagent, benzene reflux) did not give the desired thienothiophene 3 as the major product. Instead, the thienothione 2 was isolated in good yield (79%) (Scheme 1). Thione 2 is structurally similar to the 5-substituted-thiophene-2(3H)-thiones formed by Commercon and Ponsinet using CS2 and alkynes or allenes.17 Analysis of the crude product mixture by GC/MS did show some formation of the desired compound 3. The mass spectra for this minor component did display an M+ of 168 m/z (molecular ion peak) and an isotopic pattern consistent with two sulfurs present ([M+ + 2] peak 9.0% relative abundance). However, this compound represented a very minor component (about 5%) of the product mixture and thus no further spectroscopic characterization could be obtained. Varying reaction conditions by
reducing the stoichiometries of Lawesson’s reagent employed did not affect the amount of thiophene 3 observed.
The major product, thiophene 2, was isolated via alumina chromatography as a cherry red solid. Compound 2 displays high air and solution stability in a wide variety of solvents. The structure of thione 2 was confirmed by IR, NMR, and GC/MS analysis. The 1H NMR of 2 displays the expected two methyl singlets at 2.33 and 2.72 ppm (3H) and the methylene singlet at 4.09 ppm (2H). In addition, the NMR spectrum shows the disappearance of the aldehyde singlet at 10.35 ppm. The 13C NMR shows a distinctive thione (C=S) signal at 211 ppm. Mass spectrometry also confirms the molecular weight of 2, with both the base and [M+] peaks at 200 m/z. The relative abundance of the [M+ + 2] peak (13.74%) matches well with the calculated isotopic abundance of three sulfur atoms present in the compound. IR spectroscopy also showed evidence of the typical aromatic C=C (1594 cm-1) stretches, with loss of the carbonyl stretch observed in 1.
The proposed mechanism for the formation of thione 2 involves first creation of the dithione (4) (Scheme 2). Nucleophilic attack at the thionyl carbon by the adjacent sulfur atom gives the ring closed intermediate 5. The key step in the thione 2 formation involves a 1,3-hydride shift across the newly formed thiophene ring. Although this type of rearrangement is rare, since most shifts of this nature involve the adjacent atomic positions, this 1,3-shift is not without precedent. Several instances of hydride migrations involving non-adjacent positions have been noted,18,19 including by Picaud and coworkers, who observed a 1,3-hydride shift across an substituted bisabolyl ring.20 Formation of the minor product 3 is likely to also occur through the ring closed intermediate 5, followed by loss of elemental sulfur. This mechanism is consistent with the previously proposed S-closure for other non-classical thiophene systems.21 We speculate that the low yield observed for the non-classical product
3 is from its inherent instability due to the lack of electron withdrawing substituents present. This is consistent with Cava’s assertion that only non-classical thiophenes with strong EWGs present will be stable and thus can isolated.12 Therefore, thiation in this particular case primarily affords the stable thienothione and not the non-classical product 3.
The structure of thione 2 was also confirmed by X-ray crystallographic analysis (Figure 2). Compound 2 crystallizes in the monoclinic P 21/n space group, with four molecules in the unit cell. Thione 2 exhibits whole-molecule disorder in the solid state, with major (84%) and minor (16%) orientations related by an approximate 2-fold rotation about an axis that bisects the molecule through the bonds formed between atoms C3-C4, C5-C6 and C2-S2. The thione bond lengths, C2-S2 and C2’-S2’ (for the disordered molecule) are 1.642 and 1.633 Å respectively, displaying their double bond character. Conversely, the longer S1-C1 and S1’-C1’ single bond lengths are 1.832 and 1.797 Å respectively. The C-C bonds within the thiophene portion of 2 display strong aromatic character, with the C4-C5 and C6-C7 bond lengths averaging to 1.368 Å. The central thienothiophene portion of 2 is highly planar, with the root-mean-square deviation from the plane created by C1, C2, C4-C7, S1, and S3 at 0.0164Å. The methyl substituents C3 and C8 are also nearly planar with respect to the central fused ring, with deviations from the least-squares plane of 0.003 Å above and 0.010 Å below the plane of the ring respectively. Likewise, the thione sulfur S2 is nearly planar with the central ring, with a deviation from the least-squares plane of 0.052 Å above the ring plane.
