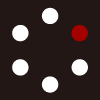
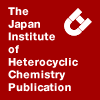
HETEROCYCLES
An International Journal for Reviews and Communications in Heterocyclic ChemistryWeb Edition ISSN: 1881-0942
Published online by The Japan Institute of Heterocyclic Chemistry
e-Journal
Full Text HTML
Received, 22nd July, 2010, Accepted, 11th November, 2010, Published online, 19th November, 2010.
DOI: 10.3987/COM-10-12026
■ Synthesis of Novel 5-(Pyrimidin-5-yl)-1,2,4-oxadiazole Derivatives via a Three-Component Cycloaddition and Subsequent Oxidative Dehydrogenation
Tong-Hui Huang, Hai-Yang Tu,* Ming Liu, and Ai-Dong Zhang*
Key Laboratory of Pesticide and Chemical Biology, Ministry of Education, College of Chemistry, Central China Normal University, Wuhan 430079, China
Abstract
A new and efficient strategy has been developed for the synthesis of novel 5-(pyrimidin-5-yl)-1,2,4-oxadiazoles with a wide diversity in substituents via Biginelli cycloaddition and subsequent oxidative dehydrogenation.Pyrimidinyl oxadiazole derivatives are of great interest because of their versatile biological activities in medicinal chemistry and pesticide chemistry.1-6 The main type of pyrimidinyl oxadiazoles are 5-(pyrimidin-5-yl)-1,3,4-oxadiazoles, which are conveniently synthesized through the cycloaddition of a hydrazide and a carboxylic acid, either with one pyrimidine moiety. In our early work, we have rationally identified one pyrimidinyl oxadiazole derivative, namely 3-aryl-5-(pyrimidin-5-yl)-1,2,4-oxadiazole, as an potential inhibitor toward the herbicidal target acetyl coenzyme A carboxylase. The potential biological activity of this class of compounds attracts us to achieve the synthesis of the novel 5-(pyrimidin-5-yl)-1,2,4-oxadiazole derivatives. The reported methods for synthesis of 1,2,4-oxadiazoles via the condensation of amidoxime and acyl cholide7 (or carboxylic acid8), either with one pyrimidine moiety, cannot be simply employed for 5-(pyrimidin-5-yl)-1,2,4-oxadiazoles, because of the harsh conditions and low yields as encountered in our attempt. Narrow diversity in substituents on pyrimidine ring compatible with the reactants is another great limit in the condensation.
Herein, we report a convenient and efficient approach for the synthesis of 5-(pyrimidin-5-yl)-1,2,4-oxadiazoles by using a three-component cycloaddition of 5-acetonyl-1,2,4-oxadiazole, urea and aldehyde, and subsequent oxidative dehydrogenation as shown in Scheme 1. The oxidative dehydrogenation has been modified to be mild enough and compatible with the heterocycle 1,2,4-oxadiazole. It is expected the highly efficiency of the classical Biginelli-type three-component condensation provides a great advantage for the synthesis of 5-(pyrimidin-5-yl)-1,2,4-oxadiazole derivatives with a wide diversity in substituents.
The building blocks of 5-acetonyl-3-substituted-1,2,4-oxadiazoles 2a–g were obtained by the reaction of substituted amidoximes 1 with acetoacetate according to the reported method9 with some modification by employing iso-butyl acetoacetate instead of tert-butyl ester. iso-Butyl acetoacetate is less sterically hindered that facilitates the solvent-free cycloaddtion at a relatively low temperature.
The Biginelli dihydropyrimidines can be viewed as the precursors of multiply substituted pyrimidines.10 In our synthesis, the precursors 5-(3,4-dihydro-2(1H)-pyrimidinon-5-yl)-1,2,4-oxadiazoles 4a–g were efficiently generated via the Biginelli-type condensation of building blocks 2a (5 mmol), urea (6 mmol), and aldehydes 3 (5 mmol) in the presence of TMSCl (5 mmol) in DMF/MeCN (2.5 mL/5.0 mL).11 Thus the desired products 5-(2-pyrimidinol-5-yl)-1,2,4-oxadiazoles 5a–g were possible to be obtained through a simple oxidative dehydrogenation of 4a–g, respectively.
