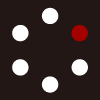
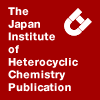
HETEROCYCLES
An International Journal for Reviews and Communications in Heterocyclic ChemistryWeb Edition ISSN: 1881-0942
Published online by The Japan Institute of Heterocyclic Chemistry
e-Journal
Full Text HTML
Received, 21st September, 2010, Accepted, 21st October, 2010, Published online, 25th October, 2010.
DOI: 10.3987/COM-10-12069
■ Synthesis of Two Estradiol-Imidazole C-Ribonucleoside Hybrid Compounds Exhibiting Inhibitory Effects against Type 1 17β-Hydroxysteroid Dehydrogenase
Shinya Harusawa,* Chihiro Kojima, Kensuke Fujii, Yuusaku Yamashita, Tomoya Tanaka, Lisa Araki, Toshinobu Yoshimura, Minoru Sakaguchi, Yoshihide Usami, and Masanori Takaoka
Osaka University of Pharmaceutical Sciences, 4-20-1 Nasahara, Takatsuki, Osaka 569-1094, Japan
Abstract
Novel estradiol-imidazole C-nucleoside hybrid compounds 4a and 4b, which have C4-linked C0- and C2-imidazole ribonucleosides as adenosine mimics and amide bond linkers, were designed and synthesized based on EM-1745, an inhibitor of type 1 17β-hydroxysteroid dehydrogenase (17β-HSD1). Compounds 4a and 4b were also tested as enzyme inhibitors.INTRODUCTION
Breast cancer is one of the most common cancers diagnosed among women and approximately 60% of breast cancers are hormone-responsive.1 Estradiol (E2), the most potent female sex hormone, stimulates the growth of mammary tumors2 and endometriosis3 by activating the estrogen receptor. One treatment approach for this type of cancer is to decrease the level of E2 by inhibiting one of the enzymes involved in its biosynthesis.4,5 Among those enzymes, type 1 17β-hydroxysteroid dehydrogenase (17β-HSD1) catalyzes the last step in the process of biosynthesis of E2, as illustrated in Figure 1.6,7 As this enzyme, utilizing the cofactor NAD(P)H, reduces the C17 ketone of estrone (E1) into E2,8 17β-HSD1 inhibitors are regarded as promising new agents for estrogen-induced diseases.9 Poirier and co-workers recently developed E2-adenosine hybrid compounds as a new type of 17β-HSD1 inhibitor (Figure 2).10,11 The compounds were designed to exhibit affinity for both substrate (E1 or E2) and cofactor [NAD(P)H] binding domains of the enzyme.11a,12 The most potent hybrid inhibitor is EM-1745 (1:IC50 = 52 nM),10 in which E2 is linked to the adenosine moiety via a 16β-oriented eight-CH2 ester spacer. Crystal structure analysis of a complex of EM-1745 and 17β-HSD1 led to the identification of a series of hydrogen bonds formed with E2 (O3/His221, O17/Ser142, and O17/Tyr155) and adenosine (NH2/Asp65 and OHs/Ser11) moieties.10,11a,12 They also reported many simplified inhibitors containing 2 to improve the bioavailability of EM-1745,13 in which aniline moieties bearing carboxylic acid function were used as adenosine mimics with 13 methylene linker (Figure 2). Although the enzyme inhibitory effects of 2 were less potent than those of EM-1745, the aniline structure of 2 showed flexibility on the adenosine moiety of EM-1745.
In our systematic studies on application towards novel bioactive compounds using imidazole C-nucleosides, we have recently reported that C4-linked (C0)- and two-carbon (C2)-elongated-imidazole ribonucleosides 3a (n = 0)14,15 and 3b (n = 2)16 are incorporated into the active sites of ribozymes to probe their role in the acid-base catalysis of the ribozymes (Figure 3).17,18 This chemogenetic approach has demonstrated the importance of particular adenine and guanine bases in the catalytic mechanism of ribozymes, showing that the imidazole-substituted ribozymes are active in both cleavage and ligation. The results suggested that the C-nucleoside 3a and its C2-elongated homologue 3b could be used as structural mimics of adenosine or guanosine having purine bases.16
In this context, we envisioned that the adenosine moiety and ester linkage in EM-1745, which were susceptible to hydrolysis, could be replaced with imidazole C-nucleoside and amide bond, respectively.19 Further, the endocyclic amine function of the imidazole had possibility to form hydrogen bonds with Asp65 of 17β-HSD1, similar to the 6-amino group of adenine in EM-1745 (Figure 2). We herein report the synthesis of new E2-imidazole C-nucleoside hybrid compounds (4a and 4b) and the preliminary evaluations for 17β-HSD1 (Figure 4).
