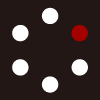
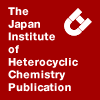
HETEROCYCLES
An International Journal for Reviews and Communications in Heterocyclic ChemistryWeb Edition ISSN: 1881-0942
Published online by The Japan Institute of Heterocyclic Chemistry
e-Journal
Full Text HTML
Received, 28th September, 2010, Accepted, 11th November, 2010, Published online, 12th November, 2010.
DOI: 10.3987/COM-10-12072
■ Facile Synthesis of 3-(Succinimid-3-yl)-2-oxo-2,3-dihydroimidazo[1,2-a]pyridine Derivatives by Sequential Intra- and Intermolecular Michael Reactions between 2-Aminopyridines and Maleimides
Tetsuro Shimo,* Tomoko Itoh, Yasutaka Araki, Tetsuo Iwanaga, Teruo Shinmyozu, and Kenichi Somekawa
Department of Applied Chemistry and Chemical Engineering, Faculty of Engineering, Kagoshima University, Korimoto, Kagoshima 890, Japan
Abstract
3-(Succinimid-3-yl)-2-oxo-2,3-dihydroimidazo[1,2-a]pyridine derivatives (3 and 4) were prepared through a one-pot reaction from 2-aminopyridines (1) by acylation with maleimides (2) and followed by an intramolecular Michael addition, and a subsequent second Michael addition with another molecule of 2. The diastereomeric configurations of the products were confirmed by X-ray crystal analyses. The reaction mechanism of the accumulated three types of additions between 1 and two equimolar amounts of 2 was calculated using MOPAC-PM6 molecular simulations and the competing addition reactions as hard and soft reactions were explained by HSAB theory.In recent years, significant attention has been paid to the synthesis or further transformations of fused [5,6] herero-ring systems. In particular, noticeable interest has focused on imidazo[1,2-a]pyridines, fused bicyclic [5,6] heterocycles with a one-ring-junction nitrogen atom and one extra nitrogen atom in the five-membered ring, because these compounds show high binding affinity to multiple receptors. The high binding affinity towards receptors indicates that these heterocycles may represent useful therapeutic compounds, such as antifungal, antibacterial and local anesthetic agents.1 There are several methods that describe the synthesis of 2-substituted or 3-substituted imidazo[1,2-a]pyridines. These methods depend primarily on condensation reactions of 2-aminopyridines with α-halocarbonyl compounds,2 glyoxal trimer dihydrate,3 or aldehydes and isonitriles4 to form the five-membered cyclic systems. It has been reported that several 2-oxo-2,3-dihydroimidazo[1,2-a]pyridines and, especially, 3,3-dibenzoyl-2-oxo-2,3-dihydro-imidazo[1,2-a]pyridine (ZSET 845) improve cerebral function, and therefore may represent therapeutic treatments for cognitive and memory disorders including Altzheimer’s desease.5 Since these types of compounds are important, we describe herein a facile synthesis and structural analysis of particular [3-(succinimid-3-yl)-2-oxo-2,3- dihydroimidazo[1,2-a]pyridin-3yl]acetamides through a one-pot reaction of 2-aminopyridines with maleimides. The reaction mechanism of accumulated three types of additions between 2-aminopyridines and two equimolar amounts of maleimides were found to be very interesting, because the additions are competing for acylation and hard and soft Michael addition reactions. Such types of selective reactions are present in many biochemical reactions.6 We have previously reported many thermal7 and photochemical8 bimolecular addition and isomerization reactions. The reaction mechanisms were analyzed by a molecular orbital method containing frontier molecular orbital (FMO) theory8 and the transition state (TS) analysis. 9 The reaction mechanism of the accumulated processes for products 3 and 4 may be analyzed using the recent MOPAC2009-PM6 level.10 The PM6 accuracy by average unsigned error (AUE) of heat of formation (HOF) for 1493 organic molecules was found to be higher than that of B3LYP/6-31G* levels.
