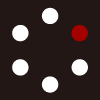
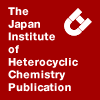
HETEROCYCLES
An International Journal for Reviews and Communications in Heterocyclic ChemistryWeb Edition ISSN: 1881-0942
Published online by The Japan Institute of Heterocyclic Chemistry
e-Journal
Full Text HTML
Received, 9th September, 2010, Accepted, 25th October, 2010, Published online, 4th November, 2010.
DOI: 10.3987/COM-10-12065
■ Synthesis and Spectroscopic Characterization Study of Some Cyclic-Azodioxides
Mohammad A. Naser Al-Magmoy,* Zeki A. Nasir Al-Shamkhani, and Ali Hashem Essa
Department of Chemistry, College of Science, University of Basrah, Basrah, Iraq
Abstract
New compounds of cyclic-azodioxides have been prepared by oxidation of the corresponding diamines with sodium tungstate and hydrogen peroxide in a mixed ethanol/water solvent. Spectroscopic techniques, including IR, UV, 1H NMR, and 13C NMR, and CHN analysis were used to identify the products. In solution, the azodioxides were found to be in equilibrium with the corresponding dinitroso compounds. The IR values of the synthesised compounds are in agreement with the theoretical IR spectra.INTRODUCTION
In the past several years, although much attention has been paid to the development of methods for preparation of organic azodioxide due to their broad utility, as units for many biologically active compounds and as important chiral ligands or chiral auxiliaries for asymmetric synthesis. 1,3-dipolar cycloaddition reactions were used to synthesize compounds having interesting pharmacological activities and for the preparation of many stereochemically defined five-membered heterocycles.1–12 Due to their importance, we report herein the preparation of new nitroso dimers by using sodium tungstate - hydrogen peroxide. This method has the advantage of facilitating the oxidation of the nitroso compounds in one step.
Many organic nitroso compounds (R-NO) in organic solvent, where the NO group is bonded to a carbon atom (C-nitroso compound), display blue or blue green colors, due to a weak absorption band in the visible region (λ =700 nm) in the crystalline state. However, most of these compounds are colorless or at most pale yellow. The color intensity of a solution of C-nitroso compounds depends on the nature of the organic group (R), temperature and concentration. The reason of this phenomenon is based on cryoscopic molecular weight measurements. Faintly colored (R-NO) solution contains significant proportion of dimeric molecules in equilibrium with monomers. 1
It is well known that C-nitroso compounds can exist in three different molecular forms: the monomer R-N = O, the trans-dimer (A) and the cis-dimer (B). The relative stabilities of these forms are function of the state, the properties of solvents in which they are dissolved, and the molecular environment within which the nitroso function are located. Examples of their functions are demonstrated by nitroso butane which is in solid state in the cis dimer,1,2 whereas in the solution or gas phase, it is 100% monomer, i.e., the monomers are green – blue in color and the dimers are colorless. Some dimers (azodioxides) exist in cyclic configuration especially the cis-dimers such as 1,4-dichloro-2,3-diazabicyclo [2.2.2]-oct-2-ene 2,3-dioxide (C) and benzo[c]cinnoline-5,6-dioxide (D), which are limited for steric reason to internal dimerization of the nitroso groups in the cis configuration.3, 4
Various methods have been reported regarding the synthesis of aliphatic and aromatic C-Nitroso compounds. Some of these compounds were prepared by oxidation of the corresponding amines but most of these compounds are obtained by oxidation of corresponding hydroxyl amine or imine,5 by pyrolysis of the corresponding aryl nitrite or by action of the nitric oxide and chlorine hydrocarbons under UV light.
RESULTS AND DISCUSSION
Schemes 1, 2, and 3 illustrated the diagram of synthesis of three new dioxides (compounds 1-3 respectively).
These dimers are white crystalline solids that dissolve in organic solvents to give nearly colorless solution developing to a blue color, which is temperature dependent, and on cooling, the solution losses its color. This indicates that these solids are dimers in solution and dissociate to their monomer forms by heating as shown in Scheme 4.
These compounds were not isomerisd13 neither by aqueous sodium hydroxide (1N) nor by hydrochloric acid (1N).
In this research were described the method of synthesis of aryl amine C-nitroso dimers by the direct oxidation of the primary amines with sodium tungstate – hydrogen peroxide. When recrystallization was carried out, the yield was reduced to 20% whether the reaction was carried out in a small or a large scale.
