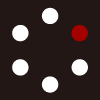
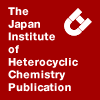
HETEROCYCLES
An International Journal for Reviews and Communications in Heterocyclic ChemistryWeb Edition ISSN: 1881-0942
Published online by The Japan Institute of Heterocyclic Chemistry
e-Journal
Full Text HTML
Received, 28th August, 2010, Accepted, 15th October, 2010, Published online, 25th October, 2010.
DOI: 10.3987/COM-10-12055
■ Asymmetric Acyl-Strecker Reaction Promoted by Novel Thiourea Organocatalyst
Takuya Kanemitsu, Eisuke Toyoshima, Michiko Miyazaki, Kazuhiro Nagata, and Takashi Itoh*
School of Pharmaceutical Sciences, Showa University, 1-5-8 Hatanodai, Shinagawa-ku, Tokyo 142-8555, Japan
Abstract
Asymmetric acyl-Strecker reaction using novel thiourea organocatalyst is described. Screening experiments of the catalysts revealed that a bifunctional organocatalyst afforded an enantio-enriched product via an unique mechanism. That is, dihydroisoquinoline derivatives were converted to a corresponding 1-cyano-1,2,3,4-tetrahydroisoquinolines using the bifunctional thiourea catalyst derived from Betti base in moderate yield and enantioselectivity.INTRODUCTION
Strecker reaction is a powerful method for the preparation of α-amino acids.1 Thus, development of asymmetric Strecker reaction has recently received much attention for the synthesis of optically active α-amino acids or their derivatives.2 Especially, organocatalytic approaches to the asymmetric reaction have been widely acknowledged.3,4 Jacobsen and co-workers described an asymmetric Strecker reaction catalyzed by a thiourea organocatalyst with excellent enantioselectivity.5 Recently, thiourea organocatalysts have been widely used in asymmetric reaction6 owing to their strong activation of imino, carbonyl or nitro groups through efficient hydrogen bonding interactions.7 We previously reported an asymmetric Strecker reaction using the Jacobsen’s catalyst for the synthesis of tetrahydroisoquinoline alkaloids.8 The Strecker reaction of 6,7-dimethoxy-3,4-dihydroisoquinoline was succeeded in high yield and high enantiomeric excess, and a 1-cyano-1,2,3,4-tetrahydroisoquinoline derivative thus obtained could be readily converted to a variety of alkaloids. One of the drawbacks of the reaction was that the Jacobsen catalyst was expensive because synthesis of the compound proceeded in multi-steps using rare L-t-leucine as a chiral source. Therefore, it was thought that more readily prepared catalyst was necessary for the present asymmetric reaction. In addition, List’s recent works described an asymmetric acyl-Strecker reaction using less toxic acetyl cyanide as a cyanide source catalyzed by thiourea catalysts.9 The result prompted us to carry out the study of asymmetric acyl-Strecker reaction of dihydroisoquinoline catalyzed by novel thiourea organocatalysts. In this paper, we report readily accessible thiourea organocatalysts, which are prepared from commercially available simple chiral amines or Betti base, and efforts to accomplish an asymmetric acyl-Strecker reaction.
RESULTS AND DISCUSSION
The study was commenced with the synthesis of new catalysts. We have designed a series of asymmetrical chiral catalysts 3a-i (Scheme 1). We planned to make thiourea catalysts bearing a bis(trifluoromethyl)phenyl group, because the substituent was reported to enhance the catalytic activity due to higher acidity of N-H group in the thiourea.10 Moreover, we decided to use chiral amines 1a-i in hope of their steric effects. Since commercially available chiral amines, (–)-1-phenylethylamine (1a), (+)-1-(1-naphthyl)ethylamine (1b), (–)-isopinocamphenylamine (1c), and (¬¬–)-cis-myrtanylamine (1d) have been used as chiral auxiliaries, they were expected to function as chirality sources of the thiourea catalyst. Betti base 1e, which can be readily prepared as an enantiopure product,11 have a phenolic hydroxyl group, which was expected to play an effective role in an asymmetric reaction. To confirm the role of the phenolic hydroxyl group, we prepared amines 1f-i derived from Betti base 1e, L-tyrosine, and L-phenylalanine, respectively. The coupling reaction of 3,5-bis(trifluoromethyl)phenyl isothiocyanate 2 and chiral amines 1a-i was carried out in CH2Cl2 at room temperature to afford corresponding chiral thiourea catalysts 3a-i in moderate to good yields (Scheme 1).