RESULTS AND DISCUSSION
The synthesis of 5’-amino imidazole C0- and C2-nucleosides 10a and 10b, each of which constitutes the right half of the target molecules 4a and 4b, is shown in Scheme 1. Tribenzylated β-ribofuranosyl imidazole 6 was prepared through the three steps from commercially available 2,3,5-tri-O-benzyl-D-ribose 520 according to our previous procedures.21 The imidazole-N of 6 was protected by a [2-(trimethylsilyl)ethoxy]methyl (SEM) group to give the 3:1 isomeric mixture 7 (75%) at the endocyclic N functions of the imidazole. Debenzylation of 7 with Pd(OH)2/C and cyclohexene and subsequent Mitsunobu reaction [DEAD/Ph3P/phthalimide] afforded selectively desired 5’-substituted phthalimide 9a in 36% yield from 7.22 Deprotection of 9a with hydrazine hydrate afforded 5’-amino derivative 10a in 60% yield. By the way, we have recently reported a method to generate vinylimidazole 11 (E/Z = 2/1) from the starting material 5 by the six-step route in 52% yield.16 Then, for the synthesis of C2- amino homologue 10b, vinylimidazole 11 was used as the key intermediate.16 The introduction of a
Reagents and conditions: (a) NaH, SEMCl, THF, rt; (b) cyclohexene, 20% Pd(OH)2/C, EtOH, reflux;
(c) phthalimide, Ph3P, DEAD, rt; d) NH2NH2·H2O, EtOH, reflux.
SEM group at the imN position of 11 followed by debenzylation and reduction of the double bond produced N-SEM-imidazole C2-ribonucleoside 8b (73%). Conversion of 8b into phthalimide 9b (63%) and deprotection with hydrazine hydrate provided 5’-amino imidazole C2-nucleoside 10b (79%).
Carboxylic acid 16 as the left half was synthesized as shown in Scheme 2 and the starting protected allyl-E2 13 was synthesized from E1 according to the literature.23 Poirier et al. recently reported the synthesis of 16 by a cross-metathesis (CM)13 between allyl compound 13 and 7-octenal followed by oxidation of CM product.11b On the other hand, as ruthenium olefin metathesis catalysts were tolerant against even the protonic functionalities like carboxylic acids and other functional groups,24 we tried to use the commercially available 7-octenoic acid (14) on 13 for CM. When a mixture of allyl compound 13 and carboxylic acid 14 was refluxed in dichloromethane for 20 h in the presence of Hoveyda-Grubbs second generation catalyst (HG-2, 5 mol%), which was a powerful catalyst for CM,25 a coupling product 15 could be obtained in 63% yield. Catalytic hydrogenation of the double bond of 15 yielded the saturated carboxylic acid 1611b (88%). Direct use of carboxylic acid 14 made four steps of Poirier procedure unnecessary: three-step preparation of 7-octenal from 6-bromo-1-hexanol and oxidation of CM product.11b
With amines 10a and 10b and carboxylic acid 16, amide bond was formed in the presence of diethyl phosphorocyanidate (DEPC)26 and triethylamine to give amide 17a (n = 0, 67%), as shown in Scheme 3. The SEM and tert-butyldimethylsilyl (TBDMS) groups with tetra-n-butylammonium fluoride under co-existing ethylenediamine27 were removed in refluxing THF for 2 h to give unsubstituted imidazole 18a in 89% yield. Finally, cleavage of the tetrahydropyranyl (THP) group of 18a with p-toluenesulfonic acid (p-TSA) successfully afforded the target C0-compound 4a in 86% yield. Similarly, amide compound 17b (n = 2), prepared from carboxylic acid 16 and C2-amino compound 10b, was converted into the desired C2-hybrid compound 4b.
Compounds 4a and 4b were evaluated for their ability to inhibit the in vitro transformation of E1 into E2 by a human recombinant 17β-HSD1.28 Preliminary results were that 4b (IC50: 3.5 µM) showed more potent 17β-HSD1 inhibitory effect than 4a (IC50: > 10 µM). In case of the ribose-(CH2)n-imidazole moiety of 4a and 4b, insertion of the two-methylene spacer (4b, n = 2) remarkably increased the inhibitory effect compared to the case of n = 0 (4a). Although the inhibitory potency of compound 4b is even much lower (about 1 / 70) than EM-1745 (IC50: 52 nM), such kinds of hybrid compounds as E2-imidazole C-nucleoside lead to the synthesis of several analogues for a structure-activity relationship study. Further work on application of the imidazole C-nucleosides toward biofunctional molecules is under way and will be published in due course.
EXPERIMENTAL
Optical rotation measurements were recorded with a DIP-1000 digital polarimeter (JASCO). IR spectra were recorded on an IR-435 spectrometer (Shimadzu). 1H- and 13C-NMR spectra were measured with tetramethylsilane as the internal standard on Gemini-200, Mercury-300, and UNITY INOVA-500 spectrometers (Varian). Low-resolution MS and high-resolution MS were obtained on a JMS-700(2) (JEOL). Reactions with air- and moisture-sensitive compounds were carried out under the argon atmosphere. Unless otherwise noted, all extracts were dried over Na2SO4 and the solvent was removed in a rotary evaporator under reduced pressure. BW-127ZH and Chromatorex NH-DM 1020 [(NH-silica gel), Fuji Silysia] were used for column chromatography. Dehydrated THF was purchased (Wako). TLC was performed on the pre-coated TLC plates with 60F254 (silica gel, Merck).