However, the dehydrogenation of dihydropyrimidines is known to be nontrivial in synthesis of pyrimidines.12 It usually occurs in presence of an assistant agent including HNO3,13 DDQ,14 CAN15 or by photocatalytic oxidation.16 Recently, Yamamoto and coworkers developed a mild and practical procedure for the oxidative dehydrogenation with 65% aqueous tert-butyl hydroperoxide (TBHP) in the presence of catalytic amounts of a Cu salt and K2CO3 in dichloromethane.17 We have tried the reaction in several manners including the use of H2O2, DDQ and CAN (Table 1, entries 2, 3 and 4) as oxidants in the synthesis of 5, however, low conversion rates were obtained. We also attempted the Yamamoto’s method,17 only low conversion of 5-(3,4-dihydro-2(1H)-pyrimidinon-5-yl)-1,2,4-oxadiazoles 4 was achieved even in a lengthened reaction time (96 h, entry 1).
The proposed mechanism for transforming dihydropyrimidinones to the corresponding pyrimidines may stem from a process of Cu salt-assisted oxidative dehydrogenation17 as shown in Scheme 2. The different solubilities of DCM, toluene and 1,2-dichloropropane (1,2-DCP) to the reactants might contribute the observed low conversion ratios (entries 1, 5 and 6). On the other hand, water from the oxidant TBHP (65% in water) might result in Cu salt and inorganic base to be mostly dissolved in the aqueous phase. The two-phase reaction is certainly not favorable for the process. A phase transfer catalyst (PTC) would be helpful to promote oxidative dehydrogenation. Tetrabutylammonium bromide (TBAB) has been reported as PTC in cupper ion catalyzed oxidation reactions of alcohols to ketones.18 Thus TBAB in catalytic amount (10 mol%) was attempted to accelerate the reaction. Good yields in greatly shortened reaction times were obtained (entries 7, 8). The remaining hydroxyl group on pyrimidine ring can be further chlorinated with phosphoryl chloride and subsequently substituted with various nucleophiles.
In conclusion, an efficient and versatile approach has been proposed for the synthesis of substituted 5-(pyrimidin-5-yl)-1,2,4-oxadiazoles. Development of the oxidative dehydrogenation of the Biginelli dihydropyrimidines provides a promising and general method for the synthesis of pyrimidine derivatives. Moreover, taking use of Biginelli dihydropyrimidines as the precursors of pyrimidines and the remaining hydroxyl group on the resulted pyrimidine ring for further derivatization renders the synthetic route versatile in the synthesis of 5-(2-pyrimidinol-5-yl)-1,2,4-oxadiazoles with a wide diversity in substituents.
EXPERIMENTAL
All melting points were determined on a Buchi B-545 melting point apparatus and uncorrected. MS spectra were measured on a Finnigan Trace MS spectrometer. IR spectra were recorded on a PE-983 infrared spectrometer as KBr pellets with absorption in cm-1. NMR spectra were recorded in CDCl3 or DMSO-d6 on a Varian Mercury 600 spectrometer and resonances relative to TMS. Elementary analyses were taken on a Vario EL III elementary analysis instrument. All 5-acetonyl-3-substituted-1,2,4-oxadiazoles 2a–g were synthesized according to the reported method9 and their structures have been confirmed.
General procedure for the preparation of 5-(3,4-dihydro-2(1H)-pyrimidinon-5-yl)-1,2,4-oxadiazoles (4a–k). 5-Acetonyl-3-phenyl-1,2,4-oxadiazole 2 (1.0 g, 5 mmol), aldehyde 3 (5 mmol), urea (0.3 g, 6 mmol), and DMF/MeCN (2.5 mL/5.0 mL) were mixed in a 25-mL flask and TMSCl (0.54 g,5 mmol) was added dropwise at room temperature. The resulting mixture was stirred at room temperature for 1–4 h and precipitation was observed. The products 4 were isolated by filtering through a Buechner funnel and washed with water followed by EtOH, and then dried to give the crystalline powder product. Yields, melting points and spectroscopic data for selected 5-(3,4-dihydro-2(1H)-pyrimidinon-5-yl)-1,2,4- oxadiazoles are listed as follows.
