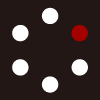
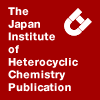
HETEROCYCLES
An International Journal for Reviews and Communications in Heterocyclic ChemistryWeb Edition ISSN: 1881-0942
Published online by The Japan Institute of Heterocyclic Chemistry
e-Journal
Full Text HTML
Received, 19th August, 2010, Accepted, 1st October, 2010, Published online, 4th October, 2010.
DOI: 10.3987/COM-10-12047
■ Isolation and Identification of a Gravity-Induced Growth Inhibitor in Etiolated Radish Hypocotyls
Tsuyoshi Hasegawa, Wai Wai Thet Tin,* Hideyuki Shigemori, Toshihiko Otomatsu, Katsutoshi Hirose, Kensuke Miyamoto, Junichi Ueda, and Koji Hasegawa
Graduate School of Life and Environmental Sciences, University of Tsukuba, Tsukuba, Ibaraki 305, Japan
Abstract
A growth inhibitor of which content in etiolated radish hypocotyls was increased under 500 g gravity conditions produced by centrifugation, but decreased under simulated microgravity conditions on a 3-dimensional clinostat, comparing to 1 g conditions, was isolated from etiolated radish hypocotyls grown under 1 g conditions. It was determined to be 3,6’-disinapoylsucrose by its 1H and 13C NMR and MS spectral data. When etiolated radish seedlings were placed horizontally, the gravitropic curvature took about 30 min to start. The distribution of endogenous 3,6’-disinapoylsucrose between the peripheral cell layers of the upper and lower flank of radish hypocotyls during gravitropic curvature was analyzed using a physicochemical assay. Its content rapidly increased in the peripheral cell layers of the upper halves, peaking at 30 min after the onset of gravitropic stimulation, then gradually decreased. On the other hand, the content in the peripheral cell layers of the lower halves was almost equal to that in the vertical control during gravitropic curvature. Its unilateral application caused the hypocotyls to bend toward the site of application. These results suggest that gravitropic stimulation suppresses the growth rate of the upper side of radish hypocotyls by increasing the content of the growth inhibitor 3,6’-disinapoylsucrose in the peripheral cell layers of the upper halves, causing a gravitropic response.INTRODUCTION
The plant seedling is equipped with a multitude of sensors that provide information about the environment in which it is growing. The directional movement of a plant in response to a unilateral stimulus is defined as "tropism". The bending of the organs of a plant toward or away from the earth is a well-known phenomenon. This response to gravity is called "gravitropism". A shoot is described as negatively gravitropic as it extends in the opposite direction to gravity (i.e. upwards) while a primary root is positively gravitropic in that it grows in the direction of the gravitational pull (i.e. downwards).
In gravitropism of shoots, it has been generally believed that the gravitropism is caused by gravity perception in the tip, leading to lateral auxin transport there, followed by basipetal auxin translocation to the elongation zone below the tip, resulting in an increase in the growth rate on the lower side of the shoot and an accompanying decrease in the rate on the upper side (Cholodny-Went theory).1,2 In contrast, however, Digby and Firn reported that the suppression of growth on the upper side of etiolated maize coleoptiles, de-etiolated sunflower, and etiolated cucumber hypocotyls is a major factor bringing about gravitropism.3 Growth suppression of the upper shoot flank following gravistimulation has also been reported in other plant systems.4-7 Recently, Tokiwa et al. measured using infrared-imaging system the changes in length on the upper and lower sides of etiolated radish hypocotyls prior to, and following gravistimulation.8 It was observed that the growth suppression was exhibited first on the upper side and then the acceleration in growth on the lower side started, suggesting that the suppression of growth on the upper side is a direct effect of gravity and the growth acceleration on the lower side is the result rather than a cause of gravitropic stimulation. From these results, it was suggested that gravitropism of radish hypocotyls may be caused by an increase in growth inhibitor(s) induced with gravitropic stimulation in the upper side, inducing growth inhibition there. In the present paper, we report on the isolation and identification of gravity-induced growth inhibitor(s) from large amounts of etiolated radish hypocotyls grown under 1 g conditions. The role of the growth inhibitor(s) in the gravitropism of radish hypocotyls will also be discussed.
