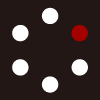
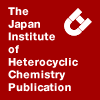
HETEROCYCLES
An International Journal for Reviews and Communications in Heterocyclic ChemistryWeb Edition ISSN: 1881-0942
Published online by The Japan Institute of Heterocyclic Chemistry
e-Journal
Full Text HTML
Received, 30th July, 2010, Accepted, 27th September, 2010, Published online, 28th September, 2010.
DOI: 10.3987/COM-10-12031
■ Synthesis of Readily Accessible BODIPY-Based Fluorescence Derivatization Reagents for Amines
Ryota Saito* and Eri Ito
Toho University, 2-2-1, Miyama, Funabashi, Chiba 274-8510, Japan
Abstract
Two BODIPY-based fluorescence derivatization reagents (FDRs) for amines were synthesized in a simple manner with practically acceptable yields via five steps. Both FDRs showed prompt reactivity, even against sterically hindered amines, to afford the corresponding FDR-labeled products in good yields. The solvent effect upon the fluorescence intensity of the derivatized amine was also investigated in a methanol-water binary solvent system to demonstrate that the strong fluorescence intensity was maintained in the solvent with a 0-60% water ratio. The detection limits of the new FDRs were estimated to be 20 fmol, which makes it much more sensitive than commercially available FDRs.The detection and quantification of trace amounts of amines and their derivatives in biological systems are important in the field of medical diagnoses. High-performance liquid chromatography (HPLC) in combination with fluorescence detection is known to be a powerful tool for the sensitive and expeditious detection of trace amounts of biological molecules. Because most biologically important amines do not contain necessary fluorophoric groups, a number of precolumn fluorescence derivatization reagents (FDRs) have been developed containing fluorophores such as NBD,1 fluorescein,2 and borondipyrromethenes (BODIPYs), all of which have different structures.3 Among these, BODIPYs have attracted considerable attention owing to their high extinction and fluorescence efficiencies, excellent photostability, largely red-shifted emission colors, and flexibility toward chemical modifications. Thus, a number of amine-labeling agents with BODIPY skeletons have been developed. However, most commercially available BODIPY-based FDRs are expensive and the methods used for their synthesis are complicated. Therefore, it is still necessary to develop BODIPY-based FDRs that are easy to prepare.
We recently developed a BODIPY-based luminescent probe for reactive oxygen species using a short number of reaction steps.4 The synthesis of this probe suggested that compounds 1 and 2, which consisted of a BODIPY and a succinimidyl group as the reactive unit for amines, can be synthesized quickly by simply modifying the synthetic route employed in the previous study. Here, we describe the synthesis of these compounds and their application as FDRs.
The synthetic routes used to obtain 1 and 2 are shown in Scheme 1. Amine 4 was prepared from 4-nitrobenzaldehyde and 3-ethyl-2,4-dimethylpyrrole via 3 as demonstrated previously.4, 5 Compound 4 was subsequently reacted with isocyanates 5 and 6 to produce 7 and 8, respectively, with quantitative yields. Saponification of 7 and 8 gave the corresponding carboxylic acids 9 and 10, respectively, with high yields. A conventional procedure for preparing a succinimidyl ester from a carboxylic acid afforded the target compound (1) from 9 with a good yield. Analog 2 was similarly obtained from 10 with a moderate yield. The yield of 2 was lower than that of 1 because of repeated column chromatography carried out to remove a byproduct, which had polarity similar to that of 2. Although the yield of 2 was slightly diminished, the syntheses of 1 and 2 were achieved via five steps with practically acceptable yields.
For sensitive and rapid detection of analytes, it is important for FDRs to readily react with target compounds. To confirm the reactivity of compounds 1 and 2 with amines, they were reacted with several aliphatic amines at room temperature. Both 1 and 2 readily reacted with n-butylamine. Figure 1 shows a representative time course of the reaction of 2 monitored by HPLC analysis. Although this reaction was almost completed within 10 min to give the corresponding amide, it required more time for the yield to be sufficient. The maximum and constant peak areas were attained after 20 min. In addition, 1 was reacted with isopropylamine and tert-butylamine. The reaction times for all of the examinations are summarized in Table 1. Each compound exhibited excellent reactivity, and all of the reactions were completed within 35 min with an almost quantitative yield. It is important to note that 1 exhibited good reactivity even with sterically hindered isopropylamine and tert-butylamine.