Next, the catalytic properties of a series of thiourea catalysts were examined using conditions shown in Table 1. An acyl-Strecker reaction of 6,7-dimethoxy-3,4-dihydroisoquinoline 4 with acetyl cyanide 5 was used for the screening process. The reaction was carried out by using 10 mol% of the chiral catalyst at –40 ˚C in CH2Cl2. A reaction hardly proceeded in the absence of the catalyst (Table 1, entry 1). In the cases of the thiourea catalysts 3a-d derived from commercially available chiral amines, nearly racemic products were obtained (Table 1, entries 2-5). On the other hand, catalyst 3e bearing Betti base exhibited improved enantioselectivity (Table 1, entry 6). Thus, Betti base was found to act as a chiral source of the catalytic acyl-Strecker reaction. In addition, TBDMS protection (3f) or methylation (3g) of the phenolic hydroxyl group resulted in disappearance of enantioselectivity (Table 1, entries 7 and 8). These results suggested that the phenolic hydroxyl group of the catalyst took part in the enantioselective mechanism in this reaction. Thus, the catalyst 3e was considered to be a bifunctional organocatalyst. On the contrary, neither L-tyrosine nor L-phenylalanine derivatives did afford the enantioselective product (Table 1, entries 9 and 10). These results indicated that the importance of a distance and/or an angle between thiourea and phenolic hydroxyl group for the enantioselectivity.
Although all the thiourea compounds 3a-i could be employed with comparable efficiency as catalyst, only the compound 3e did exhibit stereoselectivity. The thiourea catalyst 3e might form two hydrogen bonds with the substrates, thereby activating or coordinating the substrates in a synergistic manner (Figure 1). Namely, the asymmetric reaction was accelerated presumably through hydrogen bonds between the imine nitrogen and two thiourea hydrogens, and a hydrogen bond between oxygen of acetyl cyanide and hydrogen of phenolic hydroxyl group. Recently, Takemoto et al reported a practical result of asymmetric Michael addition of organoboronic acid using a bifunctional thiourea catalyst bearing a phenolic hydroxyl group on (1R)-trans-cyclohexanediamine, which is often used as a chiral scaffold of various thiourea catalyst.6(c) Here we described another type of chiral scaffold between a thiourea moiety and a phenolic hydroxyl group.
Encouraged by the results, we next investigated the solvent effect using 10 mol% of catalyst 3e and 1.5 equiv of acetyl cyanide 5. As shown in Table 2, all the tested solvents except for methanol, afforded the desired 1-cyanoisoquinoline 6 in low to moderate yields. Among the solvents examined, toluene was found to be the best one in term of enantioselectivity (Table 2, entry 3), whereas other aromatic solvents such as xylene and mesitylene obtained low enantioselectivity (Table 2, entries 4 and 5). Using CH2Cl2 as a solvent, the enantioselectivity of 6 dropped slightly (Table 2, entry 1). Weakly coordinating solvents, THF and Et2O gave 6 with low yields and enantioselectivity (Table 2, entries 6 and 7). Based on these results, we chose toluene as the solvent for the present reaction.
In order to improve the enantioselectivity, various additives were screened, and the results were summarized in Table 3. With trifluoroacetic acid as an additive, the enantioselectivity was improved (Table 3, entries 5-8). When 10 mol% of TFA was used, the enantioselectivity was slightly better with similar reaction yield (Table 3, entry 6). Further increase of TFA amount did not raise enantioselectivity (Table 3, entries 7 and 8). Thus, the best result was obtained when the reaction was conducted with 10 mol% of TFA in toluene using 30 mol% of catalyst 3e, giving 46% isolated yield and 67% ee.