RESULTS AND DISCUSSION
In order to search gravity-induced chemical substance(s), etiolated radish seedlings were grown under different magnitude of the gravitational force. Figure 1 shows the HPLC chromatograms of the extracts from hypocotyls grown on simulated microgravity conditions on a 3-dimensional clinostat and on 1 g conditions. Peaks A, B and C were decreased under simulated microgravity conditions, comparing to 1 g conditions. Figure 2 shows the HPLC chromatograms of the extracts from hypocotyls under 500 g gravity produced by centrifugation and on 1 g conditions. Only peak C was increased under 500 g conditions, comparing to 1 g conditions, whereas peaks A and B showed no change. These results suggest that peak
C is a gravity-induced substance. The peak C was isolated from large amounts of etiolated radish hypocotyls. From the 1H and 13C NMR and ESI-MS spectral data, isolated substance was identified as 3,6’-disinapoylsucrose (Figure 3). 3,6’-Disinapoylsucrose (DSS) has already been isolated from the bark of Securidaca longipedunculata9 and Polygala virgata.10 The DSS was also isolated from radish (Raphanus sativus) sprout as a strong antioxidant compound.11 The spectra of the 1H and 13C NMR of isolated peak C were absolutely in agreement with those of DSS reported in the literature.9-11 To test whether isolated DSS shows plant growth activity, its activity was studied using the cress radicle growth test which was generally used to examine plant growth-inhibiting activity. As shown in Figure 4, DSS inhibited the growth of cress radicles, recognizing as plant growth-inhibiting substance. The lateral distribution of DSS between the peripheral cell layers of the upper and lower flank of etiolated radish hypocotyls during gravitropic curvature was analyzed using a physicochemical assay (Table 1). The endogenous DSS level in the peripheral cell layers of the upper halves increased within 30 min after the onset of gravitropic stimulation, whereas that in the lower flank was similar to that in the controls. It was determined whether unilaterally applied DSS could induce bending toward the site of application. As shown in Table 2, the curvature of the hypocotyls toward the site of DSS application occurred with
amounts of 0.3 μg and more of DSS per seedling. When 0.3 μg of DSS was applied to one side of hypocotyl, about 10 ng of DSS per hypocotyl was detected in the peripheral cell layers of the applied side (data not shown), comparable to the endogenous DSS content (about 9 ng per hypocotyl) in the peripheral cell layers of the upper side of gravity-stimulated hypocotyl (Table 1). These results suggest that an increase in growth inhibitor 3,6’-disinapoylsucrose induced by gravistimulation in the upper side of etiolated radish hypocotyls causes the growth suppression on the upper side, resulting in gravitropic curvature. The observation of the gravitropic response of the etiolated radish hypocotyls in the present study is in agreement with that of the phototropic response in various plant species: a lateral gradient of the growth inhibitor(s) occur in the unilaterally illuminated side, causing a lateral difference in growth rate from which the phototropic response results.12-17 Whether gravitropism-regulating growth inhibitor(s) are same with phototropism-regulating growth inhibitor(s), is uncertain.
EXPERIMENTAL
Plant materials and simulated microgravity experiments
Dry seeds of radish (Raphanus sativus var. hortensis f. gigantissimus Makino) were put in rockwool (Minipot, Nittobo Co. Ltd., Tokyo, Japan) moistened with distilled water in an acrylic chamber. They were allowed to germinate and grow under simulated microgravity conditions on a 3-D clinostat and at 1 g conditions at 23 °C in the dark. A 3-D clinostat (CS-2, Nippon Medical and Chemical Instruments Co. Ltd., Osaka, Japan) was used to produce simulated microgravity conditions. After 4 days, 10 hypocotyls of ca. 2 cm were excised with razor blade from radish seedlings grown under simulated microgravity conditions and on 1 g conditions. Excised hypocotyls were stored at -40 °C until use.
Hypergravity experiments
Three-day-old, etiolated radish seedlings were placed in a centrifuge tube (3 cm in diameter, 7 cm in height) with moistened filter paper. The centrifuge tubes were then exposed to basipetal hypergravity by centrifugation at 500 g and at 1 g in the dark for 1 h. Ten etiolated hypocotyls of ca. 2 cm were excised from radish seedlings put under hypergravity conditions produced by centrifugation and 1 g conditions. Excised hypocotyls were stored at -40 °C until use.
Quantification of chemical substance(s) induced by different magnitude of the gravitational force
Each frozen hypocotyls were homogenized in 50 mL of 80% cold acetone using homogenizer. The filtered extracts were evaporated to dryness at 40 °C in vacuo. The samples were dissolved in methanol and applied to a HPLC (ODS-120A, Wako, Japan, 7.8 x 300 mm, 0-5 min; 0% MeCN in H2O, 5-25 min; linear gradient from 0 to 100% MeCN in H2O, 2 mL/min, detector at 280 nm and 320 nm) or a HPLC (ODS-120A, Wako, Japan, 4.6 x 250 mm, 1 mL/min, elution and detection conditions same as above).