The UV-Vis absorption and fluorescence (FL) spectra of 1 and 2 and their n-butylamine conjugates were measured. The results are summarized in Table 2. Each compound exhibited the maximum absorption band at 522 nm and strong fluorescence emission around 535 nm with a fluorescence quantum yield of about 0.7. The selected UV-Vis and FL spectra of 11a are shown in Figure 2. The other compounds exhibited similar behavior. It should be noted that the high fluorescence efficiency of 1 and 2 was maintained after the derivatization reaction with the amine.
Generally, fluorescent detection of biomolecules is achieved in a medium containing water. Therefore, it is important to investigate the effect of water concentration on the fluorescence intensity. Accordingly, the fluorescence spectra of 11a were measured in aqueous MeOH containing various amounts of water. Figure 3 shows the plots of fluorescence maxima and the relative fluorescence intensity against the given water ratio. The fluorescence intensity of 11a remained almost constant with the change in the water ratio from 0 to 60% (v/v), but a rapid decrease occurred when the water ratio increased to > 70%. However, the fluorescence maximum was not affected by the water ratio. A similar phenomenon was observed in the UV-Vis absorption in which the absorbance decreased rapidly when the water ratio increased to above 70% (data not shown). These results indicate that the observed fluorescence quenching may be caused by decomposition of the fluorophore with water or by the poor solubility of 11a toward aqueous media. To determine the cause of this quenching, methanol was added to the solution of 11a in the ratio 25% methanol:75% water so as to increase the proportion of methanol to 85%. A subsequent measurement of the fluorescent spectra revealed that the fluorescence intensity had recovered to almost the same levels as that separately measured for 11a in 85% methanol at the same concentration (Figure 4).
These findings confirmed that the observed fluorescence quenching was due to the poor solubility of 11a toward such polar protic media. To evaluate the sensitivity of 1 and 2 during HPLC analysis, the detection limits of their n-butylamine adducts 11a and 12a, respectively, were estimated. For comparison, Dansyl-Cl, FITC, and NBD-Cl, which are known to be effective FDRs detectable at the pico-mol order, were also reacted with n-butylamine and the detection limits of the resulting amine adducts were estimated under the same conditions. In all analyses, excitation and emission wavelengths for detection were set at the maximum wavelengths. The results are summarized in Table 3. All FDRs reacted effectively with n-butylamine except for NBD-Cl. The detection limits of 11a and 12a were estimated to be 20 fmol/1.0-μL injection (S/N = 2), which are much lower than those of other amine adducts. These results clearly indicate that 1 and 2 are superior FDRs when compared to other FDRs.
In summary, highly sensitive FDRs for amines were prepared via a five-step synthetic route. The fluorescent properties of the corresponding amine-FDR conjugates provided significant information regarding the solubility of the BODIPYs in aqueous media. This information suggests interesting possibilities for the fine detection of biomaterials using BODIPY-based fluorescent probes.
EXPERIMENTAL
General. All melting points were measured on MEL-TEMP apparatus (LABORATORY DEVICES Inc, U.S.A.) in open capillary tubes; the values are uncorrected. 1H-NMR spectra were recorded on a JNM-ECP400 spectrometer (JEOL Ltd., Japan). Chemical shifts (δ) are reported in ppm and were measured using tetramethylsilane or an undeuteriated solvent as an internal standard in the deuterated solvent used. Coupling constants (J) are given in Hz. Chemical shift multiplicities are reported as s = singlet, d = doublet, t = triplet, q = quartet, quint = quintet, and m = multiplet. Infrared (IR) spectra were obtained using FT/IR-660plus spectrophotometers (JASCO Co., Ltd., Japan). Fast-atom-bombardment (FAB) mass spectra were measured on a JMS-600-H mass spectrometer (JEOL Ltd., Japan). Xenon was used as a bombardment gas, and all the analyses were carried out in a positive mode with the ionization energy and accelerating voltage set at 70 eV and 3 kV, respectively. A mixture of dithiothreitol (DTT) and α-thioglycerol (TG) (1:1 or 1:2) was used as a liquid matrix. High-resolution electron impact (EI) mass spectra were obtained with a JMS-AM II 50 mass spectrometer (JEOL Co., Ltd., Japan). The ionization energy was 70 eV, and the accelerating voltage was 0.5 kV. Absorption spectra were measured on a UV-550 spectrophotometer (JASCO Co., Ltd., Japan), and combustion analyses were performed on a MT-6 analyzer (Yanaco New Science Inc., Japan). Column chromatography was carried out on silica gel (particle size: 63–210 μm or 40–100 μm; Kanto Chemical Co.). Conventional chemicals used in the present study are commercially available and were used as received.
