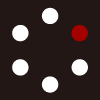
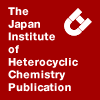
HETEROCYCLES
An International Journal for Reviews and Communications in Heterocyclic ChemistryWeb Edition ISSN: 1881-0942
Published online by The Japan Institute of Heterocyclic Chemistry
e-Journal
Full Text HTML
Received, 21st July, 2010, Accepted, 14th October, 2010, Published online, 19th October, 2010.
DOI: 10.3987/COM-10-12024
■ Non-CRET-Based Green Chemiluminescence of Imidazopyrazinone Modified by 2,3,6,7-Tetrahydro-1H,5H-benzo[i,j]quinolizine as a Strong Electron-Donating Unit
Ryota Saito*
Toho University, 2-2-1, Miyama, Funabashi, Chiba 274-8510, Japan
Abstract
A new imidazopyrazinone derivative, 1, having a 2,3,6,7-tetrahydro-1H,5H-benzo[i,j]quinolizin-9-yl (julolidin-9-yl) group at the 6-position, was synthesized and exhibited a largely red-shifted chemiluminescence in diglyme containing acetate buffer caused by a strong electron donation from the julolidin-9-yl group. The maximum wavelength was observed at 523 nm, which represents the most red-shifted light achieved only by the electron-donating effect from the 6-position of the imidazopyrazinone skeleton without any aids of extended π-conjugation systems or longer-wavelength-light emitting fluorophores.Imidazo[1,2-a]pyrazin-3(7H)-ones are known as chemiluminescent materials which can emit light as one of the products of its reaction with molecular oxygen (3O2).1 The chemiluminescent reactions of the imidazopyrazinones occur not only with 3O2 but also with reactive oxygen species such as O2•– and singlet oxygen (1O2).2 This property has attracted considerable attention from researchers because it is applicable to the detection of reactive oxygen species. Several imidazopyrazinone-based probes for reactive oxygen species have been thus developed during the last two decades.3–9 2-Methyl-6-(4-methoxyphenyl)imidazo[1,2-a]pyrazin-3(7H)-one (MCLA) has been widely recognized as the most popular probe used for detecting reactive oxygen species such as O2•– and 1O2 emitting visible light (λmax = 465 nm in buffers at physiological pH conditions) with no external light source for excitation, which enables the sensitive and real-time detection of O2•–.4 A fluorescein-linking MCLA derivative (FCLA), has been developed to detect O2•– at longer wavelengths than MCLA.5 Upon reacting with O2•– at physiological pH conditions, FCLA emits green light (λmax = 532 nm). This feature makes FCLA more useful than MCLA, because the blue light produced by MCLA is absorbed by some biomaterials.6 More recently, other imidazopyrazinone-based chemiluminescent probes, KBI and BCLA, have been developed. These possess intensely fluorescent borondipyrromethene (BODIPY) units directly connected to the central imidazopyrazinone.7 Both FCLA and the BODIPY-connected probes have been designed to emit greatly red-shifted light on the basis of chemiluminescent resonance energy transfer (CRET). The energy of the singlet-excited state generated by the reaction of the imidazopyrazinone moiety with 3O2 or the reactive oxygen species is transferred by Förester’s mechanism to the fluorophores, the fluorescein or BODIPY units, to emit the specific long-wavelength chemiluminescence. However, in some cases, the chemiluminescence intensities of CRET-based probes are lower than those observed for MCLA,8 which is probably caused by a decrease in the efficacy of the energy transfer step. Therefore, there is still a need to develop non-CRET-based chemiluminescent probes that are capable of emitting long-wavelength light directly from the corresponding singlet-excited-state products, i.e., amidopyrazines, formed during the oxidative chemiluminescent reactions.