A solution of 4-methyl-2-amiopyridine (1b) (1.6 mmol) and maleimide (2a) (3.2 mmol) in acetonitrile (10ml) was refluxed for 8 h under nitrogen atmosphere. Upon cooling to room temperature, the product 3ba (40% yield) crystallized out of solution. The filtrate was left standing overnight to give 4ba in 25% yield (Scheme 1 and entry 2 in Table 1). Product 3ba was estimated as a diastereomer of 4ba from X-ray crystallographic analysis of 4ba. The 1H NMR spectral data between 3ba and 4ba were similar to each other.11 The results of the similar reactions of 1a-c with 2a,b are summarized in Table 1. The yields of products 4ca and 4cb were calculated by 1H NMR analyses (entry 6,7) because it was difficult to isolate these compounds from the recrystallization. Products 3ca, which was obtained from the reaction of 5-methyl-2-aminopyridine (1c) with 2a (entry 6), and 4ba were recrystallized from water to give single crystals, and the structures of 3ca and 4ba (Figure 1)12 were established by X-ray crystallographyic analyses as 3S,10R- and 3R,10R-[3-(succinimid-3-yl)-2-oxo-2,3-dihydroimidazo- [1,2-a]pyridin-3-yl]- acetamides, respectively. The assignments of the same structures (3 and 4) were based on their 1H- NMR , IR and MS spectra that were analogous to those of 3ca and 4ba.11
The reaction of 1 with two equimolar amount of 2 gave 3 and 4 via two types of intermolecular reactions at the carbonyl group and the C-C double bond of 2. The reaction mechanism was estimated to proceed via acylation, two types of Michael additions and a proton transfer, as estimated from MO calculations using the accurate MOPAC PM6 level (Scheme 2).10 The values of the heat of formation
(HOF) (1-4, I-VI) are shown in Scheme 2, and those of the ionization potential (IP), frontier orbital coefficients (HOMO, LUMO) and atomic charge (AC) of 1, 2 and V are listed in Table 2. The changes of HOF values (kcal/mol) (- 20.93 → - 21.7 → - 27.97 in the reaction (1), - 30.60 → - 35.49 → - 46.44 in the reaction (2) and –98.07 → - 95.90 → - 120.61 in the reaction (3) in Scheme 2) are reasonable and suggest that these reactions proceed smoothly. The reactions (1) and (3) are also competing reactions for maleimide (2). They are similar to following soft and hard reactions ((4) and (5) in Scheme 3)13 of HSAB theory. The reaction of each step between 1 and 2 was reasonably explained as follows. Since the AC value at the 7-position (NH2) of 1 was more negative than at the 1-position of 1 (also the HOMO coefficient at the 7-position was larger than at the 1-position) and the AC value at the 3-position (C=O) of 2 was more positive than at the 1-position (Table 2), the reaction of 1 at the 7-position with 2 at the 3-position has been proceeded by the hard reaction to give I, according to the hard and soft acids and bases (HSAB) theory. Intermediate I afforded II via a proton transfer and followed by the ring opening reaction of the γ-lactam to give III. III underwent intramolecular.
Michael addition to give V having lower HOF than that of IV. Since the IP value of V was smaller than that of 1 and the HOMO coefficient at the 3-position of V was the largest value (Table 2), V reacted with another molecule of 2 at the olefinic part by the soft reaction to afford 3 or 4 via VI. The activation energy (ΔEa) of the reaction between V and 2 to give VI was estimated to 12.23 kcal/mol (vibration analysis: - 486.7 cm-1) using PM6 methods. It was found that intermediate IV, whose skeleton is known,14 was a very active species because trials to obtain IV derived from the reaction of 1b with 2a were unsuccessful in giving 3ba and 4ba, even under mild conditions (solution reaction at room temperature) or solid-state conditions (reaction at 80 ℃ without solvent) (Table 1, entry 3, 4). It was assumed that the structure of the intermediate IV, obtained by the reaction between 1 and maleic anhydride, was stable owing to the formation of an inner salt as shown in Scheme 4.14
The reaction (3) is competing with the reaction (1) and the formation of 3 and 4 as diastereomers suggests that the soft reaction (3) by the intermediate V is faster than the reaction (1).
References
1. (a) G. Trapani, M. Franco, A. Latrofa, L. Ricciardt, A. Carotti, M. Serra, E. Sanna, G. Biggto, and C. Liso, J. Med. Chem., 1999, 42, 3934; CrossRef (b)Y. Rival, G. Grassy, and G. Michael, Chem. Pharm. Bull., 1992, 40, 1170; (c) M. A. Lyon and T. M. Kercher, Org. Lett., 2004, 26, 4989; CrossRef (d) J. C. Aramillo, J. C. Carretero, J. E. de Diego, M. del Prado, C. Hamdouchi, J. L. Roldan, and C. Sanchez-Martinez, Tetrahedron Lett., 2002, 43, 9051; CrossRef (e) J. J. Kaminski, B. Wallmark, C. Briving, and B.-M. Andersson, J. Med. Chem., 1991, 34, 533; CrossRef (f) G. J. Georges, D. P. Vercauteren, G. H. Evrad, F. V. Durant, P. G. George, and A. E. Wick, Eur. J. Med. Chem., 1993, 28, 323. CrossRef
2. (a) H. Tomoda, T. Hirano, S. Saito, T. Mutai, and K. Araki, Bull. Chem. Soc. Jpn., 1999, 72, 1327; CrossRef (b) T. Ikemoto and M. Wakimasu, Heterocycles, 2001, 55, 99; CrossRef (c) R. Rydzkowski, D. Blondeau, and H. Sliua, Tetrahedron Lett., 1986, 26, 2571. CrossRef
3. M. P. Groziak, S. R. Wilson, G. L. Clauson, and N. Leonard, J. J. Am. Chem. Soc., 1986, 108, 8002. CrossRef
4. K. Groebke, L. Weber, and F. Mehlin, Synlett, 1998, 661. CrossRef
5. (a) A. Lockhart, L. Ye, D. B. Judd, A. T. Merritt, P. N. Lowe, J. L. Morgenstern, G. Z. Hong, A. D. Gee, and J. Brown, J. Biol. Chem., 2005, 280, 7677; CrossRef (b) Y. Yamaguchi, T. Matsuno, and S. Kawashima, Brain Res., 2002, 945, 259; CrossRef (c) Y. Yamaguchi, M. Higashi, T. Matsuno, and S. Kawashima, Jpn. J. Pharmacol., 2001, 87, 240. CrossRef
6. P. Y. Bruice (translated by Y. Ohfune, T. Katsuki, K. Saigo, and K. Tomioka), Organic Chemistry, Fouth ed., Kagaku-Dojin (2004) p. 869.