4(5)-(2,3,5-Tri-O-benzyl-β-D-ribofuranosyl)-1-[2-(trimethylsilyl)ethoxymethyl]imidazole (7) To suspension of NaH (60%, 54 mg, 1.34 mmol) in mineral oil in THF (5 mL) was added a solution of 621(420 mg, 0.89 mmol) in THF (3 ml). The mixture was stirred at room temperature (rt) for 1.5 h to stop the evolution of hydrogen. A solution of SEMCl (237 mg, 1.34 mmol) in THF (6 mL) was added to the resulting mixture. After stirring at rt for 1 h, saturated aqueous ammonium chloride was added and the whole was evaporated to give a residue, which was subsequently distributed between CH2Cl2 and water. After the CH2Cl2 layer was separated, the aqueous layer was further extracted with CH2Cl2. The combined CH2Cl2 layer was washed with water and brine, dried over anhydrous MgSO4, filtered and evaporated. The crude product was purified by column chromatography on silica gel using hexane, followed by EtOAc/hexane (7/3) to give 7 (402 mg, 75%) as a pale yellow oil. The 3:1 isomeric mixture of 7 was assigned on the basis of the following 1H-NMR data: 1H-NMR (CDCl3) δ: 0.21 (9H, s), 1.00 -1.20 (2H, m), 3.57-3.68 (2H, m), 3.68-4.00 (2H, m), 4.23-4.60 (3H, m), 4.63-4.94 (6H, m), 5.23-5.60 (3H, m), 7.18 (0.25H, s), 7.23 (0.75H, s), 7.40-7.63 (15H, m), 7.77 (1H, s). HR-MS: m/z: 601.3103 [Calcd for C35H45N2O5Si (M+H)+: 601.3095].
4(5)-(5-Deoxy-5-phthaloylamino-β-D-ribofuranosyl)-1-[2-(trimethylsilyl)ethoxymethyl]imidazole (9a) A mixture of 7 (177 mg, 0.30 mmol), 20% Pd(OH)2/C (106 mg), and cyclohexene (0.9 mL, 8.85 mmol) in EtOH (8.5 mL) was refluxed for 28 h. After filtration through Celite, the filtrate was evaporated to give 4(5)-(β-D-ribofuranosyl)-1-[2-(trimethylsilyl)ethoxymethyl]imidazole (8a, 96 mg). 1H-NMR (CD3OD) δ: 0.00 (9H, s), 0.91 (2H, t, J = 7.2 Hz), 3.50-3.82 (4H, m), 3.93-4.03 (1H, m), 4.05-4.18 (2H, m), 4.75 (1H, d, J = 6.0 Hz), 5.43 (1.6H, s), 5.63 (0.4 H, q, J = 7.0 Hz), 7.48 (1H, s), 8.35 (1H, s). HR-MS: m/z: 331.1685 [Calcd for C14H27N2O5Si (M+H)+: 331.1687]. Phthalimide (48 mg, 0.32 mmol) and Ph3P (270 mg, 1.03 mmol) were dissolved in a solution of 8a (96 mg) in CH2Cl2 (5 mL).22,29 To this mixture, a solution of DEAD (40%, 0.47 mL, 1.03 mmol) in toluene was added slowly with stirring. After the reaction mixture was stirred for 2 h at rt, the reaction was quenched with MeOH (0.5 mL). The whole was evaporated to give a residue that was purified by column chromatography on silica gel using hexane, 50% EtOAc/hexane, EtOAc, and 10% MeOH/EtOAc to give 9a (49 mg, 36%) as an oil. 1H-NMR (CD3OD) δ: -0.20 (9H, s), 0.78-1.00 (2H, m), 3.40-4.33 (7H, m), 4.76 (1H, d, J = 4.0 Hz), 5.30 (1.2H, s), 5.40 (0.8H, s), 6.90 (0.4H, s), 7.28 (0.6H, s), 7.60-7.90 (5H, m). EI-MS: m/z: 460 (M++H). HR-MS: m/z: 460.1901 [Calcd for C22H30N3O6Si (M+H)+: 460.1904].
4(5)-(5-Amino-5-deoxy-β-D-ribofuranosyl)-1-[2-(trimethylsilyl)ethoxymethyl]imidazole (10a)
A solution of 9a (93 mg, 0.20 mmol) and NH2NH2・H2O (0.06 mL, 1.02 mmol) in EtOH (10 mL) was refluxed for 4 h and then cooled. A heaping spatula of 10% Pd/C was added to the solution and the reaction mixture was further refluxed for 20 min. After removal of the catalyst by filtration through a Celite pad, NH-silica gel (1 g) was added to the filtrate. The solvent was evaporated to give a coated silica gel, which was subsequently placed in a column (NH-silica gel, 4 g). Chromatography using 5%, 10%, and 15% MeOH in EtOAc as the eluent gave 10a (40 mg, 60%) as an oil. 1H-NMR (CD3OD) δ: 0.00 (9H, s), 0.80-1.00 (2H, m), 2.67-3.00 (2H, m), 3.53 (2H, t, J = 8.0 Hz), 3.82-4.00 (2H, m), 4.15 (0.6H, t, J = 6.0 Hz), 4.23 (0.4H, t, J = 6.0 Hz), 4.72 (0.6H, d, J = 6.0 Hz), 4.88 (0.4H, d, J = 6.0 Hz), 5.33 (1.2H, s), 5.44 (0.8H, s), 7.04 (0.4H, s), 7.25 (0.6H, s), 7.80 (1H, s). 13C-NMR (CD3OD) δ: -1.4 (-1.40), -1.4 (-1.37), 18.5, 45.0, 45.1, 67.0, 67.4, 73.7, 73.8, 75.7, 76.6, 77.1, 77.2, 80.8, 85.3, 86.3, 119.1, 128.0, 132.1, 139.5, 140.9, 142.0. HR-MS: m/z: 330.1845 [Calcd for C14H28N3O4Si (M+H)+: 330.1849].