Ethyl 6-[4-(2,6-diethyl-4,4-difluoro-1,3,5,7-tetramethyl-4-bora-3a,4a-diaza-s-indacen-8-yl)phenyl]ureidohexanoate (7). Ethyl 6-isocyanatohexanoate (5) (0.07 ml, 0.39 mmol) was added to a solution of 45 (95.6 mg, 0.24 mmol) in anhydrous CH2Cl2/MeCN (1/1, 10 mL) at room temperature under an argon atmosphere. The reaction mixture was then refluxed for 10 h, after which water (10 mL) was added and the organic materials were extracted with CH2Cl2 (10 mL × 3). Next, the organic layer was dried over Na2SO4, and the solvents were evaporated. Finally, the crude material was purified by column chromatography on a silica gel using a hexane/EtOAc mixture as the eluent to give 7 as a red solid (quant.). mp 220–220.5 ºC. 1H NMR (CDCl3, 400 MHz) δ/ppm 7.49 (2H, d, J = 8.1 Hz), 7.18 (2H, d, J = 8.1 Hz), 6.39 (1H, s), 4.87 (1H, t, J = 7.0 Hz), 4.14 (2H, q, J = 7.3 Hz), 3.31 (2H, q, J = 7.0 Hz), 2.53 (6H, s), 2.34 (2H, t, J = 7.0 Hz), 2.30 (4H, q, J = 7.3 Hz) (6H, m), 1.67 (2H, quint, J = 7.0 Hz), 1.59 (2H, quint, J = 7.0 Hz), 1.41 (2H, quint, J = 7.0 Hz), 1.34 (6H, s), 1.27 (3H, t, J = 7.0 Hz), 0.98 (6H, t, J = 7.3 Hz). IR (KBr) νmax/cm–1 3343 (νN–H), 2957, 2929 (νC-H), 1733, (νC=O), 1651 (νC=O), 1315 (νB–O), 1186, 978 (νB–N). MS (FAB, DTT/TG = 1:2) m/z 581 [M+H]+, 561 [M–F]+. Anal. Calcd for C32H43BF2N4O3·0.6H2O: C, 64.99; H, 7.43; N, 9.48. Found: C, 64.73; H, 7.22; N, 9.19.
Ethyl 4-[4-(2,6-diethyl-4,4-difluoro-1,3,5,7-tetramethyl-4-bora-3a,4a-diaza-s-indacen-8-yl)phenyl]ureidobenzoate (8). Compound 8 was prepared in quantitative yield from 45 and ethyl 4-isocyanatobenzoate (6) by a procedure analogous to that used to obtain 5a. mp 257 ºC (decomp). 1H NMR (CDCl3, 400 MHz) δ/ppm 8.00 (2H, d, J = 8.8 Hz), 7.54 (2H, d, J = 8.8 Hz), 7.47 (2H, d, J = 8.8 Hz), 7.20 (2H, d, J = 8.8 Hz), 7.20 (1H, s), 7.15 (1H, s), 4.37 (2H, q, J = 7.0 Hz), 2.53 (6H, s), 2.29 (4H, q, J = 7.3 Hz), 1.40 (3H, t, J = 7.0 Hz), 1.33 (6H, s), 0.97 (6H, t, J = 7.3 Hz). IR (KBr) νmax/cm–1 3413 (νN–H), 1723 (νC=O), 1592 (νC=O), 1313 (νB–O), 1196, 982 (νB–N). MS (FAB, DTT/TG = 1:2) m/z 587 [M+H]+, 567 [M–F]+. Anal. Calcd for C33H37BF2N4O3·0.3AcOEt: C, 67.02; H, 6.48; N, 9.14. Found: C, 67.13; H, 6.34; N, 8.90.