The author previously synthesized imidazo[1,2-a]pyrazin-3(7H)-ones with two phenyl groups at the 6- and 8-positions of the imidazopyrazinone skeleton.9 Unfortunately, their chemiluminescence spectra measured under neutral conditions showed peaks at around 400 nm, while chemiluminescence maxima were exhibited at around 500–530 nm in basic buffer solutions. The author also reported that amidopyrazines, the products formed by the chemiluminescent reactions of imidazopyrazinones with oxygen, exhibited substituent-sensitive fluorescence, in which the fluorescence maxima were bathochromically shifted with an increase in the strength of the electron donation from R3.10 Based on this finding, it seems natural to consider the possibility that introducing a strong electron-donating substituent at the 6-position (R3) of the imidazopyrazinone skeleton might produce a largely red-shifted chemiluminescence. Thus, as a part of ongoing efforts to develop imidazopyrazinones capable of emitting long-wavelength light, a new imidazopyrazinone, 1, possessing the 2,3,6,7-tetrahydro-1H,5H-benzo[i,j]quinolizin-9-yl (julolidin-9-yl) group at the 6-position has been designed. The julolidin-9-yl group is known as a strong electron-donating group with largely negative Hammett’s substituent constant (σp = –0.89).11 Herein, the synthesis and chemiluminescent property of 1 are reported. The synthesis and fluorescence of 2 are also examined to confirm it is the light emitter produced during the chemiluminescence reaction of 1.
The synthetic routes for obtaining 1 and the corresponding light emitter, 2, are shown in Scheme 1. A palladium-catalyzed cross-coupling reaction of 9-bromojulolidine hydrochloride (3·HBr),12 which was prepared by the bromination of julolidine with pinacolborane, in the presence of Cyclohexyl JohnPhos as a ligand to afford the borate 4. The obtained 4 was condensed with 2-amino-5-bromopyrazine (5)7b,13 by a Suzuki coupling reaction to give aminopyrazine 6, which was then converted into 1 using the popular reaction condition for preparing the imidazo[1,2-a]pyrazin-3(7H)-one skeleton, involving the reaction of a precursor aminopyrazine with an α-ketoaldehyde or its synthetic equivalent in an alcohol in the presence of a catalytic amount of concentrated hydrochloric acid.14 Compound 2 was prepared by reacting 6 with acetic anhydride in the presence of N,N-dimethylaminopyridine as a base. In this reaction, imide 7 was obtained as a byproduct, which was successfully hydrolyzed to give 2. The structures of all of the synthesized compounds were assigned on the basis of 1H-NMR, IR, and mass data, as well as combustion analyses or a high-resolution mass spectrometric analysis.
Chemiluminescence of 1 in aerated dimethyl sulfoxide (DMSO), which has been commonly used for performing chemiluminescence measurements of imidazopyrazinones,7b,14b,15 was measured for the first thing. The chemiluminescence spectrum of 1 is shown in Figure 1, and the chemiluminescence maximum (CLmax) was observed at 483 nm being longer than that of MCLA under the same condition (Table 1). This CLmax of 1 was coincide with the fluorescence maximum (FLmax) of 2 in DMSO containing 1.0% of 1-M NaOMe/MeOH, while the FLmax of 2 in DMSO without additives was observed at 525 nm (Figure 1 and Table 1). This convinces us that the light emitter involved in the chemiluminescent reaction of 1 was the conjugate base form of 2 formed according to the mechanism shown in the left side of Scheme 2. The luminescence efficiency was roughly estimated, by comparing the luminescent intensity of MCLA and that of 1, to be about one-third of MCLA. Although the light yield from 1 was less than that from MCLA, it still higher than that obtained with CLA, a 6-phenyl derivative of MCLA known to exhibit eight-times less light yield than MCLA.16 It has been previously demonstrated that the chemiluminescence maxima of coelenterazine analogues (8a–d) are shifted bathochromically with an increase in the electron-donating ability of substituent R at the para position of the 6-phenyl group,13b and a linear correlation was obtained between the luminescence energy (in cm–1) and Hammett’s substituent constant (σp) of substituent R, as shown in Figure 2 (closed squares). When the observed emission energy for 1 was plotted in this figure (open square), all of the plots in Figure 2 still exhibited a straight correlation, confirming the reported Hammett’s substituent constant of the julolidine unit connecting at the para position of the nitrogen atom.
The FLmax of 2 in DMSO at 525 nm indicates that such a long-wavelength chemiluminescence could be observed under a condition that affords the singlet-excited state of 2 in non-deprotonated neutral form. Thus, the chemiluminescence of 1 was measured in diglyme containing 0.66-vol% of acetate buffer (pH = 5.6 at 25 °C), in which imidazopyrazinones have been known to predominantly exhibit emissions from singlet-exited amidopyrazines in neutral forms (Scheme 2, the right side).15a,17 As a result, CLmax was observed at 523 nm (Figure 3 and Table 1), and well coincided with the fluorescence of 2 measured under the same condition. This largely red-shifted chemiluminescence was apparently caused by the strong electron donation from the julolidine moiety toward the central pyrazine ring as observed with a coelenteramide analog possessing dimethylaminophenyl group.10 The luminescent intensity was about one-half as high as that for MCLA in DMSO, indicating 1 can be a good luminescent probe under this condition.