The existence of compounds (1-3) in monomer or dimer forms was proved by studying its electronic and infrared spectra. The electronic absorption spectra recorded in chloroform at room temperature showed two (π-π*) transition bands. The transition bands at about 290 nm was ascribed to the dimer grouping (N2O2), and the other transition around 250 nm is ascribable to the double bond of the aryl residual. There is no trace of monomeric (n-π*) band. A comparison between the electronic configuration of the monomers and that of dimers suggests that dimerization process requires redistribution of electrons in the R-NO subunit. Experimental evidence, indicating that this indeed happens. The color of monomers in solution might be due to a weak (n-π*) transition which involves the electron lone pair predominantly localized on the oxygen atom. This (n-π*) transition disappears on dimerization because the nitrogen lone pair in the monomer is used to form the (N-O) bond, as in compounds (1-3) for example.
Considering the infrared spectra which were recorded at room temperature, many workers,14, 15 assigned (N-O) stretching frequency in trans and cis-nitroso compounds. In cis- form this was reported to be a strong double band absorption in the 1180-1320 cm-1 range, whilst trans form exhibit a single strong stretching absorption in the same range. The assignment of IR spectra of the cis and trans CN(O)N(O)C grouping can be made according to the group theory.
All IR spectra indicate that the five strong bands between 900-3010 cm-1 in these compounds are correspond to five vibrations characteristic of the skeleton, though it is possible that some features of the aryl group have been included. The basic skeleton of the cis CN(O)N(O)C has C2v symmetry; the A1 class includes the (N-O), (C-N) and the (N-N) stretching modes. Also the B1 class with the symmetric (N-O) and (C-N) stretching contain infrared active vibration. The trans dimer skeleton has essentially C2h symmetry with two strong B2 class frequencies at about 900 cm-1. These may be considered as essentially antisymmetric (N-O) and (C-N) stretching vibrations.
The (N-O) bonds may have partial double bond character and are strongly polar. The IR values of the synthesised compounds are in agreement with the theoretical IR spectra as shown in Table 1. Theoretical IR spectra of the studied molecules calculated by semi-empirical PM3 method, are presented in Figure 1.
The small, but easily distinguishable difference of 1H NMR and 13C NMR spectra of cis and trans dimers16, 17 arises from the same symmetry arguments as above.
The conformation of compounds (1, 2, 3) was determined by analysis of the proton resonance signals. Spin-spin coupling constant of the protons attached to C atoms close to the nitroso group are used for determination of this group’s conformation. In particular, those attach to the carbon atoms close to the nitroso groups. The small spin-spin coupling constant of 5.3 Hz indicates that the nitroso groups adopts cis arrangement.
13C NMR spectra of compound 3 showed eight lines while seven lines were recorded for compounds (1 and 2), in addition to the lines of the solvents. This spectroscopy has yielded interesting structural information for C-Nitroso compound, and the large difference ( >20ppm) between the resonance for C-NO monomer and C-N2O2-C dimmer clearly permits an estimate to be made for any participation of monomeric NO end groups in the solid.13
CONCLUSIONS
The method of synthesis of aryl amine C-nitroso dimers by the direct oxidation of primary amines with sodium tungstate – hydrogen peroxide has been described in this research. New compounds of cyclic-azodioxides have been prepared according to this method but when recrystallization was carried out, the yield was reduced to 20%. The IR values of the synthesized compounds are in agreement with the theoretical spectra.
EXPERIMENTAL
1) general preparation of (E)-11,12-dihydrodibenzo[c,g][1,2]diazocine-5,6-dioxide (1)
2,2'-(Ethane-1,2-diyl)dianiline was prepared by catalytic reduction of the dinitro compound18 mp 65-66 °C (lit., mp 66-67 °C). E-11,12-Dihydrodibenzo[c,g][1,2]diazocine-5,6-dioxide) was prepared in the dark from cooled solution of 2,2'-(ethane-1,2-diyl)dianiline (1 g, 0.0047 mol) and sodium tungstate dehydrate (0.2 g) in (20 mL) of 85% EtOH/water containing hydrogen peroxide (2 g, 60%). The mixture was stirred for 24 h and then 25 mL of saturated aqueous NaCl along with equivalent volume of CH2Cl2 was added. The aqueous layer was extracted with a small portion of CH2Cl2, and the resulting oil was taken up in a acetone and cooled to give (0.1 g, 19%); the diazocine dioxide; mp 155 oC (decomp). Rf = 0.73. Anal. Calcd for C14H12N2O2: C, 69.98; H, 5.04; N, 11.66. Found :C,70.01; H,4.97; N, 11.3. UV (CHCl3) λmax, 292 (ε = 9800) and 250 nm (ε = 7870); IR υ (KBr), 3010, 2995, 2980, 1440, 1410, 1385, 1305, 1200, 1175, 1110, 950, 770 cm-1. 1H NMR (400 MHz) δH (ppm) (benzene-d6), 7.1-7.5 m (4H); 2.9-3.2 s (2H). 13C NMR (100 MHz) δC (ppm) ( benzene-d6), 142.6; 125.9; 138.9; 130.0; 128.3; 119.7; 98.3.