6-[4-(2,6-Diethyl-4,4-difluoro-1,3,5,7-tetramethyl-4-bora-3a,4a-diaza-s-indacen-8-yl)phenyl]ureidohexanoic acid (9). To prepare 9, 1-M aqueous lithium hydroxide (1.50 ml) was added to a solution of 7 in EtOH/CH2Cl2 (4 ml/9 ml), and the mixed solution was then stirred at room temperature for 24 h. After completion of the reaction, water (35 ml) was added to the reaction mixture and the resulting solution was acidified (pH 6) with 0.5 M HCl. The organic materials were then extracted with EtOAc (100 mL × 3), after which the organic layer was dried over Na2SO4. Removal of the solvent gave 9 directly as a red solid (223 mg, 90%). mp 260 ºC (decomp). 1H NMR (CDCl3, 400 MHz) δ/ppm 7.49 (2H, d, J = 8.4 Hz), 7.18 (2H, d, J = 8.8 Hz), 6.63 (1H, s), 4.88 (1H, t, J = 7.0 Hz), 3.29 (2H, q, J = 7.0 Hz), 2.52 (6H, s), 2.40 (2H, t, J = 7.0 Hz), 2.29 (4H, q, J = 7.3 Hz), 1.69 (2H, quint, J = 7.0 Hz), 1.59 (2H, quint, J = 7.0 Hz), 1.43 (2H, quint, J = 7.0 Hz), 1.34 (6H, s), 0.98 (6H, t, J = 7.3 Hz). IR (KBr) νmax/cm–1 3389 (νO–H), 1708 (νC=O), 1662 (νC=O), 1317 (νB–O), 1194, 981 (νB–N). MS (FAB, DTT/TG = 1:1) m/z 553 [M+H]+, 533 [M–F]+; Anal. Calcd for C30H39BF2N4O3·1.0H2O: C, 63.16; H, 7.07; N, 9.82. Found: C, 63.04; H, 6.92; N, 9.77.
6-[4-(2,6-Diethyl-4,4-difluoro-1,3,5,7-tetramethyl-4-bora-3a,4a-diaza-s-indacen-8-yl)phenyl]ureidohexanoic acid O-succinimidyl ester (1). To produce 1, WSC·HCl (59.4 mg, 0.31 mmol) in CH2Cl2 (3 mL) was added to a mixture of 9 (112.4 mg, 0.20 mmol) and N-hydroxysuccinimide (35.3 mg, 0.31 mmol) in THF (15 mL) at 0 °C under an argon atmosphere. The resulting mixture was then stirred at room temperature for 7 h. Next, the reaction mixture was washed with water (20 mL) and extracted with EtAOc (30 mL × 3). The organic phase was then washed with brine and dried over Na2SO4. Following the evaporation of the solvent, the residue was purified by chromatography on silica gel using CH2Cl2 as an eluent to give 1 as a red solid (105.4 mg, 80%). mp 193–194.5 ºC. 1H NMR (CDCl3, 400 MHz) δ/ppm 7.51 (2H, d, J = 8.4 Hz), 7.15 (2H, d, J = 8.4 Hz), 7.01 (1H, s), 5.22 (1H, t, J = 7.0 Hz), 3.35 (2H, q, J = 7.0 Hz), 2.94 (2H, s), 2.93 (2H, s), 2.69 (2H, t, J = 7.0 Hz), 2.52 (6H, s), 2.29 (4H, q, J = 7.3 Hz), 1.84 (2H, quint, J = 7.0 Hz), 1.62 (2H, quint, J = 7.0 Hz), 1.58–1.52 (2H, m, overlapped with H2O protons), 1.35 (6H, s), 0.98 (6H, t, J = 7.3 Hz). IR (KBr) νmax/cm–1 3377 (νN–H), 1740 (νC=O), 1652 (δN–H), 1542 (νC=O), 1317 (νB–O), 1194, 981 (νB–N). MS (FAB, DTT/TG = 1:1) m/z 650 [M+H]+, 630 [M–F]+. Anal. Calcd for C34H42BF2N5O5·0.3H2O: C, 62.35; H, 6.51; N, 10.70. Found: C, 62.16; H, 6.44; N, 10.45.