Finally, several isoquinoline derivatives were used as substrates under the optimized reaction conditions. Results using catalyst 3e are summarized in Table 4. 3,4-dihydroisoquinoline (7) was converted into corresponding 1-cyanoisoquinoline 9 in moderate yield and enantioselectivity (Table 4, entry 3). Decrease of enantioselectivity was observed when using TFA as an additive (Table 4, entry 4). The reactions of 6,8-dimethoxy-3,4-dihydroisoquinoline 8 provided product 10 in good yields, but the enantioselectivities were low (Table 4, entries 5 and 6).
In summary, we have discovered a novel class of organocatalyst and developed an enantioselective acyl-Strecker reaction of isoquinolines. The new thiourea catalyst which has a phenolic hydroxyl group was simple in structure and prepared easily from a chiral amine, Betti base. The phenolic hydroxyl group played an important role for the enantioselectivity. This is the first example of acyl-cyanayion of cyclic imines. The results indicated that simple and practical thiourea catalysts might be found by the combination with chiral amines which have phenolic hydroxyl group. Further study on the mechanistic aspect and the improvement of the ees are currently under investigation.
EXPERIMENTAL
Unless otherwise noted, materials were purchased from commercial suppliers and used without further purification. Progress of the reactions was monitored by thin layer chromatography (TLC) performed on Merck Art. 5715 Kieselgel 60 F254/0.25 mm thickness plates. Visualization was accomplished with UV light and cerium sulfate-ammonium molybdate solution followed by heating. Column chromatography was performed using forced flow of the indicated solvent on Sigma H-type silica Gel 60N (100-210 µm). 1H- and 13C-NMR spectra were recorded with JEOL JNM AL-400 instrument (400 MHz for 1H and 100 MHz for 13C) in deuterated solvent, using tetramethylsilane (δ = 0.0 ppm in 1H-NMR spectra) and CDCl3 (δ = 77.0 ppm in 13C-NMR spectra) as internal standards. 1H data were reported as follows: chemical shift (δ in ppm), multiplicity (s = singlet, d = doublet, t = triplet, dd = doublet of doublets, m = multiplet) and coupling costant (J in Hz). Optical rotations were measured on a JASCO P1020 polarimeter operating at the sodium D line at room temperature. Concentration is given in g/100 mL. The HPLC analyses were performed with Shimadzu equipment (254 λ absorbance Detector) using Daicel Chemical Industries, LTD. CHIRALPAK IA column with hexane/ethyl acetate mixtures (composition and flow rate as indicated). HPLC methods were calibrated with the corresponding racemic mixtures. High-resolution mass spectra (HR-FAB MS) were measured with JEOL JMS-MS700V using p-nitrobenzyl alcohol as a matrix. The known compounds have been identified by comparison of spectral data with those reported. The absolute configuration of the optically active compound 6 was determined after conversion into methyl ester through acid-mediated hydrolysis and hydrogenolysis9(c) on the basis of the measured optical rotation compared with literature values.8(a) In the case of compound 9 and 10, the absolute configurations were not determined.
Typical procedure for the synthesis of catalysts 3. To a solution of chiral amine (1.0 mmol) in CH2Cl2 (3 mL) was added dropwise 3,5-bis(trifluoromethyl)phenyl isothiocyanate (1.0 mmol) at rt under an argon atmosphere. After stirring the reaction mixture for 24 h at rt, the solvent was removed under reduced pressure and the resulting residue was recrystallized from CH2Cl2/hexane to provide 3.
Thiourea catalyst 3a: 83% yield; white crystals; mp 136-137℃; [α]18D +14.0 (c 0.70, CHCl3); 1H-NMR (400 MHz, CDCl3, 24.5℃) δ 7.86 (bs, 1H), 7.65 (s, 3H), 7.42-7.35 (m, 5H), 6.57 (bs, 1H), 5.39 (bs, 1H), 1.60 (d, J = 6.8 Hz, 3H); 13C-NMR (100 MHz, CDCl3, 24.5℃) δ 179.9, 141.3, 139.1,132.7 (q, JCF = 34.6 Hz), 129.3, 128.4, 126.1, 123.9, 122.8 (q, JCF = 271.7 Hz), 119.4, 54.8, 22.2; HR-FAB MS: Calcd for C17H15F6N2S [M+H]+: 393.0860. Found 393.0872.