Thus, in the present study, a long-wavelength chemiluminescence of over 520 nm was produced in the imidazopyrazinone chemiluminescence system, not by using CRET or expanding its π-conjugate system, but only by introducing a strong electron donor at the para-position of 6-phenyl group. The present study provides a new principle as a guide for developing non-CRET-based long-wavelength chemiluminescent imidazopyrazinones.
EXPERIMENTAL
All melting points were measured on MEL-TEMP apparatus (LABORATORY DEVICES Inc, U.S.A.) in open capillary tubes; the values were uncorrected. 1H-NMR spectra were recorded on a JNM-ECP400 spectrometer (JEOL Ltd., Japan). Chemical shifts (δ) are reported in ppm and were measured using tetramethylsilane or an undeuterated solvent as an internal standard in the deuterated solvent used. Coupling constants (J) are given in Hz. Chemical shift multiplicities are reported as s = singlet, d = doublet, t = triplet, q = quartet, quint = quintet, and m = multiplet. Infrared (IR) spectra were obtained using FT/IR-4100, FT/IR-460plus, or FT/IR-660plus spectrophotometers (JASCO Co., Ltd., Japan). Fast-atom-bombardment (FAB) mass spectra were measured on a JMS-600-H mass spectrometer (JEOL Ltd., Japan). Xenon was used as a bombardment gas, and all the analyses were carried out in a positive mode with the ionization energy and accelerating voltage set at 70 eV and 3 kV, respectively. A mixture of dithiothreitol and α-thioglycerol (1:1 or 1:2) was used as a liquid matrix. High- and low-resolution electron impact (EI) mass spectra were obtained with a JMS-AM II 50 mass spectrometer (JEOL Co., Ltd., Japan). The ionization energy was 70 eV, and the accelerating voltages were 0.3 and 0.5 kV, respectively, for the low- and high-resolution analyses. Combustion analyses were performed on a MT-6 analyzer (Yanaco New Science Inc., Japan). Column chromatography was carried out on silica gel (particle size: 63–210 μm or 40–100 μm; Kanto Chemical Co.). Chemiluminescence and fluorescence spectra were collected with a JASCO F-777 Spectrofluorometer. For Chemiluminescence measurements, excitation light was shut off and only the light detector with the highest sensitivity was used to measure the emitted light; bandpass of the detector: 10 nm; scan speed: 1000 nm/min.
MCLA was purchased from Tokyo Chemical Industry Co., LTD. (Japan). Spectroscopic grade MeOH and DMSO were obtained from Kanto Chemical Co. Inc. (Japan). Commercially available diglyme was distilled prior to use. Other conventional chemicals used in the present study are commercially available and were used as received.
9-(4,4,5,5-Tetramethyl-1,3,2-dioxaborolan-2-yl)-2,3,6,7-1H,5H-benzo[i,j]quinolizine (4)
Under argon atmosphere, to a solution of 312 hydrobromide (602 mg, 1.81 mmol) in 1,4-dioxane (dehydrated, 10 mL) was added triethylamine (1.8 mL, 12.8 mmol), Pd(OAc)2 (27.7 mg, 123 µmol, 7 mol%), 2-dicyclohexylphosphinobiphenyl (126 mg, 358 µmol, 20 mol%), and pinacolborane (0.81 mL, 5.41 mmol, 3 equiv). The mixture was stirred to 80 ºC for 3 h. After removal of the solvent, water was added to the reaction mixture and organic materials were extracted with EtOAc/hexane (1/1, v/v). The organic layer was washed with brine, and dried over Na2SO4. After evaporation of the solvent, the obtained crude product was purified by column chromatography on silica gel (46–50 µm, 48 g) with EtOAc/hexane (1/20, v/v) as eluents to give 4 as yellow solids (466 mg, 86%); mp 76.0–76.5 ºC (decomp); 1H-NMR (400 MHz, CDCl3) δ/ppm 7.25 (s, 2H, phenyl-2’,6’-H), 3.19–3.16 (m, 4H, -NCH2CH2-), 2.74 (t, J = 6.2 Hz, 4H, -CH2CH2CH2N-), 1.97–1.91 (m, 4H, -CH2CH2CH2-), 1.31 (s, 12H, CH3). IR (KBr) νmax/cm–1 2976, 2932, 2840 (νC–H), 1235 (νC–N); MS (FAB+, DTT/TG = 1/1) m/z 300 [M+H]+. HR-MS (positive EI) Calcd for C18H26BNO2: 299.2057. Found: 299.2057.