4-[4-(2,6-Diethyl-4,4-difluoro-1,3,5,7-tetramethyl-4-bora-3a,4a-diaza-s-indacen-8-yl)phenyl]ureidobenzoic acid O-succinimidyl ester (2). The precursor 10 was prepared from 8 by a procedure analogous to that used to obtain 7 (83%); 1H NMR (DMSO-d6, 400 MHz) δ/ppm 12.6 (1H, s), 9.15 (1H, s), 9.07 (1H, s), 7.88 (2H, d, J = 8.5 Hz), 7.66 (2H, d, J = 8.5 Hz), 7.58 (2H, d, J = 8.5 Hz), 7.25 (2H, d, J = 8.5 Hz), 2.43 (6H, s), 2.30 (4H, q, J = 7.3 Hz), 1.34 (6H, s), 0.94 (6H, t, J = 7.3 Hz); IR (KBr) νmax/cm–1 3398 (νO–H), 3376 (νN–H), 1692 (νC=O), 1594 (δN–H), 1542 (νC=O), 1313 (νB–O), 1196, 982 (νB–N). MS (FAB, DTT/TG = 1:2) m/z 559 [M+H]+, 539 [M–F]+; This material was used in the next step without further purification. Next, 10 was converted into 2 by a procedure analogous to that used to obtain 1 in 56% yield. mp 272 ºC (decomp). 1H NMR (CDCl3, 400 MHz) δ/ppm 7.93 (2H, d, J = 8.4 Hz), 7.73 (1H, s,), 7.62 (1H, s), 7.56 (2H, d, J = 8.4 Hz), 7.44 (2H, d, J = 8.4 Hz), 7.13 (2H, d, J = 8.4 Hz), 2.97 (4H, s), 2.53 (6H, s), 2.29 (4H, q, J = 7.3 Hz), 1.34 (6H, s), 0.97 (6H, t, J = 7.3 Hz). IR (KBr) νmax/cm–1 3496 (νN–H), 1737 (νC=O), 1590 (δN–H), 1539 (νNC=O), 1316 (νB–O), 1197, 983 (νB–N). MS (FAB, DTT/TG = 1:1) m/z 656 [M+H]+, 636 [M–F]+. Anal. Calcd for C35H36BF2N5O5·2.5H2O: C, 60.00; H, 5.54; N, 10.00. Found: C, 59.90; H, 5.43; N, 9.82.
Derivatization reactions. To a solution of an FDR (21 μmol) in DMF (0.7 ml) was added n-butylamine (4.2 μL, 25 μmol) and stirred at room temperature for 3.5 h under argon atmosphere. After evaporation of the solvent, the crude material was purified by column chromatography on silica gel using EtOAc as eluents to give the corresponding FDR-derivatized amine.
N-Butyl-6-[4-(2,6-diethyl-4,4-difluoro-1,3,5,7-tetramethyl-4-bora-3a,4a-diaza-s-indacen-8-yl)phenyl]ureidohexanamide (11a) was obtained in 80% yield; 1H NMR (CDCl3 400 MHz) δ/ppm 7.53 (2H, d, J = 8.4 Hz), 7.15 (2H, d, J = 8.4 Hz), 6.97 (1H, s), 5.56 –5.49 (2H, m), 3.33 (2H, q, J = 7.0 Hz), 3.29 (2H, q, J = 7.0 Hz), 2.52 (6H, s), 2.29 (4H, q, J = 7.3 Hz), 2.24 (2H, t, J = 7.0 Hz), 1.69 (2H, quint, J = 7.0 Hz), 1.61–1.47 (4H, m), 1.42 (0.98 (6H, t, J = 7.3 Hz), 0.92 (3H, t, J = 7.3 Hz); IR (KBr) νmax/cm–1 1708 (νC=O), 1644 (νC=O), 1543, 1477 (C=C, C=N, ring stretching ), 1316 (νB–O), 1191, 979 (νB–N); MS (FAB, DTT/TG = 1:2) m/z 608 [M+H]+ and 588 [M–F]+.