4(5)-[(E,Z)-2-(2,3,5-Tri-O-benzyl-β-D-ribofuranos-1-yl)vinyl]-1-[2-(trimethylsilyl)ethoxymethyl]imidazole (12) Using the same procedure as that for the preparation of 7, 1116 (E/Z = 2/1, 1.30 g, 2.5 mmol) was converted into the isomeric mixture 12 (1.10 g, 70%) as an oil. 1H-NMR (CDCl3) δ: -0.05 (2H, s), -0.03 (3H, s), -0.01 (4H, s), 0.86 (0.4H, t, J = 8.1 Hz), 0.88 (0.7H, t, J = 8.1 Hz), 0.90 (0.9H, t, J = 8.1 Hz), 3.40-3.50 (2H, m), 3.52-3.65 (2H, m), 3.74 (0.2H, t, J = 5.3 Hz), 3.82 (0.5H, t, J = 5.3 Hz), 3.86 (0.3H, t, J = 5.3 Hz), 3.93-4.04 (1H, m), 4.22-4.30 (1H, m), 4.47-4.76 (7H, m), 5.14 (0.4H, s), 5.17 (0.6H, s), 5.20 (1H, s), 5.57 (0.3H, dd, J = 11.7, 8.3 Hz), 6.04 (0.2H, dd, J = 16.7, 6.7 Hz), 6.34 (0.5H, dd, J = 16.7, 6.7 Hz), 6.49 (0.3H, d, J = 11.7 Hz), 6.60 (0.5H, d, J = 16.7 Hz), 6.62 (0.2H, d, J = 16.7 Hz), 6.79 (0.3H, s), 7.12 (0.2H, s), 7.20-7.40 (15.5H, m), 7.55 and 7.58 (1H, 2s). HR-MS: m/z: 627.3252 [Calcd for C37H47N2O5Si (M+H)+: 627.3254].
4(5)-[2-(β-D-Ribofuranos-1-yl)ethyl]-1-[2-(trimethylsilyl)ethoxymethyl]imidazole (8b) Using the same procedure as that for the preparation of 8a, 12 (84 mg, 0.13 mmol) was converted into 8b (35 mg, 73%) as an oil. 1H-NMR (CD3OD) δ: -0.02 (9H, s), 0.89 and 0.90 (2H, 2t, J = 8.2 Hz), 1.75-2.04 (2H, m), 2.57-2.94 (2H, m), 3.50-3.61 (4H, m), 3.64-3.82 (4H, m), 5.32 (1.3H, s), 5.38 (0.7H, s), 6.90 (0.3H, s), 7.05 (0.7H, s), 7.89 (0.7H, s), 7.93(0.3H, s). HR-MS: m/z: 358.1919 [Calcd for C16H30N2O5Si (M+): 358.1922].
4(5)-[2-(5-Deoxy-5-phthaloylamino-β-D-ribofuranos-1-yl)ethyl]-1-[2-(trimethylsilyl)ethoxymethyl]imidazole (9b) Phthalimide (21 mg, 0.14 mmol) and Ph3P (89 mg, 0.34 mmol) were dissolved in a solution of 8b (35 mg, 0.097 mmol) in THF (4 mL). To this mixture, a toluene solution of DEAD (40%, 0.15 mL, 0.34 mmol) was added slowly with stirring. The reaction mixture was stirred for 16 h at rt and then the reaction was quenched with a small amount of water. The whole mixture was evaporated to give a residue, which was subsequently purified by column chromatography on silica gel using 10% MeOH/EtOAc to give 9b (30 mg, 63%) as a colorless oil. 1H-NMR (CD3OD) δ: -0.04 (9H, s), 0.87 (2H, t, J = 8.3 Hz), 1.70-1.99 (2H, m), 2.56-2.81 (2H, m), 3.42 (2H, t, J = 8.3 Hz), 3.72-4.13 (6H, m), 5.15 (1.3H, s), 5.17 (0.7H, s), 6.74 (0.3H, s), 6.77 (0.7H, s), 7.49 (1H, m), 7.64-7.82 (4H, m). HR-MS: m/z: 487.2126 [Calcd for C24H33N3O6Si (M+): 487.2137].