7. S. Kiri, Y. Odo, H. I. Omar, T. Shimo, and K. Somekawa, Bull. Chem. Soc. Jpn., 2004, 77, 1499. CrossRef
8. H. Miyauchi, C. Ikematsu, T. Shimazaki, S. Kato, T. Shinmyozu, T. Shimo, and K. Somekawa, Tetrahedron, 2008, 64, 4108. CrossRef
9. K. Somekawa, Y. Odo, T. Ooto, H. Hashimoto, J. Miyaji, and T. Shimo, J. Comput. Chem. Jpn., 2010, 9, 79. CrossRef
10. MOPAC2009, J. J. P. Stewart, Stewart Computational Chemistry, Colorado Springs, CO, USA, http://openmopac.net (2008).
11. All the new compounds gave the correct analytical and MS data. Selected spectral data are given below. 3aa: mp 243-246 ℃; 1H NMR (DMSO-d6) δ 1.73 (1H, dd, J=18.0, 4.0 Hz), 2.53 (1H, dd, J=18.0, 9.2 Hz), 3.18, 3.30 (each 1H, d, J=17.3 Hz), 3.74 (1H, dd, J=9.2, 4.0 Hz), 6.83, 7.43, 11.33 (each 1H, s), 6.85 (1H, dd, J=7.6, 6.4 Hz), 7.07 (1H, d, J=9.2 Hz), 7.79 (1H, dd, J=9.2, 7.6 Hz), 8.39 (1H, d, J=6.4 Hz). IR (KBr) 1770, 1740, 1673 cm-1. 4aa: mp 206-207 ℃; 1H NMR (DMSO-d6) δ 2.75 (1H, dd, J=18.0, 9.2 Hz), 2.81, 3.10 (each 1H, d, J=16.0 Hz), 3.02 (1H, dd, J=18.0, 4.4 Hz), 3.63 (1H, dd, J=9.2, 4.4 Hz), 6.86, (1H, dd, J=7.6, 6.8 Hz), 6.96, 7.45, 11.20 (each 1H, s), 7.03 (1H, d, J=8.8Hz), 7.78 (1H, dd, J=8.8, 7.6 Hz), 8.31(1H, d, J=6.8 Hz). IR (KBr) 1780, 1720, 1683 cm-1. 3ba: mp 232-236 ℃; 1H NMR (DMSO-d6) δ 1.71 (1H, dd, J=18.0, 4.0 Hz), 2.33 (3H, s), 2.40 (1H, dd, J=18.0, 9.2 Hz), 3.15, 3.22 (each 1H, d, J=16.4 Hz), 3.73 (1H, dd, J=9.2, 4.0 Hz), 6.72 (1H, d, J=6.8 Hz), 6.82, 7.41, 11.30 (each 1H, s), 6.91 (1H, s), 8.24 (1H, d, J=6.8 Hz). IR (KBr) 1760, 1735, 1665 cm-1. 4ba: mp 207- 210 ℃; 1H NMR (DMSO-d6) δ 2.33 (3H, s), 2.74 (1H, dd, J=18.2, 9.2 Hz), 2.76, 3.08 (each 1H, d, J=15.6 Hz), 2.97 (1H, dd, J=18.2, 4.4 Hz), 3.61 (1H, dd, J=9.2, 4.4 Hz), 6.73, (1H, d, J=6.8 Hz), 6.85, 7.42, 11.19 (each 1H, s), 6.93 (1H, s), 8.14 (1H, d, J=7.2 Hz). IR (KBr) 1775, 1710, 1650 cm-1. 3bb: mp 181-184 ℃; 1H NMR (DMSO-d6) δ 1.85 (1H, dd, J=18.0, 4.0 Hz), 2.37 (3H, s), 2.83 (1H, dd, J=18.0, 6.0 Hz), 3.50, 3.61 (each 1H, d, J=17.2 Hz), 3.96 (1H, dd, J=6.0, 4.0 Hz), 6.83 (1H, d, J=6.0 Hz), 7.00, 7.1-7.5 (10H, m), 7.01 (1H, s), 8.46 (1H, d, J=6.0 Hz), 10.16 (1H, s). IR (KBr) 1780, 1710, cm-1. 