N-n-Butyl-4-[4-(2,6-diethyl-4,4-difluoro-1,3,5,7-tetramethyl-4-bora-3a,4a-diaza-s-indacen-8-yl)phenyl]ureidobenzamide (12a) was obtained quantitatively 1H NMR (CDCl3, 400 MHz) δ/ppm 8.15 (1H, s, NH), 8.13 (1H, s, NH), 7.65 (2H, d, J = 8.4 Hz, ArH), 7.61 (2H, d, J = 8.4 Hz, ArH), 7.39 (2H, d, J = 8.4 Hz, ArH), 7.19 (2H, d, J = 8.4 Hz, ArH), 6.32 (1H, t, J = 7.0 Hz, NH), 3.51 (2H, q, J = 7.0 Hz), 2.53 (6H, s), 2.29 (4H, q, J = 7.3 Hz), 1.65 (2H, quint., J = 7.0 Hz), 1.43 (2H, sex, J = 7.0 Hz), 1.34 (6H, s), 0.97 (6H, t, J = 7.3 Hz), 0.96 (3H, t, J = 7.0 Hz); MS (FAB, DTT/TG = 1:1) m/z 614 [M+H]+ and 594 [M–F]+.
N-Isopropyl-4-[4-(2,6-diethyl-4,4-difluoro-1,3,5,7-tetramethyl-4-bora-3a,4a-diaza-s-indacen-8-yl)phenyl]ureidohexanamide (11b) was obtained in 92% yield 1H NMR (CDCl3, 400 MHz) δ/ppm 7.85 (1H, s, NH), 7.56 (2H, d, J = 8.4 Hz), 7.12 (2H, d, J = 8.4 Hz), 5.86 (1H, t, J = 7.0 H), 5.73 (1H, d, J = 6.6 Hz), 4.09 (1H, octet, J = 6.6 Hz), 3.29 (2H, q, J = 7.0 Hz), 2.52 (6H, s), 2.28 (4H, q, J = 7.3 Hz), 2.20 (2H, t, J = 7.0 Hz), 1.67 (2H, quint, J = 7.0 Hz), 1.56 (2H, quint, J = 7.0 Hz), 1.40 (2H, quint, J = 7.0 Hz), 1.33 (6H, s), 1.17 (6H, d, J = 6.6 Hz), 0.96 (6H, t, J = 7.3 Hz); MS (FAB, DTT/TG = 1:1) m/z 594 [M+H]+ and 574 [M–F]+.
N-tert-Butyl-4-[4-(2,6-diethyl-4,4-difluoro-1,3,5,7-tetramethyl-4-bora-3a,4a-diaza-s-indacen-8-yl)phenyl]ureidobenzamide (11c) was obtained quantitatively; 1H NMR (CDCl3, 400 MHz) δ/ppm 7.79 (1H, s), 7.56 (2H, d, J = 8.4 Hz), 7.12 (2H, d, J = 8.4 Hz), 5.90 (1H, t, J = 7.0 Hz), 5.60 (1H, s), 3.28 (2H, q , J = 7.0 Hz), 2.52 (6H, s), 2.28 (4H, q, J = 7.3 Hz), 2.16 (2H, t, J = 7.0 Hz), 1.64 (2H, quint, J = 7.0 Hz), 1.56 (2H, quint, J = 7.0 Hz), 1.40 (2H, quint, J = 7.0 Hz), 1.37 (9H, s), 1.33 (6H, s), 0.97 (6H, t, J = 7.3 Hz); MS (FAB, DTT/TG = 1:1) m/z 608 [M+H]+ and 588 [M–F]+. Dansyl-Cl, FITC and NBD-Cl were reacted with n-butylamine by the same procedure that for obtaining 11a–c. The corresponding derivatized products, N-n-butyl-5-(dimethylamino)naphthalene-1-sulfonamide (N-n-butyl-Dansyl)6, N-n-butyl-5-thioureido-fluorescein (FTU-n-butyl),7 and 4-n-butylamino-7-nitrobenzo[c][1,2,5]oxadiazol (4-n-butylamino-NBD),8 were obtained in quantitative, 87% and 34% yields, respectively. The identification of these products was achieved by comparing their spectral features with those of previously reported data.