4(5)-[2-(5-Amino-5-deoxy-β-D-ribofuranos-1-yl)ethyl]-1-[2-(trimethylsilyl)ethoxymethyl]imidazole (10b) Using the same procedure as that for the preparation of 10a, 9b (29 mg, 0.06 mmol) was converted into 10b (17 mg, 79%) as a colorless oil. 1H-NMR (CD3OD) δ: -0.01 (9H, s), 0.84 (2H, t, J = 8.1 Hz), 1.72-2.06 (2H, m), 2.57-2.91 (4H, m), 3.51 and 3.53 (2H, 2t, J = 8.1 Hz), 3.68-3.82 (4H, m), 5.28 (0.7H, s), 5.34 (0.3H, s), 6.78 (0.3H, s), 6.96 (0.7H, s), 7.67 (1H, s). HR-MS: m/z: 357.2089 [Calcd for C16H31N3O4Si (M+): 357.2082].
(E,Z)-9-[3-(tert-Butyldimethylsilyloxy)-17β-(tetrahydro-2H-pyran-2-yl-oxy)-estra-1,3,5(10)-trien-16β-yl]-7-nonenoic acid (15) A mixture of protected 16β-allyl E2 1323 (114 mg, 0.22 mmol), 7-octenoic acid 14 (71 mg, 0.48 mmol), and HG-2 (10 mg, 0.015 mmol) in dry CH2Cl2 (7 mL) was refluxed for 20 h. The solvent was evaporated to give a residue, which was subsequently chromatographed with EtOAc/hexane (10 to 30%) as eluent to give 15 (87 mg, 63%) as a foam. It was dissolved by visual bubbling in saturated aqueous sodium bicarbonate. IR (film) cm-1: 1640-1810 (br, C=O). 1H-NMR (CDCl3) δ: 0.19 (6H, s), 0.80 and 0.84 (3H, 2s), 0.97 (9H, s), 1.15-2.28 (30H, m), 2.35 (2H, t, J = 11.4 Hz), 2.72-2.86 (2H, m), 3.43-3.59 (1H, m), 3.68-3.85 (1H, m), 3.85-4.02 (1H, m), 4.61-4.79 (1H, m), 5.32-5.44 (2H, m), 6.54 (1H, d, J = 3.8 Hz), 6.60 (1H, dd, J = 12.2, 3.8 Hz), 7.10 and 7.12 (1H, 2d, J = 12.2 Hz). Selected 13C-NMR (CDCl3) δ: 117.1, 119.9, 126.1, 133.2, 137.8, 153.2, 179.5 (COOH). HR-MS: m/z: 624.4205 [Calcd for C38H60O5Si (M+): 624.4207].
9-[3-(tert-Butyldimethylsilyloxy)-17β-(tetrahydro-2H-pyran-2-yl-oxy)-estra-1,3,5(10)-trien-16β-yl]nonanoic acid (16) A solution of 15 (35 mg, 0.056 mmol) in EtOH (5 mL) was hydrogenated over 10% Pd on carbon (21 mg) at 3.0 kg/cm2 for 3 h. After filtration through Celite, a small amount of silica gel was added to the filtrate. The solvent was evaporated to give a coated silica gel, which was subsequently placed in a column. Chromatography using EtOAc/hexane (5:95) as eluent gave 1611b (31 mg, 88%) as a colorless oil. It was dissolved by visual bubbling in saturated aqueous sodium bicarbonate. 1H-NMR (CDCl3) δ: 0.18 (6H, s), 0.79 and 0.83 (3H, 2s), 0.97 (9H, s), 1.03-2.30 (32H, m), 2.35 (2H, t, J = 7.0 Hz), 2.72-2.85 (2H, m), 3.44-3.56 (1H, m), 3.71 and 3.78 (1H, 2d, J = 9.6 Hz), 3.84-4.04 (1H, m), 4.58-4.77 (1H, m), 6.54 (1H, d, J = 1.9 Hz), 6.60 (1H, dd, J = 8.7, 1.9 Hz), 7.09 and 7.11 (1H, 2d, J = 8.7 Hz). HR-MS: m/z: 626.4359 [Calcd for C38H62O5Si (M+): 626.4363].