2-general preparation of (Z)-11,13-dihydrodibenzo[c,g][1,5,6]oxadiazonine 5,6-dioxide) (2)
2-1 Preparation of 2,2'-oxybis(methylene)bis(nitrobenzene)
The mixture of (2-nitrophenyl)methanol19 (2 g, 0.013 mol), 1-(chloromethyl)-2-nitrobenzene) (4 g, 0.023 mol) and silver oxide (3.5 g) was added to a mixture of 50 mL of dry DMF and DMSO (50 mL). The solution was heated on a steam bath, filtered and recrystallized from CHCl3 to yield white crystals (2.5 g) mp 160-1661 °C. Anal. Calcd for C14H12N2O5: C, 58.33; H, 4.20; N, 9.72. Found C, 57.98; H, 4.84; N, 10.01. IR υ (KBr), 3020, 2930, 2880, 1535, 1515, 1370, 1360, 1212, 870, 790, 725 cm-1.
2-2 Preparation of 2,2'-oxybis(methylene)dianiline
A solution of 2,2'-oxybis(methylene)bis(nitrobenzene) (2 g, 0.007 mol) and tin metal (1 g) with 10% hydrochloric acid in EtOH (100 mL) was stirred. Hydrazine hydrate (2 g) was added to the solution and stirred for 15 min at rt and then reflex for 1h. The cooled solution was filtered and the resultant yellow oil was taken up in Et2O and extracted with salted water. The ether was evaporated, and the collected product was recrystallized from CHCl3 to yield the product (1.7 g, 85%) mp 67-69 °C. Anal. Calcd for C14H16N2O: C, 73.68; H, 7.01; N, 12.28. Found C, 73.08; H, 7.35; N, 12.83. IR, υ (KBr), 3460, 3380, 3010, 1493, 1036 cm-1.
2-3 Preparation of (Z)-11,13-dihydrodibenzo[c,g][1,5,6]oxadiazonine 5,6-dioxide (2)
A suspension of 2,2'-oxybis(methylene)bis(nitrobenzene) (1 g, 0.005 mol) and sodium tungstate dihydrate (0.2 g) in 19 mL of 85% EtOH/water was added to hydrogen peroxide (3 g, 60%) dropwise. The mixture was stirred for (24 h) at rt and poured into 5% HCl and extracted with three portions of CHCl3. The extract was evaporated, the resulting residue treated briefly in 20 mL of acetone and allowed to cool to yield oxadiazonine 5,6-dioxide (0.25 g, 25%), mp 123 °C. Anal. Calcd for C14H12N2O3: C, 65.61; H, 4.72; N, 10.13. Found C, 65.01; H, 4.5; N, 10.38. UV absorption in CHCl3 λmax 285 (ε = 8980) and 248 nm (ε =7810). IR υ (KBr), 3010, 1428, 1380, 1290, 1212, 1109, 1040, 765 cm-1. 1H NMR (400 MHz) δH (ppm) (DMSO d6), 7.38-7.70 m (4H); 4.52-4.72 s (2H). 13C NMR (100 MHz) δC (ppm) (Me2SO d6), 148.3; 126.7; 135.4; 128.2; 126.5; 115.3; 100.1.
3-general preparation of (5Z,12E)-11H-dibenzo[c,h][1,2,6]triazonine 5,6-dioxide (3)
3-1 Preparation of (E)-N-(2-nitrobenzylidene)-1-(2-nitrophenyl)methanamine
A solution of benzene (100 mL) containing of the 2-nitrophenyl)methanamine20 (2 g, 0.013mol.) and 2-nitrobenzaldehyde (2 g, 0.013 mol.) was refluxed in Dean-Stark apparatus for 2 h. After evaporation of solvent, the solid products was collected and recrystallized from EtOH to give 3 (3.5 g, 89%), mp 154-155 °C. Anal. Calcd for C14H11N3O4:- C, 58.94; H, 3.86; N, 14.73. Found C, 59.13; H, 3.33; N, 14.18. IR: - υ (KBr), 1640, 1610, 1574, 1513, 1382, 1345, 1325, 849, 793, 732 cm-1.