Tables of crystallographic details, atomic coordinates and displacement parameters, bond distances and angles, intermolecular contact distances, structure factors and a crystallographic information file (CIF) for the structure of 2 have been deposited with the Cambridge Crystallographic Data Centre.22 Crystal data and a summary of experimental details are given in Table 1. General procedures and experimental data for 2 can be found in the references and notes section.23
ACKNOWLEDGEMENTS
We wish to thank our sources of support for this research, including the Department of Chemistry, the Junior Faculty Summer Research Award from the College of Arts and Sciences and the Chemistry Diversity Initiative at Eastern Kentucky University. We also wish to thank Dr. Jay Baltisberger at Berea College in Berea, KY for his support on the NMR spectroscopy.
References
1. A. R. Katritzky and C. W. Rees, 'Comprehensive Heterocyclic Chemistry: structure, reactions, synthesis and uses of heterocyclic compounds,' Pergamon Press: Oxford, 1984.
2. J. Roncali, Chem. Rev., 1997, 97, 173. CrossRef
3. G. Heywang and F. Jonas, Adv. Mater., 1992, 4, 116. CrossRef
4. V. P. Litvinov, Russ. Chem. Rev., 2005, 74, 217. CrossRef
5. M. C. Gather, M. Heeny, W. Zhang, K. S. Whitehead, D. D. C. Bradley, I. McCulloch, and A. J. Campbell, Chem. Commun., 2008, 1079. CrossRef
6. M. He, J. Li, M. L. Sorensen, F. Zhang, R. R. Hancock, H. H. Fong, V. A. Pozdin, D. Smilgies, and G. G. Malliaras, J. Am. Chem. Soc., 2009, 131, 11930. CrossRef
7. N. C. Tice, S. M. Peak, and S. Parkin, Heterocycles, 2010, 81, 1631. CrossRef
8. A. Ishii, J. Nakayama, J. Kazami, Y. Ida, T. Nakamura, and M. Hoshino, J. Org. Chem., 1991, 56, 78. CrossRef
9. J. Nakayama, M. Machida, R. Saito, and M. Hoshino, Tetrahedron Lett., 1985, 26, 1983. CrossRef
10. J. Nakayama, A. Ishii, Y. Kobayashi, and M. Hoshino, J. Chem. Soc., Chem. Commun., 1988, 959. CrossRef
11. R. R. Amaresh, M. V. Lakshmikantham, R. Geng, and M. P. Cava, Tetrahedron Lett., 2000, 41, 8843. CrossRef
12. R. R. Amaresh, M. V. Lakshmikantham, J. W. Baldwin, M. P. Cava, R. M. Metzger, and R. D. Rogers, J. Org. Chem., 2002, 67, 2453. CrossRef
13. S. Yoneda, K. Ozaki, T. Inoue, A. Sugimoto, K. Yanagi, and M. Minobe, J. Am. Chem. Soc., 1985, 107, 5801. CrossRef
14. S. Yoneda, K. Ozaki, A. Tsubouchi, H. Kojima, and K. Yanagi, J. Heterocycl. Chem., 1988, 25, 559. CrossRef
15. N. Matsumura, Y. Yagyu, H. Tanaka, H. Inoue, K. Takada, M. Yasui, and F. Iwasaka, Chem. Lett., 1996, 421. CrossRef
16. N. Matsumura, H. Tanaka, Y. Yagyu, K. Mizuno, H. Inoue, K. Takada, M. Yasui, and F. Iwasaka, J. Org. Chem., 1998, 63, 163. CrossRef
17. A. Commercon and G. Ponsinet, Tetrahedron Lett., 1985, 26, 5131. CrossRef
18. E. G. Mazitova, D. V. Shornikoz, E. V. Klyuchareva, and E. A. Kantor, Russ. J. Org. Chem., 2004, 40, 785.
19. A. G. Cook, K. A. Switek, K. A. Cutler, and A. M. Witt, Lett. Org. Chem., 2004, 1, 1. CrossRef
20. S. Picaud, P. Mercke, X. He, O. Sterner, M. Brodelius, D. E. Cane, and P. E. Brodelius, Arch. Biochem. Biophys., 2006, 448, 150. CrossRef
21. M. P. Cava and M. I. Levinson, Tetrahedron, 1985, 41, 5061. CrossRef
22. CCDC 784095 contains the supplementary crystallographic data for this paper. These data can be obtained free of charge from The Cambridge Crystallographic Data Centre via www.ccdc.cam.ac.uk/data_request/cif.