2-Amino-5-(2,3,6,7-tetrahydro-1H,5H-benzo[i,j]quinolizin-9-yl)pyrazine (6)
Tetrakis(triphenylphosphine)palladium (38.0 mg, 32.9 µmol, 3 mol%), 5 7b,13 (183 mg, 1.05 mmol), and 4 (375 mg, 1.25 mmol) were successively dissolved in 1,4-dioxane (5 mL) under argon atmosphere. To the solution was added 2M Na2CO3 (1.0 mL, 2.00 mmol), and the mixture was refluxed for 29 h. After cooling to room temperature, water was added to the reaction mixture, and organic materials were extracted with CHCl3. The organic layer was washed with brine, and dried over Na2SO4. After evaporation of the solvent, the obtained crude product was purified by column chromatography on silica gel (46–50 µm, 25 g) with hexane/EtOAc (10/1, v/v) as eluents, followed by recrystallization from ethanol to give 7 as a greenish solid (82.3 mg, 29%); mp 165.0–166.0 ºC; 1H-NMR (400 MHz, CDCl3) δ/ppm 8.33 (d, J = 1.4 Hz, 1H, pyrazine-3’-H), 7.99 (d, J = 1.4 Hz, 1H, pyrazine-6’-H), 7.31 (s, 2H, phenyl-2’,6’-H), 4.42 (s, 2H, NH2), 3.18 (t, J = 5.5 Hz, 4H, -NCH2CH2-), 2.82 (t, J = 6.2 Hz, 4H, -CH2CH2CH2N-), 2.02–1.96 (m, 4H, -CH2CH2CH2-); IR (KBr) νmax/cm–1 3432, 3306 (νN–H), 3172, 2934, 2830 (νC–H); MS (FAB+, DTT/TG = 1/1) m/z 267 [M+H]+. HR-MS (positive EI) Calcd for C16H18N4: 266.1531. Found: 266.1528.
2-Methyl-6-(2,3,6,7-tetrahydro-1H,5H-benzo[i,j]quinolizin-9-yl)imidazo[1,2-a]pyrazin-3(7H)-one (1)
A solution of aminopyrazine hydrochloride (46.0 mg, 0.152 mmol) and pyruvaldehyde dimethyl acetal (48.0 µL, 0.406 mmol) in water (0.9 mL) and 1,4-dioxane (2.5 mL) was stirred at 80 ºC under argon for 240 h. After evaporation of the solvent, the obtained materials were purified by preparative TLC to afford the aimed compound as orange solids. (5.6 mg, 12%); mp >360 ºC; 1H-NMR (MeOH-d4, 400 MHz) δ/ppm 7.79 (s, 1H), 7.49 (s, 1H), 7.44 (s, 1H), 7.02 (s, 2H), 3.21 (t, J = 5.5 Hz, 4H), 2.77 (t, J = 6.2 Hz, 4H), 2.42 (s, 3H), 1.99–1.93 (m, 4H); MS (FAB+, DTT:TG = 1:1) m/z 321 [M+H]+. HR-MS (positive EI) Calcd for C19H20N4O: 320.1637. Found: 320.1635.