3-2 Preparation of (E)-2-((2-aminobenzylideneamino)methyl)aniline
Dry hydrogen chloride gas was bubbled into a solution of (E)-N-(2-nitrobenzylidene)-1-(2-nitrophenyl)methanamine (3 g, 0.01 mol.) in 100 mL of THF. The product was cooled to rt and stirred for 30 min with dry MeOH. The solvent was removed under vacuum. CH2Cl2 was add to the residue and extracted with aqueous NaOH (150 mL, 0.1 N), the organic fraction was dried by using (MgSO4), concentrated and recrystallized from EtOH to give the diamine (2.7 g, 92%), mp 98-100 °C. Anal. Calcd. for C14H15N3, C,74.66; H,6.66; N, 18.66. Found C, 73.97; H, 6.21; N, 19.02. IR :- υ (KBr), 3380, 3290, 1612, 1575, 1515, 1346, 1867, 792 cm-1.
3-3 Preparation of (5Z,12E)-11H-dibenzo[c,h][1,2,6]triazonine 5,6-dioxide (3)
Oxidation of the diamine by the tungstenate–peroxide (procedure 2-2-1), afford azodioxide in 21% yield after recrystallization from acetone, white crystals mp 144-145 °C. Anal. Calcd for C14H11N3O2, C, 66.41; H, 4.37; N, 16.59. Found C, 65.04; H, 3.92; N, 13.42. UV absorption in CHCl3 λmax 290 (ε = 9100) and 251 nm (ε = 7850). IR : υ (KBr), 3010, 1620, 1409, 1393, 1308, 1273, 1215, 1105, 762 cm-1. 1H NMR δH (ppm) (400 MHz, CDCl3), 7.01-7.33 m (4H); 3.97-4.09 s (2H); 1.8 s (1H). 13C NMR δC (ppm) (100 MHz, CDCl3), 144.0; 123.5; 133.3; 126.6; 123.3; 116.5;97.8; 132.3 (C=N).
ACKNOWLEDGEMENTS
We wish to express thanks to Professor B. G. Gowenlok, School of Chemistry, the University of Exeter for carrying out NMR analysis.
References
1. B. G. Gewenlock and W. Q. Luttke, Rev. Chem. Soc., 1958, 12, 321. CrossRef
2. D. A. Dictarich, I. C. Poul, and D. Incurs, J. Am. Chem. Soc., 1974, 96, 6372. CrossRef
3. L. Anderson, M. Cameron, B. G. Gewnlock, and I. J. McEwen, J. Chem. Soc., Perkin Trans. 1, 1992, 243.
4. B. G. Gowenlock, H. Speeding, T. Trotman, and B. A. Whiffin, J. Chem. Soc., Perkin Trans. 1, 1957, 3927.
5. J. C. Stowell, J. Org. Chem., 1971, 36, 3055. CrossRef
6. Z. A. N. Al-Shamkhani and A. H. Essa, Tetrahedron Lett., 2007, 48, 5547. CrossRef
7. F. Bosch and J. Kochi, J. Org. Chem., 1994, 59, 5573. CrossRef
8. A. K. Parhi and R. W. Franck, Org. Lett., 2004, 6, 3063. CrossRef
9. F. Stappers, R. Broeckx, S. Leurs, L. Van Den Bergh, J. Agten, A. Lambrechts, D. Van den Heuvel, and D. De Smaele, Org. Process Res. Dev., 2002, 6, 911. CrossRef
10. T. K. M. Shing, W. F. Wong, T. Ikeno, and T. Yamada, Org. Lett. 2007, 9, 207. CrossRef
11. D. Topic´, P. Aschwanden, R. Fässler, and E. M. Carreira, Org. Lett., 2005, 7, 5329. CrossRef
12. S. Kanemasa, Y. Oderaotoshi, J. Tanaka, and E. Wada, J. Am. Chem. Soc., 1998, 120, 12355. CrossRef
13. B. G. Gowenlock and L. Batt, J. Molecular Structure (Theochem.), 1998, 454, 103. CrossRef
14. Z. A. Nasir, M.Sc. Thesis, Basrah University, 2000.
15. M. A. Al-Naimi, A. Al-Mowali, and N. N. Mejeed, Basrah J. Science, 1998, 16, 1.
16. K. G. Orrell, V. Sik, and D. Stephenson, Mag. Reso. Chem., 1987, 25, 1007. CrossRef
17. K. G. Orrell, D. Stephenson, and T. Rault, Mag. Reso. Chem., 1989, 27, 368. CrossRef
18. R. B. Kaplan and H. Shechter, J. Am. Chem. Soc., 1961, 83, 3535. CrossRef
19. C. F. Lane, Aldrichimica Acta, 1974, 713.
20. P. L. Southwick and J. Casanova, J. Am. Chem. Soc., 1958, 80, 1168. CrossRef