23. General Procedures. All reactions were carried out using standard Schlenk techniques under a nitrogen atmosphere unless otherwise noted. NMR solvent CDCl3 (Aldrich) was used without further purification. Benzene, methylene chloride (CH2Cl2), diethyl ether (Et2O), Lawesson’s reagent, and alumina (Aldrich) were also used without further purification. Thiophene precursor 2,5-dimethylthiophene- 3,4-dicarboxaldehye (1) was prepared according to literature methods24 and its structural identity was confirmed by NMR and GC/MS.
1H and 13C NMR spectra were recorded on a JEOL-300 MHz NMR spectrometer at ca. 22 oC and were referenced to residual solvent peaks. All 13C NMR spectra were listed as decoupled. Infrared spectra were recorded on Spectrum One FT-IR Spectrometer. Electron ionization (EI) mass spectra were recorded at 70 eV on a Perkin Elmer GC/MS. Melting points were taken on a standard Mel-Temp apparatus. X-Ray diffraction data were collected at 90 K on a Bruker-Nonius X8 Proteum diffractometer at the University of Kentucky X-Ray Crystallographic Laboratories. Elemental analysis was performed at Atlantic Microlabs, Inc. in Norcross, GA.
Synthesis of 4,6-dimethylthieno[3,4-c]thiophene-1(3H)-thione (2). To a 100 mL round bottom flask, Lawesson’s reagent (300 mg, 0.74 mmol) was added to 40 mL of benzene. Then 1 (300 mg, 1.79 mmol) was added and the suspension was allowed to reflux for 8 h. Lawesson’s reagent (300 mg, 1.79 mmol) was again added and the suspension was allowed to reflux for an additional 8 h. The reaction mixture was cooled and then passed through a thick pad of neutral alumina with benzene. The volatiles were removed under reduced pressure to afford 2 (283 mg, 1.42 mmol, 79.1%) as a red solid. The molecular structure of 2 was confirmed by X-ray crystallographic methods. Mp 102.3-104.2 °C. 1H NMR (300 MHz, CDCl3, ppm): δ 2.33 (s, 3H, Me), 2.72 (s, 3H, Me), 4.09 (s, 2H, CH2) 13C NMR (75 MHz, CDCl3, ppm): δ 12.90 (CH3), 14.15 (CH3), 33.13 (CH2), 111.03 (C), 125.55 (C), 140.95 (C), 143.95 (C), 211.26 (CS) IR (KBr, cm-1): 2915 (C-H), 1594 (C=C). GC/MS(EI-pos): m/z 200 (M+ and base peak). Anal. Calcd for C8H8S3: C, 48.00; H, 4.02. Found: C, 48.83; H, 4.69.
The X-ray crystallographic structure of 2 was determined by X-ray crystallographic methods. The crystals for which data were collected were typical of others in the batch, which had been grown by slow evaporation from a 75:25 mixture of CH2Cl2: Et2O ether at room temperature. These crystals were mounted on glass fibers with polyisobutene oil. Data were collected at 90 K on a Bruker-Nonius X8 Proteum diffractometer. The main programs used were Bruker APEX2 to obtain cell parameters and for data reduction, SADABS for absorption correction,25 SHELXS-97 for structure solution, and SHELXL-97 for refinement.26 Hydrogen atoms were placed in geometrically calculated positions.
24. T. M. Brown, W. Carruthers, and M. G. Pellatt, J. Chem. Soc., Perkin Trans. I, 1982, 2, 483. CrossRef
25. Bruker, 2009. APEX2 and SADABS. Bruker AXS Inc., Madison, Wisconsin, USA.
26. G. M. Sheldrick, Acta Cryst., 2008, A64, 112.