2-Acetamido-5-(2,3,6,7-tetrahydro-1H,5H-benzo[i,j]quinolizin-9-yl)pyrazine (2)
Under argon atmosphere, a mixture of (101 mg, 379 µmol), N,N-dimethylaminopyridine (3.8 mL) and acetic anhydride (0.194 mL, 2.05 mmol) in pyridine was stirred at 50 ºC for 5.5 h. After removal of the solvent, water was added to the reaction mixture, and organic materials were extracted with CHCl3. The organic layer was washed with brine, and dried over Na2SO4. After evaporation of the solvent, the obtained crude product was purified by column chromatography on silica gel (46–50 µm, 25 g) with CHCl3/EtOAc (1/1, v/v) as eluents, followed by recrystallization from Ethanol to give 2 as a yellow solid (33.4 mg, 29%); mp. 207–208 ºC (decomp); 1H-NMR (400 MHz, CDCl3) δ/ppm 9.43 (s, 1H, NH), 8.53 (d, J = 1.1 Hz, 1H, pyrazine-3’-H), 7.76 (d, J = 1.1 Hz, 1H, pyrazine-6’-H), 7.43 (s, 2H, phenyl-2’,6’-H), 3.22 (t, J = 5.5 Hz, 2H, -NCH2CH2-), 2.82 (t, J = 6.2 Hz, 4H, -CH2CH2CH2N-), 2.02–1.96 (m, 4H, -CH2CH2CH2-); IR (KBr) νmax/cm–1 3255, 3219 (νN–H), 3131, 3078, 3030, 2931, 2836 (νC–H), 1698 (νC=O), 1352, 1316 (νC-N); MS (FAB+, DTT/TG = 1/1) m/z 309 [M+H]+. HR-MS (positive EI) Calcd for C18H20N4O: 308.1637. Found: 308.1639.
Hydrolysis of N,N-diasetamido-5-(2,3,6,7-tetrahydro-1H,5H-benzo[i,j]quinolizin-9-yl)pyrazine (7)
To a solution of 7 (79.6 mg, 227 µmol) in MeOH-H2O (9/1, v/v, 5 mL) was added 5M NaOH (0.05 mL, 250 µmol) under argon atmosphere, and the reaction mixture was stirred at room temperature for 1 h. After removal of the solvent, water was added to the reaction mixture, and organic materials were extracted with CHCl3. The organic layer was washed with brine, and dried over Na2SO4. After evaporation of the solvent, the obtained crude product was purified by column chromatography on silica gel (46–50 µm, 16 g) with CHCl3/EtOAc (1/1, v/v) as eluents, followed by recrystallization from ethanol to give 2 as yellow solids (22.9 mg, 33%).
Chemiluminescence reactions
Chemiluminescent reactions were achieved by mixing 1.0-mM solution of an imidazopyrazinone in MeOH (100 μL) and 2.0-mL of DMSO or diglyme containing 0.66-vol% of 0.2-M acetate buffer (pH = 5.6 at 25 °C) in a quartz cuvette (path length: 1 cm) at 25 °C.
Fluorescence measurements
A solution of 2 for fluorescence measurement was prepared by mixing a stock solution (100 µL) of 1.0 mM 2 in DMSO and 2.0 mL of DMSO containing 1.0-vol% of 1-M NaOMe/MeOH or diglyme containing 0.66-vol% of 0.2-M acetate buffer (pH = 5.6 at 25 °C) within a quartz cuvette (path length: 1 cm) at room temperature.
ACKNOWLEDGEMENTS
This work was financially supported by “High-Tech Research Center” Project for Private Universities: matching fund subsidy from the Ministry of Education, Culture, Sports, Science, and Technology (2005–2009).