5-(6-Methyl-4-propyl-3,4-dihydro-2(1H)-pyrimidinon-5-yl)-3-phenyl-1,2,4-oxadiazole (4a). White solid; yield: 88%; mp 247–248 °C; IR (KBr) ν: 3242, 1709, 1656, 1254 cm-1; 1H NMR (600 MHz, DMSO-d6): δ 0.88 (t, J = 7.2 Hz, 3H, Pr-CH3), 2.50–1.29 (m, 4H, Pr-CH2CH2), 2.38 (s, 3H, 6-CH3), 4.43–4.37 (m, 1H, 4-CH), 7.57–7.54 (m, 3H, m-H + p-H), 7.58 (s, 1H, 3-NH), 8.00 (d, J = 7.2 Hz, 2H, o-H), 9.37 (s, 1H, 1-NH); 13C NMR (150 MHz, DMSO-d6): δ 13.7, 16.8, 17.9, 38.8, 50.5, 93.8, 126.6, 126.9, 129.1, 131.2, 146.7, 152.4, 167.0, 174.9; MS (EI, 70 eV): m/z (%) = 299 (M+ + 1, 30), 240 (100), 195 (62), 117 (46); Anal. Calcd for C16H18N4O2 (%): C, 64.41; H, 6.08; N, 18.78. Found: C, 64.18; H, 6.19; N, 18.52.
5-(6-Methyl-4-phenyl-3,4-dihydro-2(1H)-pyrimidinon-5-yl)-3-phenyl-1,2,4-oxadiazole (4b). White solid; yield: 94%; mp 284–285 °C; IR (KBr) ν: 3242, 3111, 1706, 1645, 1249 cm-1; 1H NMR (600 MHz, DMSO-d6): δ 2.49 (s, 3H, 6-CH3), 5.46 (s, 1H, 4-CH), 7.55–7.24 (m, 8H, Ar-H {R2} + m-H + p-H {R1}), 7.96 (d, J = 6.8 Hz, 2H, o-H {R1}), 8.03 (s, 1H, 3-NH), 9.66 (s, 1H, 1-NH); 13C NMR (150 MHz, DMSO-d6): δ 18.1, 54.5, 93.8, 126.5, 126.5, 126.9, 127.7, 128.7, 129.1, 131.3, 143.9, 147.0, 151.8, 167.0, 174.8; MS (EI, 70 eV): m/z (%) = 332 (M+, 19), 274 (56), 255 (100), 178 (42); Anal. Calcd for C19H16N4O2 (%): C, 68.66; H, 4.85; N, 16.86. Found: C, 68.52; H, 4.63; N, 17.02.
5-(6-Methyl-4-(3-nitrophenyl)-3,4-dihydro-2(1H)-pyrimidinon-5-yl)-3-phenyl-1,2,4-oxadiazole (4d). Slight yellow solid; yield: 87%; mp 284–286 °C; IR (KBr) ν: 3238, 1711, 1657, 1255 cm-1; 1H NMR (600 MHz, DMSO-d6): δ 2.50 (s, 3H, 6-CH3), 5.65 (d, J = 2.4 Hz, 1H, 4-CH), 7.57–7.53 (m, 3H, m-H + p-H {R1}), 7.84–7.66 (m, 2H, Ar-H {R2}), 7.97 (d, J = 7.2 Hz, 2H, o-H {R1}), 8.16–8.14 (m, 2H, Ar-H {R2}), 8.27 (s, 1H, 3-NH), 9.79 (s, 1H, 1-NH); 13C NMR (150 MHz, DMSO-d6): δ 18.1, 54.0, 92.8, 121.3, 122.7, 126.4, 126.9, 129.1, 130.4, 131.3, 133.1, 145.9, 147.7, 147.8, 151.4, 166.9, 174.5; MS (EI, 70 eV): m/z (%) = 377 (M+, 21), 319 (67), 255 (100), 219 (49); Anal. Calcd for C19H15N5O4 (%): C, 60.47; H, 4.01; N, 18.56. Found: C, 60.19; H, 4.06; N, 18.30.