Fluorescence measurements
Fluorescence spectra were collected using a JASCO F-777 Spectrofluorometer. The excitation wavelength was set at 492 nm for each measurement. The fluorescence quantum yields were measured at 25 °C relative to fluorescein in 0.1 M NaOH (ΦFL = 0.85, excited at 467 nm) and then calculated according to the manufacturer’s instructions.
HPLC analysis
HPLC analysis was conducted using a Capcell Pak C18 SG120 column (particle size: 5 μm, 250 mm × 4.6 mm id., SHISEIDO Co., Ltd., Japan) with an SSC-3461 pump (SENSHU SCIENTIFIC Co. Ltd., Japan), a DG-1110 degasser (SENSHU SCIENTIFIC Co., Ltd., Japan), an 875-UV Intelligent UV/Vis detector (JASCO Co., Ltd, Japan), and a FL SPECTRO PHOTO METER S-3370 (SOMA Co Ltd., Japan). HPLC-grade methanol and water were used as the eluents. The analytes were eluted with 90% (v/v) MeOH at a flow rate of 1.0 mL/min. The excitation wavelength of the detector was set at 520 nm and the fluorescence was detected at 540 nm. Measurements were conducted at a temperature of 25 ºC, which was maintained using a SSC-2300 column oven (SENSHU SCIENTIFIC Co. Ltd., Japan).
ACKNOWLEDGEMENTS
This work was financially supported by “High-Tech Research Center” Project for Private Universities: matching fund subsidy from the MEXT (2005-2009).
References
1. (a) S. Uchiyama, T. Santa, T. Fukushima, H. Homma, and K. Imai, J. Chem. Soc., Perkin Trans. 2, 1998, 2165; CrossRef (b) S. Uchiyama, K. Takehira, S. Kohtani, T. Santa, R. Nakagaki, S. Tobita, and K. Imai, Phys. Chem. Chem. Phys., 2002, 4, 4514. CrossRef
2. (a) H. Maeda, N. Ishida, H. Kawauchi, and K. Tuzimura, J. Biochem., 1969, 65, 777; (b) H. Kawauchi, K. Tuzimura, H. Maeda, and N. Ishida, J. Biochem., 1969, 66, 783; (c) B. Shi, W. Huan, and J. Cheng, J. Sep. Sci., 2008, 31, 1144. CrossRef
3. (a) J. Li, H. Wang, L. Cao, and H. Zhang, Talanta, 2006, 69, 1190; CrossRef (b) J. Li, H. Wang, K. Huang, and H. Zhang, Anal. Chem. Acta, 2006, 575, 255. CrossRef
4. R. Saito, A. Ohno, and E. Ito, Tetrahedron, 2010, 66, 583. CrossRef
5. (a) R. Ziessel, L. Bonardi, P. Retailleau, and G. Ulrich. J. Org. Chem., 2006, 71, 3093; CrossRef (b) L. Bonardi, G. Ulrich, and R. Ziessel. Synlett, 2006, 450. CrossRef
6. V. S. Jisha, A. J. Thomas, and D. Ramaiah, J. Org. Chem., 2009, 74, 6667. CrossRef
7. A. Junnila, D. S. Bohle, R. Prichard, I. Perepichka, and C. Spina, Bioconjugate Chem., 2007, 18, 1818. CrossRef
8. M. R. Crampton, C. Isanbor, and T. Willett, Can. J. Chem., 2005, 83, 1222 CrossRef