This experiment was repeated three times.
Isolation of gravity-induced growth inhibitor(s)
For the purpose of identifying the peak C, 4-day-old, de-etiolated radish seedlings (1 kg fresh weight) were homogenized in 10 L of 80% acetone and the homogenate was filtered through a sheet of filter paper. The extracts were evaporated to dryness in vacuo at 40 °C. The concentrate was applied to a C18 Sep-Pak cartridge column (Waters) pretreated with 20% MeOH in H2O, and eluted with 20, 40, 60, 80 and 100% MeOH (40 mL per step). The 40% and 60% MeOH fractions, which contained the peak C, were combined and evaporated to dryness at 40 °C in vacuo and finally purified by HPLC (ODS-120A, Wako, Japan, 7.8 x 300 mm, 0-5 min; 0% MeCN in H2O, 5-25 min; linear gradient from 0% to 100% MeCN in H2O, 2 mL/min, detector at 280 and 320 nm). Elute of peak C was evaporated to dryness in vacuo at 40 °C to give 4 mg.
Spectrometric analysis
ESI-MS was recorded on a Waters Platform. The 1H and 13C NMR spectra were measured and recorded on a Bruker AVANCE500 spectrometer in CD3OD. The resonances of CD3OD at δH 3.35 and δC 49.8 were used as internal references for NMR spectra. On the basis of negative ESI-MS spectrometry, the molecular formula of the isolated compound was assigned as C34H42O19 (Exact Mass: 754.23, Mol. Wt.: 754.69). The 1H NMR spectral data δ (500 MHz, CD3OD) were as follows: 3.30 (1H, overlapped, H-4’), 3.52 (1H, dd, J = 3.9 and 9.0 Hz, H-2’), 3.62 (1H, d, J = 12.2 Hz, H-1b), 3.65 (1H, d, J = 12.2 Hz, H-1a), 3.69 (1H, t, J = 9.0 Hz, H-3’), 3.80 (1H, overlapped, H-6b), 3.89 (3H, s, OMe), 3.90 (3H, s, OMe), 3.90 (1H, overlapped, H-6a), 4.01 (1H, m, H-5), 4.25 (1H, dd, J = 7.3 and 11.6 Hz, H-6’b), 4.33 (1H, m, H-5’), 4.55 (1H, t, J = 8.1 Hz, H-4), 4.72 (1H, dd, 1.4 and 11.6 Hz, H-6’a), 5.55 (1H, d, J = 8.1 Hz, H-3), 5.56 (1H, d, J = 2.8 Hz, H-1’), 6.49 (1H, d, J = 15.9 Hz, H-8’’’), 6.50 (1H, d, J = 15.9 Hz, H-8’’), 6.93 (2H, s, H-2’’ and H-6’’), 6.96 (2H, s, H-2’’’ and H-6’’’), 7.64 (1H, d, J = 15.9 Hz, H-7’’’) and 7.72 (1H, d, J = 15.9 Hz, H-7’’). The 13C NMR spectral data δ (125 MHz, CD3OD) were as follows: 57.6 (OMe), 57.7 (OMe), 64.7 (C-6), 66.4 (C-6’), 66.5 (C-1), 72.8 (C-4’), 73.3 (C-5’), 73.9 (C-2’), 75.0 (C-4), 75.9 (C-3’), 80.1 (C-3), 85.2 (C-5), 93.5 (C-1’), 105.7 (C-2), 105.7(C-2’’ and C-6’’), 107.9 (C-2’’’ and C-6’’’), 116.1 (C-8’’’), 116.5 (C-8’’), 127.1 (C-1’’), 127.1 (C-1’’’), 139.9 (C-4’’), 139.9 (C-4’’’), 148.1 (C-7’’’), 148.8 (C-7’’), 150.3 (C-3’’ and C-5’’), 150.3 (C-3’’’ and C-5’’’), 169.1 (C-9’’) and 169.9 (C-9’’’).
Bioassay
Ten seeds of cress (Lepidium sativum) were placed on a filter paper moistened with 500 μL of test solution in a 2.7-cm Petri dish and kept for 18 h at 23 °C in the dark, after which the length of their radicles were measured.