References
1. (a) T. Goto, Pure Apll. Chem., 1968, 17, 421; CrossRef (b) F. McCapra and M. J. Manning, J. Chem. Soc., Chem. Commun., 1973, 467; CrossRef (c) K. Hori, J. E. Wampler, and M. J. Cormier, J. Chem. Soc., Chem. Commun., 1973, 492; CrossRef (d) K. Teranishi and T. Goto, Chem. Lett., 1989, 18, 1423. CrossRef
2. (a) T. Goto and T. Takagi, Bull. Chem. Soc. Jpn., 1980, 53, 833; CrossRef (b) S. Mashiko, N. Suzuki, S. Koga, M. Nakano, T. Goto, T. Ashino, I. Mizumoto, and H. Inaba, J. Biolumin. Chemilumin., 1991, 6, 69. CrossRef
3. (a) K. Teranishi and O. Shimomura, Anal. Biochem., 1997, 249, 37; CrossRef (b) O. Shimomura, C. Wu, A. Murai, and H. Nakamura, Anal. Biochem., 1998, 258, 230. CrossRef
4. M. Nakano, M. Kikuyama, T. Hasegawa, T. Ito, K. Sakurai, K. Hiraishi, E. Hashimura, and M. Adachi, FEBS Lett., 1995, 372, 140. CrossRef
5. N. Suzuki, K. Suetsuna, S. Mashiko, B. Yoda, T. Nomoto, Y. Toya, H. Inaba, and T. Goto, Agric. Biol. Chem., 1991, 55, 157. CrossRef
6. J. Wang, D. Xing, Y. He, and X. Hu, FEBS Lett., 2002, 523, 128. CrossRef
7. (a) M. Sekiya, K. Umezawa, A. Sato, D. Citterio, and K. Suzuki, Chem. Commun., 2009, 3047; CrossRef (b) R. Saito, A. Ohno, and E. Ito, Tetrahedron, 2010, 66, 583. CrossRef
8. K. Teranishi and T. Nishiguchi, Anal. Biochem., 2004, 325, 185. CrossRef
9. (a) R. Saito, C. Inoue, and A. Katoh, Heterocycles, 2004, 63, 759; CrossRef (b) R. Saito, N. Suga, A. Katoh, S. Maki, T. Hirano, and H. Niwa, ‘Proceedings of the 13th International Symposium on Bioluminescence & Chemiluminescence. Progress and Perspectives,’ ed. by A. Tsuji, M. Matsumono, M. Maeda, L. J. Kricka, and P. E. Stanley, World Scientific, Singapore, 2005, pp. 335–338.
10. R. Saito, T. Hirano, H. Niwa, and M. Ohashi, J. Chem. Soc., Perkin Trans. 2, 1997, 1711. CrossRef
11. A. Zakrzewska, R. Gawinecki, E. Kolehmainen, and B. Osmaialowski, Int. J. Mol. Sci., 2005, 6, 52. CrossRef
12. (a) H. Wang, Z. Lu, S. J. Lord, K. A. Willets, J. A. Bertke, S. D. Bunge, W. E. Moerner, and R. J. Tweig, Tetrahedron, 2007, 63, 103; CrossRef (b) H. Katayama, M. Ohkoshi, and K. Kaneko, Chem. Pharm. Bull., 1984, 32, 1770. CrossRef
13. T. Itoh, S. Kato, N. Nonoyama, T. Wada, K. Maeda, and T. Mase, Org. Process. Res. Dev., 2006, 10, 822. CrossRef
14. (a) O. Shimomura, B. Musicki, and Y. Kishi, Biochem. J., 1989, 261, 913; (b) R. Saito, T. Hirano, H. Niwa, and M. Ohashi, Chem. Lett., 1998, 27, 95; CrossRef (c) H. Nakamura, M. Aizawa, D. Takeuchi, A. Murai, and O. Shimomura, Tetrahedron Lett., 2000, 41, 2185. CrossRef
15. (a) T. Goto, S. Inoue, S. Sugiura, K. Nishikawa, M. Isobe, and Y. Abe, Tetrahedron Lett., 1968, 9, 4035; CrossRef (b) K. Teranishi and T. Goto, Bull. Chem. Soc. Jpn., 1990, 63, 3132; CrossRef (c) H. Kondo, T. Igarashi, S. Maki, H. Niwa, H. Ikeda, and T. Hirano, Tetrahedron Lett., 2005, 46, 7701; CrossRef (d) Y. Takahashi, H. Kondo, S. Maki, H. Niwa, H. Ikeda, and T. Hirano, Tetrahedron Lett., 2006, 47, 6057; CrossRef (e) T. Hirano, Y. Takahashi, H. Kondo, S. Maki, S. Kojima, H. Ikeda, and H. Niwa, Photochem. Photobiol. Sci., 2008, 7, 197. CrossRef
16. Y. Toya, T. Kayano, K. Sato, and T. Goto, Bull. Chem. Soc. Jpn., 1992, 65, 2475. CrossRef
17. (a) T. Hirano, Y. Gomi, T. Takahashi, K. Kitahara, F. Q. Chen, I. Mizoguchi, S. Kyushin, and M. Ohashi, Tetrahedron Lett., 1992, 33, 5771; CrossRef (b) K. Fujimori, H. Nakajima, K. Akutsu, M. Mitani, H. Sawada, and M. Nakayama, J. Chem. Soc., Perkin Trans. 2, 1993, 2405. CrossRef