Thiourea catalyst 3b: 82% yield; white crystals; mp 130-131℃; [α]18D –46.1 (c 0.69, CHCl3); 1H-NMR (400 MHz, CDCl3, 23.8℃) δ 8.15 (d, J = 8.4 Hz, 1H), 7.92-7.84 (bs, 1H), 7.91 (d, J = 8.0 Hz, 1H), 7.85 (d, J = 8.0 Hz, 1H), 7.63-7.45 (m, 7H), 6.24 (bs, 2H), 1.80 (d, J = 6.8 Hz, 3H); 13C-NMR (100 MHz, CDCl3, 23.7℃) δ 138.8, 136.3, 134.1, 132.8 (q, JCF = 31.4 Hz), 130.8, 129.2, 127.2, 126.3, 125.3, 123.8, 123.8, 123.8, 122.9, 122.6 (q, JCF = 271.4 Hz), 119.3, 51.0, 19.0; HR-FAB MS: Calcd for C21H17F6N2S [M+H]+: 443.1017. Found 443.0986.
Thiourea catalyst 3c: 70% yield; colorless needles; mp 137-138℃; [α]18D –17.5 (c 0.69, CHCl3); 1H-NMR (400 MHz, CDCl3) δ 8.64 (s, 1H), 7.76 (s, 2H), 7.71 (s, 1H), 6.22 (s, 1H), 3.62 (s, 2H), 2.42-2.29 (m, 2H), 2.00-1.85 (m, 5H), 1.56-1.48 (m, 1H), 1.19 (s, 3H), 1.04 (s, 3H), 0.91 (d, J = 9.8 Hz, 1H); 13C-NMR (100 MHz, CDCl3) δ 180.4, 138.8, 133.2 (q, JCF = 38.1 Hz), 123.9, 122.7 (q, JCF = 273.1 Hz), 119.4, 51.0, 43.8, 41.2, 40.7, 38.6, 33.1, 27.8, 25.8, 23.1, 19.7; HR-FAB MS: Calcd for C19H23F6N2S [M+H]+: 425.1486. Found 425.1488.
Thiourea catalyst 3d: 71% yield; colorless needles; mp 197-198℃; [α]18D –20.3 (c 0.67, CHCl3); 1H-NMR (400 MHz, CDCl3) δ 8.53 (s, 1H), 7.77 (s, 2H), 7.69 (s, 1H), 6.15 (s, 1H), 4.79 (s, 1H), 2.78 (t, J = 12.0 Hz, 1H), 2.46-2.40 (m, 1H), 2.00-1.99 (m, 1H), 1.85 (t, J = 5.6 Hz, 1H), 1.79 (s, 1H), 1.57 (s, 1H), 1.24 (s, 3H), 1.19 (d, J = 7.1 Hz, 3H), 1.06 (s, 3H), 0.77 (d, J = 9.5 Hz, 1H); 13C-NMR (100 MHz, CDCl3) δ 179.9, 139.0, 133.0 (q, JCF = 31.4 Hz), 123.7, 122.7 (q, JCF = 273.1 Hz), 119.2, 54.1, 47.5, 46.0, 41.3, 38.4, 36.4, 35.3, 27.8, 23.3, 20.6; HR-FAB MS: Calcd for C19H23F6N2S [M+H]+: 425.1486. Found 425.1462.
Thiourea catalyst 3e: 84% yield; white crystals; mp 139-140℃, [α]18D +14.4 (c 0.71, CHCl3); 1H-NMR (500 MHz, CD2Cl2) δ 8.29 (d, J = 5.8 Hz, 1H), 8.17 (s, 1H), 8.14 (bs, 1H), 7.94 (d, J = 5.7 Hz, 1H), 7.84 (d, J = 6.9 Hz, 1H), 7.80 (d, J = 9.2 Hz, 1H), 7.72 (s, 2H), 7.71 (s, 1H), 7.50 (t, J = 7.5 Hz, 1H), 7.39 (t, J = 7.2 Hz, 1H), 7.23-7.18 (m, 5H), 7.07 (d, J = 8.6 Hz, 1H), 5.76 (bs, 1H); 13C-NMR (125 MHz, CD2Cl2) δ 180.7, 151.5, 140.6, 139.0, 133.1 (q, JCF = 33.6 Hz), 132.8, 130.9, 130.1, 129.2, 128.9, 128.1, 127.7, 126.6, 124.5, 124.3, 123.3 (q, JCF = 271.0 Hz), 123.3, 119.8, 119.2, 118.6, 54.0; HR-FAB MS: Calcd for C26H19F6N2OS [M+H]+: 521.1122. Found 521.1116.