5-(4-(4-Methoxyphenyl)-6-methyl-3,4-dihydro-2(1H)-pyrimidinon-5-yl)-3-phenyl-1,2,4-oxadiazole (4g). White solid; yield: 95%; mp 235–237 °C; IR (KBr) ν: 3321, 1695, 1658, 1251 cm-1; 1H NMR (600 MHz, DMSO-d6): δ 2.47 (s, 3H, 6-CH3), 3.70 (s, 3H, OCH3), 5.40 (d, J = 2.4 Hz, 1H, 4-CH), 6.89 (d, J = 7.8 Hz, 2H, m-H {R2}), 7.27 (d, J = 7.8 Hz, 2H, o-H {R2}), 7.55–7.54 (m, 3H, m-H + p-H {R1}), 7.93 (s, 1H, 3-NH), 7.96 (d, J = 6.6 Hz, 2H, o-H {R1}), 9.59 (s, 1H, 1-NH); 13C NMR (150 MHz, DMSO-d6): δ 18.0, 53.8, 55.6, 94.0, 113.9, 126.5, 126.9, 127.6, 129.1, 131.3, 136.0, 146.6, 151.6, 158.7, 166.9, 174.8; MS (EI, 70 eV): m/z (%) = 362 (M+, 42), 285 (100), 255 (73); Anal. Calcd for C20H18N4O3 (%): C, 66.29; H, 5.01; N, 15.46. Found: C, 66.12; H, 5.17; N, 15.38.
General procedure for the preparation of 5-(2-pyrimidinol-5-yl)-1,2,4-oxadiazoles (5a–k). 5-(3,4-Dihydro-2(1H)-pyrimidinon-5-yl)-1,2,4-oxadiazoles 4 (5 mmol, 1 equiv), CuCl2 (0.03 g, 0.25 mmol, 5 mol%), Cs2CO3 (0.8 g, 2.5 mmol, 0.5 equiv), tetrabutylammonium bromide (0.16 g, 0.5 mmol, 10 mol%) and 1,2-dichloropropane (50 mL) were mixed in a 100-mL flask. The suspension was heated to 90 °C and treated with tert-butylhydroperoxide (65% in water) (3.5 g, 25mmol, 5.0 equiv) over 60 min with vigorous agitation. After 4–6 h, TLC indicated the consumption of the starting material. The solution was cooled to room temperature and washed with water. The two phases were separated and the organic phase was concentrated to the minimum agitation volume via reduced pressure distillation. The residue was purified by flash column chromatography (eluent: petroleum ether/EtOAc, 1:2) to afford the product 5 as a white or slight yellow powder. Yields, melting points and spectroscopic data for selected 5-(2-pyrimidinol-5-yl)-1,2,4-oxadiazoles are listed as follows.
5-(4-Methyl-6-propyl-2-pyrimidinol-5-yl)-3-phenyl-1,2,4-oxadiazole (5a). White solid; yield: 91%; mp 198–199 °C; IR (KBr) ν: 3433, 2959, 1660, 1608, 702 cm-1; 1H NMR (600 MHz, DMSO-d6): δ 0.97 (t, J = 7.2 Hz , 3H, Pr-CH3), 1.71–1.69 (m, 2H, CH2), 2.46 (s, 3H, 6-CH3), 2.72 (t, J = 7.2 Hz , 2H, CH2), 7.58–7.61 (m, 3H, m-H + p-H), 8.08 (d, J = 6.6 Hz, 2H, o-H), 12.55 (s, 1H, 2-OH); 13C NMR (150 MHz, DMSO-d6): δ 13.7, 16.9, 17.9, 50.56, 126.0, 126.9, 129.0, 131.6, 146.7, 152.4, 167.0, 167.6, 173.1, 174.9; MS (EI, 70 eV): m/z (%) = 297 (M+ + 1, 23), 267 (81), 236 (42), 219 (100), 159 (45); Anal. Calcd for C16H16N4O2 (%): C, 64.85; H, 5.44; N, 18.91. Found: C, 64.39; H, 5.21; N, 19.02.
