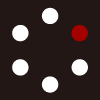
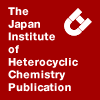
HETEROCYCLES
An International Journal for Reviews and Communications in Heterocyclic ChemistryWeb Edition ISSN: 1881-0942
Published online by The Japan Institute of Heterocyclic Chemistry
e-Journal
Full Text HTML
Received, 10th August, 2010, Accepted, 26th August, 2010, Published online, 26th August, 2010.
DOI: 10.3987/COM-10-12042
■ An Enantio- and Diastereocontrolled Synthesis of (–)-Salinosporamide A
Yosuke Sato, Hayato Fukuda, Masaki Tomizawa, Tomohito Masaki, Masatoshi Shibuya, Naoki Kanoh, and Yoshiharu Iwabuchi*
Pharmaceutical Institute, Tohoku University, Aobayama, Sendai 980-8578, Japan
Abstract
The enantio- and diastereocontrolled total synthesis of (–)-salinosporamide A, a potent 20S proteasome inhibitor, was accomplished through organocatalytic aldolization, diastereoselective Claisen condensation, a Rh-catalyzed Reformatsky reaction, and an AZADO-catalyzed oxidative β-lactonization reaction as the key reactions.Salinosporamide A (1, aka NPI-0052, marizobnib) was discovered by Fenical and coworkers as a cytotoxic principle produced by a marine actinomycete Salinosopra tropicana.1 Structurally, salinosporamide A has the same unique fused β-lactone-γ-lactam core as omuralide (2), a well-known 20S proteasome inhibitor derived from lactacystin (3),2 but is distinguished by its five contiguous asymmetric centers consisting of a β-chloroethyl group, two quaternary carbons, and a 2-cyclohexene ring. Salinosporamide A has been reported to be approximately 35 times more effective at proteasome inhibition than omuralide,1 making it as a candidate for the development of anticancer drugs.3 The beneficial pharmacological profile of salinosporamide A coupled with its structural complexity has spurred intense efforts to synthesize it.
To date, a number of total4,5 and formal6 syntheses have been accomplished, most of which employ Corey’s approach4a to realize the diastereoselective installation of the cyclohexene ring of 1 in the later stage of the synthesis, with the exception of the Nagamitsu-Omura synthesis,4e in which the cyclohexene moiety is mounted in the early stage. We now report the enantio- and diastereocontrolled synthesis of (–)-salinosporamide A (1) featuring the extensive adoption of aldol and related reactions, where the cyclohexene ring is installed in the early stage of the synthesis.
Our retrosynthetic analysis of 1 is illustrated in Scheme 1. We set 4 as a logical subgoal, of which the deprotected oxazoline and the methyl ester moieties could serve as suitable intermediates to obtain 1. Among several possible disconnections, we chose the intermolecular aldol reaction of the ketone 5 and an enolate derived from γ-butyrolactone, which would ultimately allow us to secure the vicinal asymmetric quaternary centers in a diastereocontrolled manner. The quaternary center of the ketone 5 should be secured from the oxazoline 6 via a diastereocontrolled mixed Claisen condensation with an acetyl counterpart. The precursor of the oxazoline 6 was expediently assigned to the known aldol 7:7 the highly diastereocontrolled synthesis of the enantiomeric ent-7 was previously attained via an L-proline-catalyzed aldol reaction of (S)-Garner’s aldehyde8a and cyclohexanone.
The oxazoline 12, serving as the stereochemical basis throughout the synthesis, was prepared as shown in Scheme 2. In accordance with the procedure developed by Rode,7 (R)-Garner’s aldehyde8b was treated with cyclohexanone in the presence of 20 mol% D-proline in CHCl3-DMSO (3:1) in 5 °C to give 7 with 81% yield and a high diaseteroselectivity of over 98% de, of which the diastereochemical preference is rationalized by the transition state model A. 7 Upon TMS etherification, triflation of the kinetically generated enolate of 7 afforded 8. Pd-catalyzed reduction of the triflate 8 employing formic acid gave 9 with concomitant desilylation. The exposure of 9 to a mixture of cHCl and AcOEt effected the deprotection of the acetonide and the Boc group to give the corresponding amino-diol, which was then condensed with p-chlorobenzoyl chloride to give 10. Note that the installation of the p-chlorobenzoyl group was not the result of a capricious choice (vide infra).9 Treatment of 10 with a catalytic amount of TEMPO in the presence of PhI(OAc)210 in CH3CN-H2O (1:1) led to the selective oxidation of the primary alcohol moiety, which upon treatment with diazomethane gave the methyl ester 11. The cyclization of 11 to the oxazoline 12 was accomplished in boiling toluene using a catalytic amount of p-TsOH and Dean-Stark apparatus.