Thiourea catalyst 3f: 50% yield; yellow amorphous; mp 85-87℃; [α]18D +40.6 (c 0.69, CHCl3); 1H-NMR (400 MHz, CD3OD, 24.8℃) δ 7.96 (s, 2H), 7.72 (s, 1H), 7.60-7.50 (m, 4H), 7.36 (s, 1H), 7.11-6.92 (m, 9H), 0.90 (s, 9H), 0.25 (s, 3H), 0.21 (s, 3H); HR-FAB MS: Calcd for C32H33F6N2OSSi [M+H]+: 635.1987. Found 635.2012.
Thiourea catalyst 3g: 95% yield; yellow amorphous; mp 88-90℃; [α]18D –34.0 (c 0.69, CHCl3); 1H-NMR (400 MHz, CDCl3, 22.8℃) δ 8.83 (bs, 1H), 8.36 (d, J = 8.4 Hz, 1H), 8.35 (bs, 1H), 8.06 (d, J = 9.2 Hz, 1H), 7.87 (d, J = 8.8 Hz, 1H), 7.82 (d, J = 8.4 Hz, 1H), 7.54 (t, J = 7.4 Hz, 1H), 7.40 (t, J = 7.6 Hz, 1H), 7.25-7.15 (m, 6H), 3.47 (s, 3H); 13C-NMR (100 MHz, CDCl3, 22.8℃) δ 179.9, 155.0, 140.9, 138.5, 133.1 (q, JCF = 34.6 Hz), 132.3, 130.5, 129.6, 128.6, 128.3, 127.8, 127.0, 125.8, 124.4, 123.6, 123.2, 122.8 (q, JCF = 271.7 Hz), 122.1, 119.3, 114.2, 56.5, 55.7; HR-FAB MS: Calcd for C27H21F6N2OS [M+H]+: 535.1279. Found 535.1252.
Thiourea catalyst 3h: 50% yield; colorless amorphous; mp 95-97℃; [α]18D +18.6 (c 0.69, CHCl3); 1H-NMR (400 MHz, CDCl3, 22.8℃) δ 9.04 (s, 1H), 8.79 (s, 1H), 7.91 (s, 2H), 7.43 (s, 1H), 7.17-7.11 (m, 7H), 6.76-6.70 (m, 2H), 5.61 (bs, 1H), 5.50 (q, J = 7.2 Hz, 1H), 4.84 (d, J = 15.2 Hz, 1H), 4.32 (d, J = 14.8 Hz, 1H), 3.15 (ddd, J = 4.6, 13.6, 21.0 Hz, 2H), 2.94 (s, 3H); 13C-NMR (100 MHz, CDCl3, 23.1℃) δ 181.4, 174.6, 155.1, 139.8, 135.3, 131.1 (q, JCF = 33.3 Hz), 130.5, 128.7, 127.5, 127.4, 127.3, 124.1, 123.0 (q, JCF = 271.4 Hz), 118.0, 115.7, 56.6, 52.2, 37.7, 35.3; HR-FAB MS: Calcd for C26H24F6N3O2S [M+H]+: 556.1493. Found 556.1524.
Thiourea catalyst 3i: 69% yield; colorless amorphous; mp 60-62℃; [α]18D +13.5 (c 0.67, CHCl3); 1H-NMR (400 MHz, CDCl3, 22.9℃) δ 9.28 (s, 1H), 8.80 (s, 1H), 7.96 (s, 2H), 7.41 (s, 1H), 7.36-7.29 (m, 5H), 7.15-7.10 (m, 5H), 5.55 (q, J = 7.1 Hz, 1H), 4.88 (d, J = 14.4 Hz, 1H), 4.30 (d, J = 15.2 Hz, 1H), 3.26 (ddd, J = 7.7, 13.3, 24.3 Hz, 2H), 2.92 (s, 3H); 13C-NMR (100 MHz, CDCl3, 23.6℃) δ 181.6, 174.8, 139.9, 135.4, 135.3, 131.0 (q, JCF = 33.5 Hz), 129.3, 128.9, 128.7, 127.7, 127.6, 127.5, 124.1, 123.1 (q, JCF = 271.7 Hz), 117.9, 56.6, 52.3, 38.7, 35.2; HR-FAB MS: Calcd for C26H24F6N3OS [M+H]+: 540.1544. Found 540.1560.