5-(4-Methyl-6-phenyl-2-pyrimidinol-5-yl)-3-phenyl-1,2,4-oxadiazole (5b). Slight yellow solid; yield: 90%; mp 251–253 °C. IR (KBr) ν: 3432, 2910, 1649, 1606, 707 cm-1. 1H NMR (600 MHz, DMSO-d6): δ = 2.50 (s, 3 H, 6-CH3), 7.55–7.26 (m, 8 H, Ar-H {R2} + m-H + p-H {R1}), 7.97 (d, J = 7.6 Hz, 2 H, o-H {R1}), 13.95 (s, 1 H, 2-OH). 13C NMR (150 MHz, DMSO-d6): δ = 14.08, 100.6, 126.5, 127.2, 127.4, 127.6, 127.7, 127.9, 128.3, 128.6, 128.8, 157.9, 167.7, 170.2, 173.3. MS (EI, 70 eV): m/z (%) = 330 (M+, 34), 253 (39), 176 (100), 145 (52). Anal. Calcd for C19H14N4O2 (%): C, 69.08; H, 4.27; N, 16.96. Found: C, 69.49; H, 4.16; N, 16.72.
5-(4-Methyl-6-(3-nitrophenyl)-2-pyrimidinol-5-yl)-3-phenyl-1,2,4-oxadiazole (5d). Slight yellow solid; yield: 85%; mp 261–262 °C; IR (KBr) ν: 3441, 2922, 1663, 1663, 698 cm-1; 1H NMR (600 MHz, DMSO-d6): δ 2.73 (s, 3H, 6-CH3), 7.61–7.55 (m, 3H, m-H + p-H {R1}), 7.76–7.65 (m, 2H, Ar-H {R2}), 7.94 (d, J = 7.2 Hz, 2H, o-H {R1}), 8.33–8.23 (m, 2H, Ar-H {R2}), 12.99 (s, 1H, 2-OH); 13C NMR (150 MHz, DMSO-d6): δ 18.9, 109.1, 122.8, 125.0, 125.8, 127.0, 129.3, 130.1, 131.8, 134.3, 139.0, 147.5, 155.1, 164.5, 167.7, 170.2, 172.8; MS (EI, 70 eV): m/z (%) = 375 (M+, 22), 329 (61), 298 (100), 266 (43); Anal. Calcd for C19H13N5O4 (%): C, 60.80; H, 3.49; N, 18.66. Found: C, 60.52; H, 3.69; N, 18.51.
5-(6-(4-Methoxyphenyl)-4-methyl-2-pyrimidinol-5-yl)-3-phenyl-1,2,4-oxadiazole (5g). White solid; yield: 90%; mp 236–237 °C; IR (KBr) ν: 3432, 2839, 1643, 1579, 704 cm-1; 1H NMR (600 MHz, DMSO-d6): δ 2.42 (s, 3H, 6-CH3), 3.70 (s, 3H, OCH3), 6.88 (d, J = 7.2 Hz, 2H, m-H {R2}), 7.26 (d, J = 7.2 Hz, 2H, o-H {R2}), 7.54–7.51 (m, 3H, m-H + p-H {R1}), 7.97 (d, J = 6.6 Hz, 2H, o-H {R1}), 12.65 (s, 1H, 2-OH); 13C NMR (150 MHz, DMSO-d6): δ 14.0, 59.7, 101.6, 123.1, 126.5, 127.0, 127.3, 127.9, 128.3, 129.1, 129.7, 129.8, 130.2, 141.6, 167.6, 173.1; MS (EI, 70 eV): m/z (%) = 361 (M+ + 1, 12), 257 (58), 243 (100), 176 (43); Anal. Calcd for C20H16N4O3 (%): C, 66.66; H, 4.48; N, 15.55. Found: C, 66.42; H, 4.53; N, 15.91.