N-[1-(Imidazol-4-yl)-5-deoxy-β-D-ribofuranos-5-yl]-9-[3-hydroxy-17β-(tetrahydro-2H-pyran-2- yloxy)-estra-1,3,5(10)-trien-16β-yl]nonanamide (18a) To a solution of 16 (89 mg, 0.14 mmol) in DMF (3 mL) were added a solution of 10a (47 mg, 0.14 mmol) in DMF (2 mL), a solution of DEPC (90%, 38 mg, 0.21 mmol) in DMF (2 mL), and Et3N (58 µL, 0.42 mmol) in turn. The mixture was stirred at rt for 22 h and then diluted with EtOAc-hexane (3:1). The whole mixture was washed with H2O, saturated aq. NaHCO3, and brine, dried, filtered and evaporated to give a crude oil, which was purified by column chromatography (100% EtOAc to 5% MeOH in EtOAc) to give 17a (87 mg, 67%) as an oil. 1H-NMR (CD3OD) δ: 0.00 (9H, s), 0.17 (6H, s), 0.75-0.95 (5H, m), 0.99 (9H, s), 1.03-2.33 (35H, m), 2.70-2.88 (2H, br), 3.39-4.26 (10H, m), 4.57-4.66 (0.5H, br), 4.70 (1H, d, J = 7.2 Hz), 4.70-4.78 (0.5H, br), 5.33 (1.3H, s), 5.45 (0.7H, s), 6.50 (1H, s), 6.57 (1H, d, J = 8.0 Hz), 7.03 (0.3H, s), 7.07-7.14 (1H, m), 7.26 (0.7H, s), 7.79 (0.3H, s), 7.81 (0.7H, s). HR-MS: m/z: 938.6116 [Calcd for C52H88N3O8Si2 (M+H)+: 938.6110]. Next, a 1 M solution of tetra-n-butylammonium fluoride (0.46 mL, 0.46 mmol) in THF and ethylenediamine (0.05 mL, 0.74 mmol) were added to a solution of 17a (87 mg, 0.09 mmol) in THF (6 mL). The resulting mixture was refluxed for 3.5 h and then THF was evaporated to give a residue. The residue was subjected to chromatography [NH-silica gel, MeOH/CHCl3 (10:90 to 20:80)] to give a pale yellow oil. As 1H-NMR measurement of the oil indicated the presence of remaining tetra-n-butylammonium hydroxide, it was removed by filtration on C18 silica gel [MeOH/H2O (70:30 to 100:0)] to give 18a (57 mg, 89%) as a colorless oil. 1H-NMR (CD3OD) δ: 0.77 (1.2H, s), 0.82 (1.8H, s), 0.86-2.30 (35H, m), 2.72-2.80 (2H, br), 3.18-4.12 (8H, m), 4.57-4.62 (0.5H, br), 4.70-4.75 (0.5H, br), 4.75 (1H, d, J = 7.2 Hz), 6.46 (1H, s), 6.52 (1H, d, J = 8.0 Hz), 7.02-7.08 (1H, m), 7.10 (1H, s), 7.71 (1H, s). HR-MS: m/z: 694.4428 [Calcd for C40H60N3O7 (M+H)+: 694.4431].
N-[1-(Imidazol-4-yl)-5-deoxy-β-D-ribofuranos-5-yl]-9-[3,17β-dihydroxy-estra-1,3,5(10)-trien-16β-yl]nonanamide (4a) p-TsOH (15 mg, 0.09 mmol) was added to a solution of 18a (56 mg, 0.08 mmol) in MeOH (3.5 mL). After stirring at rt for 2 h, the reaction mixture was neutralized with saturated aq. NaHCO3 and evaporated. The residue was dissolved in EtOAc and the solution was washed with saturated aq.NaHCO3 and brine, dried, filtered, and evaporated. The residual oil was purified on N-H silica gel using MeOH-CHCl3 (5:95 to 15:85, v/v) to give 4a (42 mg, 86%) as a colorless oil. Rf = 0.33 (30% MeOH in CHCl3). [α]D = + 26.2o (c = 1.85, MeOH). 1H-NMR (CD3OD) δ: 0.76 (3H, s), 0.93-2.30 (29H, m), 2.71-2.84 (2H, m), 3.20-3.70 (3H, m), 3.93-3.99 (2H, m), 4.06-4.11 (1H, m), 4.73 (1H, d, J = 6.1 Hz), 6.47 (1H, d, J = 2.7 Hz), 6.53 (1H, dd, J = 8.5, 2.7 Hz), 7.06 (1H, d, J = 8.5 Hz), 7.09 (1H, s), 7.68 (1H, s). 13C-NMR (CD3OD) δ: 13.3, 27.1, 27.6, 28.8, 29.9, 30.4, 30.5, 30.7, 30.8, 31.0, 33.0, 33.7, 37.1, 39.1, 40.0, 41.7, 42.5, 45.2, 45.5, 49.9, 50.0, 62.3, 73.5, 76.8, 80.2, 83.4, 83.8, 113.7, 116.1, 127.2, 132.7, 137.0, 138.8, 155.9, 176.7. HR-MS: m/z: 610.3860 [Calcd for C35H52N3O6 (M+H)+: 610.3856].