General procedure for the acyl-Strecker reaction. To a solution of 6,7-dimethoxy-3,4-dihydroisoquinoline (1 mmol) and catalyst 3 in CH2Cl2 (10 mL) was added dropwise acetyl cyanide (1 mmol) at –40˚C under an argon atmosphere. After stirring the reaction mixture for 48 h at –40˚C, the reaction mixture was directly purified though column chromatography on silica gel (hexane-ethyl acetate, 6:4) to provide 6.
Compound 6: white solid; mp 204-205℃, [α]24D –101.3 (c 0.45, CHCl3, 67% ee); HPLC tR (minor: R): 16.8 min; tR (major: S): 19.5 min [Chiralpak IA (0.46 cm x 25 cm, Daicel Chemical Ind., Ltd.), ethyl acetate/hexane, 2/3, 0.5 mL/min]; 1H NMR (CDCl3) δ 6.77 (s, 1 H), 6.65 (s, 1 H), 6.41 (s, 1 H), 3.96 (ddd, 1 H, J = 4.3, 4.3, 13.5 Hz), 3.89 (s, 3 H), 3.88 (s, 3 H), 3.67 (ddd, 1 H, J = 4.3, 10.7, 13.7 Hz), 2.95 (ddd, 1 H, J = 5.4, 10.6, 16.0 Hz), 2.86 (ddd, 1 H, J = 3.8, 3.8, 15.8 Hz), 2.23 (s, 3 H); 13C NMR (CDCl3) δ 169.5, 149.5, 148.6, 125.9, 120.1, 118.0, 111.4, 109.5, 56.1, 56.0, 43.2, 42.1, 28.1, 21.3; HR-FAB MS: Calcd for C14H17N2O3 [M+H]+: 261.1239. Found 261.1227.
Compound 9: white solid; mp 112-114℃, [α]24D –96.6 (c 0.1, CHCl3, 62% ee); HPLC tR (minor): 24.9 min; tR (major): 26.8 min [Chiralpak IA (0.46 cm x 25 cm, Daicel Chemical Ind., Ltd.), ethyl acetate/hexane, 1/3, 0.5 mL/min]; 1H NMR (CDCl3) δ 7.33-7.21 (m, 4 H), 6.48 (s, 1 H), 3.96 (dt, 1 H, J = 4.7, 13.3 Hz), 3.72 (ddd, 1 H, J = 5.5, 9.6, 12.4 Hz), 3.02-2.99 (m, 2 H), 2.23 (s, 3 H); 13C NMR (CDCl3) δ 169.6, 133.8, 129.2, 129.0, 128.6, 127.6, 127.4, 117.8, 43.5, 42.2, 28.5, 21.3; HR-FAB MS: Calcd for C28H31N2OS [M+H]+: 201.1028. Found 201.1037.
Compound 10: white solid; mp 107-109℃, [α]24D –27.1 (c 0.1, CHCl3, 29% ee); HPLC tR (minor): 25.8 min; tR (major): 33.4 min [Chiralpak IA (0.46 cm x 25 cm, Daicel Chemical Ind., Ltd.), ethyl acetate/hexane, 1/3, 0.5 mL/min]; 1H NMR (CDCl3) δ 6.48 (s, 1 H), 6.36 (d, 1 H, J = 2.4 Hz), 6.29 (d, 1 H J = 1.6 Hz), 3.96 (dt, 1 H, J = 4.6, 13.7 Hz), 3.88 (s, 3 H), 3.80 (s, 3 H), 3.67 (ddd, 1 H, J = 4.6, 10.6, 14.0 Hz), 2.95 (ddd, 1 H, J = 5.4, 10.6, 16.2 Hz), 2.87 (dt, 1 H, J = 3.8, 12.7 Hz), 2.21 (s, 3H); 13C NMR (CDCl3) δ 169.3, 161.0, 157.4, 135.7, 117.8, 109.9, 104.5, 97.0, 55.7, 55.4, 41.9, 39.5, 28.7, 21.3; HR-FAB MS: Calcd for C14H17N2O3 [M+H]+: 261.1239. Found 261.1210.