Having formed the projected chiral platform 12, we considered the formation of the vicinal quaternary centers in 1 (Scheme 3). The deprotonation of 12 with lithium diisopropylamide in THF and acylation of the resulting enolate with acetyl chloride afforded the required 13 exclusively with 76% yield. The next step, the attachment of γ-butyrolactone, turned out to be troublesome: all the attempted aldol reactions failed to give even a trace amount of the anticipated product and instead gave 2-acetyl-γ-butyrolactone (14) as a major product, indicating the formation of the primary adduct B and the prompt collapse via a retro-Claisen reaction. After many unsuccessful trials, we decided to make a detour so as to accommodate the transient oxy-anion species generated by the nucleophilic attack of the enolate counterpart (Scheme 3).
To this end, ketone 13 was selectively reduced to the alcohol 15 by treatment with NaBH4 in MeOH at –40 °C. Upon THP protection, the reduction of the ester moiety with DIBALH, acetylation, and the removal of the THP group, we obtained 16 from 15. Oxidation of the highly congested alcohol 16 was easily achieved under 1-Me-AZADO11-catalyzed conditions using PhI(OAc)2 to give 17 as a revised substrate for attaching the γ-butyrolactone moiety. Owing to the challenging steric hindrance of 17, most of attempted aldolizations including the Mukaiyama aldolization12 using (4,5-dihydrofuran-2-yloxy)trimethylsilane were unsuccessful and only Shibasaki’s protocol13 using CuF·3PPh3·2EtOH/(EtO)3SiF gave the desired adduct with 8% yield. Eventually, we found that the Honda variant14 of the Reformatsky conditions gave the best results. Thus, 17 was treated with α-bromo-γ-butyrolactone and Et2Zn in the presence of 5 mol% Wilkinson’s catalyst to give 18a and 18b in 31% and 30% isolated yields, respectively. The relative stereochemistry of 18a was confirmed by X-ray analysis of the corresponding Δ7,8-hydrogenated derivative.15 Fortunately, 18a was cleanly isomerized to the desired epimer 18b upon treatment with DBU. Detachment of the chlorophenylmethylene portion of 18b was best effected in a two-step sequence through the convenient formation of γ-lactam: (1) reduction of oxazoline with NaBH3CN4a to form 19 via an intramolecular translactamization, (2) removal of the p-chlorobenzylidene group with cHCl and 1,3-propanedithiol16 to give 20. It should be stressed that the p-methoxy counterpart4a of 18b was significantly resistant9 to reduction, resulting in a significant yield loss (Scheme 3).
To progress from 20 to (–)-1 (Scheme 4), the primary and secondary hydroxyl groups had selectively to be protected in a discriminable manner, with the acetate group detached, of which purpose was expediently attained via the selective TBDPS etherification, deacetylation, selective TMS etherification of the primary and secondary hydroxyl groups, and selective removal of the primary TMS ether on treatment with silica gel to furnish 21. Fortunately, upon the attempted oxidation of the primary alcohol under AZADO11-catalyzed conditions using PhI(OAc)2, 21 directly afforded β-lactone 22. Note that the use of TEMPO17 significantly retarded the formation of β-lactone. Global deprotection of the silyl groups in 22, followed by treatment of the resulting diol with Ph3PCl24a finally gave (–)-salinosporamide A. The specific rotation, melting point, and spectroscopic properties of the synthetic 1 were full accordance with the reported data.
In conclusion, we have developed an enantio- and diastereocontrolled route to synthesize (–)-salinosporamide A from (R)-Garner aldehyde. The key features of the synthetic route are the Rh-catalyzed Reformatsky reaction of 17 to 18, and the simple, one-step, oxidative β-lactonization of 22 catalyzed by AZADO. Additional information on the chemo- and diastereoselective manipulations around the β-lactone-γ-lactam motif should provide useful insight for the design and synthesis of advanced 20S proteasome inhibitors related to salinosporamide A/lactacystin.
ACKNOWLEDGEMENTS
This work was financially supported in part by a Grant-in-Aid for Scientific Research on Priority Areas 180320110 and Grant-in-Aid for Global COE Program for “International Center of Research & Education for Molecular Complex Chemistry” from Ministry of Education, Culture, Sports, Science, and Technology, Japan.
References
1. R. H. Felig, G. O. Buchanan, T. J. Mincer, C. A. Kauffman, P. R. Jensen, and W. Fenical, Angew. Chem. Int. Ed., 2003, 42, 355. CrossRef
2. (a) S. Omura, T. Fujimoto, K. Otoguro, K. Matsuzaki, R. Moriguchi, H. Tanaka, and Y. Sasaki, J. Antibiot., 1991, 44, 113; (b) S. Omura, K. Natsuzaki, T. Fujimoto, K. Kosuge, T. Furuya, S. Fujita, and A. Nakagawa, J. Antibiot., 1991, 44, 117.