Determination of 3,6’-disinapoylsucrose level during gravitropic curvature
Radish seeds were germinated in moist filter paper in plastic tray in the dark at 23 °C. After 2 days 10 germinated seeds were transplanted in a row in moist vermiculite in plastic trays, and cultured in the dark at 23 °C for 1 day. The seedlings were placed horizontally in the dark at 23 °C. After onset of gravitropic stimulation, 10 hypocotyls from 0 to 1.5 cm below the hook of seedlings were harvested at 0, 30, 45, and 60 min. The hypocotyls were bisected into upper and lower halves with a razor. The peripheral cell layers (epidermis plus 1-2 parenchyma layers) were peeled off the bisected sections with forceps. All samples were immersed immediately in liquid nitrogen. Fresh weight was measured after preparation, in the frozen state. All manipulations were carried out under dim green light. Each frozen materials were homogenized in 50 mL of 80% cold acetone using homogenizer. The filtered extracts were evaporated to dryness at 40 °C in vacuo. The samples were dissolved in methanol and applied to a HPLC (ODS-120A, Wako, Japan, 4.6 x 250 mm, 0-5 min; 0% MeCN in H2O, 5-25 min; linear gradient from 0 to 100% MeCN in H2O, 1mL/min, detector at 320 nm). Retention time of 3,6’-disinapoylsucrose was 16.50 min. After the area of the peak was determined, the amount of endogenous DSS was calculated from standard curve. A known amount of isolated DSS was added to the other half of each sample during extraction in order to determine losses during the quantitation procedures. Overall recovery of DSS added to the samples was between 85 and 90%. All data have been corrected for losses. The experiment was repeated ten times.
Unilateral application of 3,6’-disinapoylsucrose
3,6’-Disinapoylsucrose isolated from large amounts of radish hypocotyls smeared with 0.5 mg lanolin at endogenous levels was unilaterally applied length wise from 0 to 2 cm below the hook of 5 uniform, 4-day-old etiolated radish seedlings. Treated seedlings were incubated in the dark at 23 °C. The hypocotyl curvature was measured at 30-min intervals using an infrared-imaging system.7 Manipulations were carried out under dim green light. The experiments were repeated three times.
ACKNOWLEDGEMENTS
This work was supported in part by a Grant-in-Aid for Young Scientist (B) (21710218) from The Ministry of Education, Culture, Sports, Science and Technology, Japan to T. H.
References
1. F. W. Went and K. V. Thimann, Phytohormones, MacMillan, New York, 1937.
2. N. Cholodny, Biol. Zent., 1927, 47, 604.
3. J. Digby and R. D. Firn, Curr. Adv. Plant Sci., 1976, 8, 953.
4. A. Müller, P. Düchting, and E. W. Weiler, Planta, 2002, 216, 44. CrossRef
5. D. J. Cosgrove, Plant Cell Environ., 1990, 13, 227. CrossRef
6. R. D. Meicenheimer and T. A. Nackid, Int. J. Plant Sci., 1994, 155, 395. CrossRef
7. K. Zandomeni and P. Schopfer, Photoplasma, 1993, 173, 103. CrossRef
8. H. Tokiwa, T. Hasegawa, K. Yamada, H. Shigemori, and K. Hasegawa, J. Plant Physiol., 2006, 163, 1267. CrossRef
9. N. De Tommasi, S. Piacente, F. De Simone, and C. Pizza, J. Nat. Prod., 1993, 56, 134. CrossRef
10. A. Bashir, M. Hamburger, J. D. Msonthi, and K. Hostettmann, Phytochemistry, 1993, 32, 741. CrossRef
11. Y. Takaya, Y. Kondo, T. Furukawa, and M. Niwa, J. Agric. Food Chem., 2003, 51, 8061. CrossRef
12. J. Bruinsma and K. Hasegawa, Physiol. Plant., 1990, 79, 700. CrossRef
13. K. Yokotani-Tomita, J. Kato, K. Yamada, S. Kosemura, S. Yamamura, J. Bruinsma, and K. Hasegawa, Physiol. Plant., 1999, 106, 326. CrossRef
14. T. Hasegawa, K. Yamada, S. Kosemura, S. Yamamura, and K. Hasegawa, Phytochemistry, 2000, 54, 275. CrossRef
15. T. Hasegawa, K. Yamada, S. Kosemura, S. Yamamura, J. Bruinsma, K. Miyamoto, J. Ueda, and K. Hasegawa, Plant Growth Regul., 2001, 33, 175. CrossRef
16. S. Yamamura and K. Hasegawa, Chem. Rec., 2001, 1, 362. CrossRef
17. T. Hasegawa, K. Yamada, H. Shigemori, K. Miyamoto, J. Ueda, and K. Hasegawa, Heterocycles, 2004, 63, 2707. CrossRef