ACKNOWLEDGEMENTS
This work was supported in part by a Grant for the High-Tech Research Center Project from the Ministry of Education, Culture, Sports, Science and Technology of Japan.
References
1. A. Strecker, Ann. Chem. Pharm., 1850, 75, 27. CrossRef
2. For recent reviews on the asymmetric Strecker reactions, see: (a) Y. Takemoto, Org. Biomol. Chem., 2005, 3, 4299; CrossRef (b) A. G. Doyle and E. N. Jacobsen, Chem. Rev., 2007, 107, 5713; CrossRef (c) S. J. Connon, Angew. Chem. Int. Ed., 2008, 47, 1176; CrossRef (d) P. Merino, E. Marqués-López, T. Tejero, and R. P. Herrera, Tetrahedron, 2009, 65, 1219; CrossRef (e) J. Martens, ChemCatChem, 2010, 2, 379. CrossRef
3. For recent organocatalytic Strecker reactions, see: (a) C. Becker, C. Hoben, and H. Kunz, Adv. Synth. Catal., 2007, 349, 417; CrossRef (b) M. Rueping, E. Sugiono, and S. A. Moreth, Adv. Synth. Catal., 2007, 349, 759; CrossRef (c) M. Negru, D. Schollmeyer, and H. Kunz, Angew. Chem. Int. Ed., 2007, 46, 9339; CrossRef (d) Z. Hou, J. Wang, X. Liu, and X. Feng, Chem. Eur. J., 2008, 14, 4484; CrossRef (e) Y. Wen, B. Gao, Y. Fu, S. Dong, X. Liu, and X. Feng, Chem. Eur. J., 2008, 14, 6789; CrossRef (f) F. Cruz-Acosta, A. Santos-Expósito, P. de Armas, and F. García-Tellado, Chem. Commun., 2009, 6839; CrossRef (g) G.-W. Zhang, D.-H. Zheng, J. Nie, T. Wang, and J.-A. Ma, Org. Biomol. Chem., 2010, 8, 1399. CrossRef
4. For recent reviews of organocatalysts, see: (a) D. W. C. MacMillan, Nature, 2008, 455, 304; CrossRef (b) K. N. Houk and P. H.-Y. Cheng, Nature, 2008, 455, 323; CrossRef (c) X. H. Yu and W. Wang, Chem. Asian J., 2008, 3, 516; CrossRef (d) V. Caprio and J. M. J. Williams, Catalysis in Asymmetric Synthesis, 2nd ed: John Wiley and Sons, Ltd.: Chichester. 2009; (e) S. J. Connon, Synlett, 2009, 354; CrossRef (f) L.-W. Xu, J. Luo, and Y. Lu, Chem. Commun., 2009, 1807; CrossRef (g) X. Liu, L. Lin, and X. Feng, Chem. Commun., 2009, 6145; CrossRef (h) C. Grondal, M. Jeanty, and D. Enders, Nat. Chem., 2010, 2, 167; CrossRef (i) Y. Takemoto, Chem. Pharm. Bull., 2010, 58, 593. CrossRef
5. (a) M. S. Sigman and E. N. Jacobsen, J. Am. Chem. Soc., 1998, 120, 4901; CrossRef (b) M. S. Sigman, P. Vachal, and E. N. Jacobsen, Angew. Chem. Int. Ed., 2000, 39, 1279; CrossRef (c) P. Vachal and E. N. Jacobsen, Org. Lett., 2000, 2, 867; CrossRef (d) P. Vachal and E. N. Jacobsen, J. Am. Chem. Soc., 2002, 124, 10012; CrossRef (e) A. G. Wenzel, M. P. Lalonde, and E. N. Jacobsen, Synlett, 2003, 1919; CrossRef (f) M. S. Taylor and E. N. Jacobsen, Angew. Chem. Int. Ed., 2006, 45, 1520; CrossRef (g) S. J. Zuend and E. N. Jacobsen, J. Am. Chem. Soc., 2007, 129, 15872; CrossRef (h) S. J. Zuend, M. P. Coughlin, M. P. Lalonde, and E. N. Jacobsen, Nature, 2009, 461, 96. CrossRef