ACKNOWLEDGEMENTS
Financial supports of this research by the National Natural Science Foundation of China (Nos. 20972056, 20672044) and the PCSIRT (No. IRT0953) are greatly acknowledged.
References
1. G. Sonia, K. P. Manof, R. C. Acharjee, and K. R. Thengungal, Acta Pharm., 2008, 58, 119. CrossRef
2. K. G. Estep, K. A. Josef, E. R. Bacon, and P. M. Carabateas, J. Med. Chem., 1995, 38, 2582. CrossRef
3. P. G. Dunbar, G. J. Durant, F. Zheng, and Y. F. Abuh, J. Med. Chem., 1993, 36, 842. CrossRef
4. H. Ishida, S. Isami, T. Matsumura, and J. Kajita, Bioorg. Med. Chem. Lett., 2008, 18, 5472. CrossRef
5. A. G. Koryakova, Y. A. Ivanenkov, and A. V. Ivachtchenko, Bioorg. Med. Chem. Lett., 2008, 18, 3661. CrossRef
6. G. MekuH. Z. Zhang, S. Kasibhatla, J. Kuemmerle, and L. Qiu, J. Med. Chem., 2005, 48, 5215skine, P. Vainilavicius, A. Hetzheim, and R. Shematovich, Chem. Heterocycl. Compd., 1993, 29, 598. CrossRef
7. H. Z. Zhang, S. Kasibhatla, J. Kuemmerle, and L. Qiu, J. Med. Chem., 2005, 48, 5215. CrossRef
8. A. L. Braga, D. S. Ludtke, E. E. Alberto, and L. Dornelles, Synthesis, 2004, 10, 1589. CrossRef
9. (a) W. Du, W. K. Hagmann, and J. J. Hale, Tetrahedron Lett., 2006, 47, 4271; CrossRef (b) W. Du, Q. Truong, and H. B. Qi, Tetrahedron Lett., 2007, 48, 2231. CrossRef
10. (a) F. A. Kang, J. Kodah, Q. Y. Guan, X. B. Li, and W. V. Murray, J. Org. Chem., 2005, 70, 1957; CrossRef (b) N. A. Kheder, Y. N. Mabkhot, and A. M. Farag, Heterocycles, 2009, 78, 937. CrossRef
11. (a) Y. L. Zhu, Y. J. Pan, and S. L. Huang, Heterocycles, 2005, 65, 133; CrossRef (b) S. V. Ryabukhin, A. S. Plaskon, E. N. Ostapchuk, D. M. Volochnyuk, and A. A. Tolmachev, Synthesis, 2007, 3, 417. CrossRef
12. (a) C. O. Kappe and A. Stadler, Org. React., 2004, 63, 1; (b) C. O. Kappe, Tetrahedron, 1993, 49, 6937. CrossRef
13. A. Puchala, F. Belaj, J. Bergman, and C. O. Kappe, J. Heterocycl. Chem., 2001, 38, 1345. CrossRef
14. E. Okada and N. Tsukushi, Heterocycles, 1999, 51, 2471. CrossRef
15. C. O. Kappe and P. Roschger, J. Heterocycl. Chem., 1989, 26, 1555. CrossRef
16. M. Nasresfahani, M. Montazerozohori, and K. Abdi, ARKIVOC, 2009, 10, 255.
17. K. Yamamoto, Y. G. Chen, and F. G. Buono, Org. Lett., 2005, 7, 4673. CrossRef
18. (a) L. Feldberg and Y. Sasson, Tetrahedron Lett., 1996, 37, 2063; CrossRef (b) L. Feldberg and Y. Sasson, J. Chem. Soc., Chem. Commun., 1994, 1807. CrossRef