3. (a) D. Chauhan, L. Catley, G. Li, K. Podar, T. Hideshima, M. Velankar, C. Mitsiades, N. Mitsiades, H, Yasui, A. Letai, H, Ovaa, C, Berkers, B. Nicholson, T.-H, Chao, S. T. C. Neuteboom, P. Richardson, M. A. Palladino, and K. C. Anderson, Cancer Cell, 2005, 8, 407; CrossRef (b) V. R. Macherla, S. S. Mitchell, R. R. Manam, K. A. Reed, T.-H. Chao, B. Nicholson, G. Deyanat-Yazdi, B. Mai, P. R. Jensen, W. Fenical, S. T. C. Neuteboom, K. S. Lam, M. A. Palladino, and B. C. M. Potts, J. Med. Chem., 2005, 48, 3684; CrossRef (c) W. H. Fenical, P. R. Jensen, M. A. Palladino, K. S. Lam, G. K. Lloyd, and B. C. Pott, Bioorg. Med. Chem., 2009, 17, 2157; CrossRef (d) A. V. Singh, M. A. Palladino, G. K. Lloyd, B. C. Potts, D. Chauhan, and K. C. Anderson, Br. J. Heamatol., 2010, 149, 550. CrossRef
4. Enantioselective synthesis; (a) L. R. Reddy, P. Saravanan, and E. J. Corey, J. Am. Chem. Soc., 2004, 126, 6230; CrossRef (b) A. Endo and S. J. Danishefsky, J. Am. Chem. Soc., 2005, 127, 8298; CrossRef (c) T. Ling, V. R. Macherla, R. R. Manam. K. A. McArthur, and B. C. M. Potts, Org. Lett., 2007, 9, 2289; CrossRef (d) K. Takahashi, M. Midori, K. Kawano, J. Ishihara, and S. Hatakeyama, Angew. Chem. Int. Ed., 2008, 47, 6244; CrossRef (e) T. Fukuda, K. Sugiyama, S. Arima, Y. Harigaya, T. Nagamitsu, and S. Omura, Org. Lett., 2008, 10, 4239; CrossRef (f) H. Nguyen, G. Ma, and D. Romo, Chem. Commun., 2010, 46, 4803. CrossRef
5. Racemic synthesis; (a) N. P. Mulholland, G. Pattenden, and I. A. S. Walters, Org. Biomol. Chem., 2006, 4, 2845; CrossRef (b) G. Ma, H. Ngayen, and D. Romo, Org. Lett., 2007, 9, 2143. CrossRef
6. Formal synthesis; (a) V. Caubert, J. Massé, P. Retailleau, and N. Langlois, Tetrahedron Lett., 2007, 48, 381; CrossRef (b) I. V. Margalef, L. Rupnicki, and H. W. Lam, Tetrahedron, 2008, 64, 7896; CrossRef (c) T. Momose, Y. Kaiya, N. Hama, J. Hasegawa, T. Sato, and N. Chida, Synthesis, 2009, 2983; CrossRef (d) R. A. Mosey and J. J. Tepe, Tetrahedron Lett., 2009, 50, 295; CrossRef (e) J. R. Struble and J. W. Bode, Tetrahedron, 2009, 65, 4957; CrossRef (f) T. Ling, B. C. Potts, and V. R. Macherla, J. Org. Chem., 2010, 75, 3882. CrossRef
7. I. Kumar and C. V. Rode, Tetrahedron: Asymmetry, 2007, 18, 1975. CrossRef
8. (a) P. Garner and J. M. Park, J. Org. Chem., 1987, 52, 2361; CrossRef (b) A. McKillop, R. J. K. Taylor, R. J. Watson, and N. Lewis, Synthesis, 1994, 31. CrossRef
9. Y. Kaburagi, H. Osajima, H. Tokuyama, and T. Fukuyama, Tetrahedron Lett., 2004, 45, 3817. CrossRef
10. A. De Mico, R. Margarita, L. Parlanti, A. Vescovi, and G. Piancatelli, J. Org. Chem., 1997, 62, 6974. CrossRef
11. (a) M. Shibuya, M. Tomizawa, I. Suzuki, and Y. Iwabuchi, J. Am. Chem. Soc., 2006, 128, 8412; CrossRef (b) Y. Iwabuchi, J. Syn. Org. Chem. Jpn., 2008, 66, 1076. CrossRef
12. K. Narasaka, K. Banno, and T. Mukaiyama, Chem. Lett., 1973, 1011. CrossRef
13. K. Oisaki, Y. Suto, M. Kanai, and M. Shibasaki, J. Am. Chem. Soc., 2003, 125, 5644. CrossRef
14. K. Kanai, H. Wakabayashi, and T. Honda, Org. Lett., 2000, 2, 2549. CrossRef
15. Δ7,8-H2-18a was prepared from Δ7,8-H2-17, together with Δ7,8-H2-18b, under the same Honda-Reformatsky reaction conditions. The relative stereochemistry of Δ7,8-H2-18b was confirmed by observing the n.O.e of Δ7,8-H2-19.
Full account of our synthetic venture toward salinosporamide A will be reported elsewhere.
16. E. J. Corey and G. A. Reichard, J. Am. Chem. Soc., 1992, 114, 10677. CrossRef
17. (a) T. M. Hansen, G. J. Florence, P. Lugo-Mas, J. Chen, J. N. Abrams, and C. J. Forsyth, Tetrahedron Lett., 2003, 44, 57; CrossRef (b) M. Ebine, Y. Suga, H. Fuwa, and M. Sasaki, Org. Biomol. Chem., 2010, 8, 39. CrossRef