6. For selected recent thiourea organocatalystic reactions, see: (a) X.-Q. Dong, H.-L. Teng, and C.-J. Wang, Org. Lett., 2009, 11, 1265; CrossRef (b) Q. Zhu and Y. Lu, Org. Lett., 2009, 11, 1721; CrossRef (c) T. Inokuma, K. Takasu, T. Sakaeda, and Y. Takemoto, Org. Lett., 2009, 11, 2425; CrossRef (d) K. Mei, M. Jin, S. Zhang, P. Li, W. Liu, X. Chen, F. Xue, W. Duan, and W. Wang, Org. Lett., 2009, 11, 2864; CrossRef (e) X. Wang, Y.-F. Chen, L.-F. Niu, and P.-F. Xu, Org. Lett., 2009, 11, 3310; CrossRef (f) T. Bui, S. Syed, and C. F. Barbas, III, J. Am. Chem. Soc., 2009, 131, 8758; CrossRef (g) D. R. Li, A. He, and J. R. Falck, Org. Lett., 2010, 12, 1756; CrossRef (h) X.-L. Liu, Y.-H. Liao, Z.-J. Wu, L.-F. Cun, X.-M. Zhang, and W.-C. Yuan, J. Org. Chem., 2010, 75, 4872; CrossRef (i) R. R. Knowles, S. Lin, and E. N. Jacobsen, J. Am. Chem. Soc., 2010, 132, 5030; CrossRef (j) A. K. Basak, N. Shimada, W. F. Bow, D. A. Vicic, and M. A. Tius, J. Am. Chem. Soc., 2010, 132, 8266. CrossRef
7. (a) C. M. Kleiner and P. R. Schreiner, Chem. Commun., 2006, 4315; CrossRef (b) A. Hamza, G. Schubert, T. Soós, and I. Pápai, J. Am. Chem. Soc., 2006, 128, 13151; CrossRef (c) T. Weil, M. Kotke, C. M. Kleiner, and P. R. Schreiner, Org. Lett., 2008, 10, 1513. CrossRef
8. (a) T. Kanemitsu, Y. Yamashita, K. Nagata, and T. Itoh, Synlett, 2006, 1595; CrossRef (b) T. Kanemitsu, Y. Yamashita, K. Nagata, and T. Itoh, Heterocycles, 2007, 74, 199. CrossRef
9. (a) S. C. Pan, J. Zhou, and B. List, Synlett, 2006, 3275; CrossRef (b) S. C. Pan and B. List, Synlett, 2007, 318; CrossRef (c) S. C. Pan, J. Zhou, and B. List, Angew. Chem. Int. Ed., 2007, 46, 612; CrossRef (d) S. C. Pan and B. List, Org. Lett., 2007, 9, 1149; CrossRef (e) S. C. Pan and B. List, Chem. Asian. J., 2008, 3, 430. CrossRef
10. A. Wittkopp and P. R. Schreiner, Chem. Eur. J., 2003, 9, 407. CrossRef
11. (a) M. Betti, Gazz. Chim. Ital., 1900, 30, II-310; (b) M. Betti, Org. Synth. Coll., 1941, I, 381; (c) H. E. Smith and N. E. Cooper, J. Org. Chem., 1970, 35, 2212; CrossRef (d) I. Szatmári and F. Fülöp, Curr. Org. Syn., 2004, 1, 155; CrossRef (e) Y. Dong, R. Li, J. Lu, X. Xu, X. Wang, and Y. Hu, J. Org. Chem., 2005, 70, 8617; CrossRef (f) V. A. Alfonsov, K. E. Metlushka, C. E. McKenna, B. A. Kashemirov, O. N. Kataeva, V. F. Zheltukhin, D. N. Sadkova, and A. B. Dobrynina, Synlett, 2007, 488. CrossRef