N-{1-[2-(Imidazol-4-yl)ethyl]-5-deoxy-β-D-ribofuranos-5-yl}-9-[3-hydroxy-17β-(tetrahydro-2H-pyran-2-yloxy)-estra-1,3,5(10)-trien-16β-yl]nonanamide (18b) A mixture of 16 (67 mg, 0.11 mmol), 10b (41 mg, 0.12 mmol), DEPC (29 mg, 0.16 mmol), and Et3N (0.05 mL, 0.32 mmol) in DMF (10 mL) was stirred at rt for 13 h to give 17b (64 mg, 62%) as a colorless oil using the same procedure as that for the preparation of 17a. 1H-NMR (CD3OD) δ: -0.08 (9H, s), 0.17 (6H, s), 0.78 and 0.82 (3H, 2s), 0.84-0.93 (2H, m), 0.96 (9H, s), 1.02-2.06 (33H, m), 2.08-2.30 (2H, m), 2.18 (2H, t, J = 6.9 Hz), 2.62-2.88 (4H, m), 3.36-3.58 (5H, m), 3.66-3.88 (5H, m), 3.89-4.15 (1H, m), 4.57-4.75 (1H, m), 5.18 (0.7H, s), 5.21 (0.3H, s), 6.50 (1H, d, J = 2.3 Hz), 6.55 (1H, dd, J = 9.2, 2.3 Hz), 6.78 (0.3H, s), 6.94 (0.7H, s), 7.08 and 7.10 (1H, 2d, J = 9.2 Hz), 7.52 (1H, s). HR-MS: m/z: 966.6422 [Calcd for C54H92N3O8Si2 (M+H)+: 966.6418]. A mixture of 17b (18 mg, 0.02 mmol), 1 M THF solution of tetra-n-butylammonium fluoride (0.09 mL, 0.09 mmol) and ethylenediamine (0.01 mL, 0.15 mmol) in THF (1.5 mL) was refluxed for 2 h to give 18b (9 mg, 69%) as a colorless oil using the same procedure as that for the preparation of 18a. 1H-NMR (CD3OD) δ: 0.78 and 0.82 (3H, 2s), 1.08-2.06 (37H, m), 2.06-2.83 (2H, m), 2.20 (2H, t, J = 8.2 Hz), 2.60-2.83 (4H, m), 3.26-3.56 (2H, m), 3.64-3.82 (5H, m), 3.84-4.03 (1H, m), 4.56-4.73 (1H, m), 5.32-5.43 (2H, m), 6.46 (1H, d, J = 3.0 Hz), 6.52 (1H, dd, J = 9.0, 3.0 Hz), 6.77 (1H, s), 7.04 and 7.05 (1H, 2d, J = 9.0 Hz), 7.53 (1H, s). HR-MS: m/z: 722.4739 [Calcd for C42H64N3O7 (M+H)+: 722.4740].
N-{1-[2-(Imidazol-4-yl)ethyl]-5-deoxy-β-D-ribofuranos-5-yl}-9-[3,17β-dihydroxy-estra-1,3,5(10)-trien-16β-yl]nonanamide (4b) A mixture of 18b (20 mg, 0.03 mmol) and p-TsOH (5 mg, 0.03 mmol) in MeOH (1 mL) was stirred at rt for 4 h to give 4b (12 mg, 70%) as a colorless oil using the same procedure as that for the preparation of 4a. Rf = 0.32 (20% MeOH in CHCl3). [α]D = + 25.5o (c = 1.65, MeOH). 1H-NMR (CD3OD) δ: 0.76 (3H, s), 1.22-2.34 (32H, m), 2.20 (2H, t, J = 7.5 Hz), 2.60-2.87 (4H, m), 3.64-3.83 (5H, m), 6.46 (1H, d, J = 3.2 Hz), 6.52 (1H, dd, J = 7.9, 3.2 Hz), 6.78 (1H, s), 7.06 (1H, d, J = 7.9 Hz), 7.56 (1H, s). 13C-NMR (CD3OD) δ: 13.5, 24.1, 27.2, 27.7, 28.9, 30.0, 30.5, 30.6, 30.8, 30.9, 31.2, 33.1, 33.7, 34.9, 37.1, 39.1, 40.1, 41.7, 42.8, 45.2, 45.5, 50.0, 73.8, 76.0, 83.1, 83.3, 113.2, 115.5, 126.6, 132.1, 135.1, 138.1, 155.1, 175.6. HR-MS: m/z: 638.4165 [Calcd for C37H56N3O6 (M+H)+: 638.4166].
Biological Assay
Human 17β-HSD1 was overexpressed in the BL21-AI strain of Escherichia coli containing the pET-41 Ek/LIC-17β-HSD1 vector, basically according to the method of Chang et al.28 The human recombinant 17β-HSD1 was purified by using a Glutathione Sepharose 4B column (GE Healthcare) and a Mono Q 5/50 GL column (GE Healthcare). The purified enzyme was incubated with substrate E1 and cofactor NADH in the presence or absence of inhibitors at pH 7.4. The residual E1 and newly produced E2 in the incubation mixture were extracted with hexane. The organic phase was evaporated, the residue containing E1 and E2 was dissolved in acetonitrile solution and then measured by reverse-phase high-performance liquid chromatography with an amperometric detector (HTEC-500, EICOM).
ACKNOWLEDGEMENTS
We are grateful to Dr. K. Minoura and Ms. M. Fujitake of Osaka University of Pharmaceutical Sciences for NMR and MS measurements, respectively. This work was supported in part by a Grant-in-Aid for Scientific Research [No. 21590130 (S. H.)] from JSPS and a Grant-in-Aid for “High Technology Research Center from MEXT (Ministry of Education, Sciences, Sports and Culture), 2009-2013, Japan.
References
1. A. J. Theobald, Int. J. Clin. Pract., 2000, 54, 665.
2. R. C. Travis and T. J. Key, Breast Cancer Res., 2003, 5, 239. CrossRef
3. G. S. Dizerega, D. L. Barber, and G. D. Hodgen, Fertil. Steril., 1980, 33, 649.
4. F. Labrie, V. Luu-The, S. X. Lin, J. Simard, C. Labrie, M. Elalfy, G. Pelletier, and A. Bélanger, J. Mol. Endocrinol., 2000, 25, 1. CrossRef
5. H. J. Smith, P. J. Nicholls, C. Simons, and R. Le Latin, Exp. Opin, Ther. Patents, 2001, 11, 789. CrossRef
6. V. Luu-The, Y. Zhang, D. Poirier, and F. Labrie, J. Steroid Biochem. Mol. Biol., 1995, 55, 581. CrossRef
7. V. Luu-The, J. Steroid Biochem. Mol. Biol., 2001, 76, 143. CrossRef
8. R. Breton, D. Housset, C. Mazza, and J. C. Fontecilla-Camps, Structure, 1996, 4, 905. CrossRef
9. J. M. Day, H. J. Tutill, A. Purohit, and M. J. Reed, Endocr. Relat. Cancer., 2008, 15, 665 and references therein. CrossRef
10. D. Poirier, R. P. Boivin, M. R. Tremblay, M. Bérubé, W. Qiu, and S.-X. Lin, J. Med. Chem., 2005, 48, 8134. CrossRef
11. (a) D. Fournier, D. Poirier, M. Mazumdar, and S. -X. Lin, Eur. J. Med. Chem., 2008, 43, 2298; CrossRef (b) M. Bérubé and D. Poirier, J. Enzyme Inhib. Med. Chem., 2009, 24, 832. CrossRef
12. W. Qiu, R. L. Campbell, A. Gangloff, P. Dupuis, R. P. Boivin, M. R. Tremblay, D. Poirier, and S.-X. Lin, FASEB., 2002, 16, 1829.
13. (a) M. Bérubé and D. Poirier, Org. Lett., 2004, 6, 3127; CrossRef (b) M. Bérubé and D. Poirier, Can. J. Chem, 2009, 87, 1180. CrossRef
14. (a) L. Araki, S. Harusawa, M. Yamaguchi, S. Yonezawa, N. Taniguchi, D. M. J. Lilley, Z. Zhao, and T. Kurihara, Tetrahedron Lett., 2004, 45, 2657; CrossRef (b) L.Araki, S. Harusawa, M. Yamaguchi, S. Yonezawa, N. Taniguchi, D. M. J. Lilley, Z. Zhao, and T. Kurihara, Tetrahedron, 2005, 61, 11976. CrossRef
15. L. Araki, Z. Zhao, D. M. J. Lilley, and S. Harusawa, Heterocycles, 2010, 81, 1861. CrossRef
16. L. Araki, K. Morita, M. Yamaguchi, Z. Zhao, T. J. Wilson, D. M. J. Lilley, and S. Harusawa, J. Org. Chem., 2009, 74, 2350. CrossRef
17. Z. Zhao, A. Mcleod, S. Harusawa, L. Araki, M. Yamaguchi, T. Kurihara, and D. M. J. Lilley, J. Am. Chem. Soc., 2005, 127, 5026. CrossRef
18. T. J. Wilson, J. Ouellet, Z. Zhao, S. Harusawa, L. Araki, T. Kurihara, and D. M. J. Lilley, RNA, 2006, 12, 980. CrossRef
19. D. E. Levy and C. Tang, ‘Tetrahedron Organic Chemistry Series: The Chemistry of C-Glycosides,’ Vol. 13, Chap. 1, ed. by J. E. Baldwin and P. D. Magnus, Pergamon, New York, 1995, pp. 4-27.
20. 2,3,5-Tri-O-benzyl-D-ribofuranose 5 was purchased from Carbosynth Ltd.
21. S. Harusawa, M. Murai, H. Moriyama, T. Imazu, H. Ohishi, R. Yoneda, and T. Kurihara, J. Org. Chem.,1996, 61, 4405. CrossRef
22. S. Harusawa, H. Moriyama, Y. Murai, T. Imazu, H. Ohishi, R. Yoneda, T. Kurihara, H. Hata, and Y. Sakamoto, Chem Pharm. Bull.,1997, 45, 53. CrossRef
23. M. R. Tremblay and D. Poirier, J. Comb. Chem., 2000, 2, 48. CrossRef
24. (a) S. J. Connon and S. Blechert, Angew. Chem. Int. Ed., 2003, 42, 1900; CrossRef (b) A. Fürstner, Angew. Chem. Int. Ed., 2000, 39, 3012. CrossRef
25. S. Randl, S. Gessler, H. Wakamatsu, and S. Blechert, Synlett, 2001, 430. CrossRef
26. T. Shioiri, Y. Yokoyama, Y. Kasai, and S. Yamada, Tetrahedron, 1976, 32, 2211. CrossRef
27. J. M. Muchowski and D. R. Solas, J. Org. Chem.,1984, 49, 203. CrossRef
28. Y. H. Chang, Y. L. Wang, J. Y. Lin, L. Y. Chuang, and C. C. Hwang, Mol. Biotechnol., 2010, 44, 133. CrossRef
29. Since triol 8a was insoluble in THF, dichloromethane was used as a solvent for the reaction.