4bb: mp 162-165 ℃; 1H NMR (DMSO-d6) δ 2.33 (3H, s), 2.82 (1H, dd, J=15.6, 6.0 Hz), 2.87, 3.05 (each 1H, d, J=15.6Hz), 3.02 (each 1H, dd, J=15.6, 4.0 Hz), 3.82 (1H, dd, J=6.0, 4.0 Hz), 6.65 (1H, d, J=6.0 Hz), 6.80 (1H, s), 7.00, 7.1-7.5 (10H, m), 8.32 (1H, d, J=6.0 Hz), 10.10 (1H, s). IR (KBr) 1780, 1710 cm-1. 3ca: mp 240-243 ℃; 1H NMR (DMSO-d6) δ 1.70 (1H, dd, J=18.0, 4.0 Hz), 2.17 (3H, s), 2.50 (1H, dd, J=18.0, 9.2 Hz), 3.14, 3.30 (each 1H, d, J=16.0 Hz), 3.69 (1H, dd, J=9.2, 4.0 Hz), 6.81, 7.42, 11.31 (each 1H, s), 7.02 (1H, d, J=9.2 Hz), 7.68 (1H, d, 9.2 Hz), 8.22 (1H, s). IR (KBr) 1780, 1720, 1680 cm-1. 4ca (not isolated): 1H NMR (DMSO-d6) δ 2.17 (3H, s), 2.65 (1H, dd, J=18.0, 9.2 Hz), 2.80, 3.07 (each 1H, d, J=16.4 Hz), 2.96 (1H, dd, J=18.0, 4.0 Hz), 3.59 (1H, dd, J=9.2, 4.9 Hz), 6.91, 7.80, 11.20 (each 1H, s), 6.98 (1H, d, J=9.2 Hz), 7.67 (1H, d, J=9.2 Hz), 8.15 (1H, s). 3cb: mp 252- 255 ℃; 1H NMR (DMSO-d6) δ 1.85 (1H, dd, J=18.4, 6.0 Hz), 2.21 (3H, s), 2.84 (1H, dd, J=18.4, 9.2 Hz), 3.48, 3.66 (each 1H, d, J=16.8Hz), 3.91 (1H, dd, J=9.2, 4.4 Hz), 7.1 (1H, d, J=9.2 Hz), 7.0-7.5 (10H, m), 7.76 (1H, d, J=9.2 Hz), 8.42 (1H, s), 10.15 (1H, s). IR (KBr) 1780, 1710 cm-1. 4cb (not isolated): 1H NMR (DMSO-d6) δ 2.14 (3H, s), 2.95 (1H, dd, J=18.0, 5.2 Hz), 3.05 (1H, dd, J=18.0, 9.2 Hz), 3.20, 3.46 (each 1H, d, J=16.8 Hz), 3.80 (1H, dd, J=9.6, 4.0 Hz), 7.0-7.5 (11H, m), 7.73 (1H, d, J=9.0 Hz), 8.32 (1H, s).
12. X-Ray crystal data for 4ba (C14H14N4O4); T=23.0 ℃, Mo-Kα (Rigaku RAXSIS-RAPID imaging plate diffractometer, λ=0.71069 Å), crystal dimensions 0.12 x 0.15 x 0.57 mm3 (a colorless block crystal), a=7.3922 (4), b=8.8511 (5), c=12.6067 (9) Å, α=94.739 (5), β=93.452 (4), γ=114.591 (2) ゜triclinic, space group P-1 (#2), Z=2, µMoKα=1.02 cm-1, Mr=302.29, V=743.37 (5) Å3, anode power 50 KV x 32 mA, ρcalc=1.350 g/cm3, 2θmax=54.9゜, F (000)=316.00. 6128 reflections measured, 2846 observed (I > 2σ (I)), number of parameters 217. The structure was solved by direct methods and was refined on SIR 97.15 Data were corrected for Lorentz polarizations. The data/parameter ratio was 13.12. R=0.051, Rw=0.178, GOF=1.46, max/min residual density +0.65/-0.47 eÅ-3. 3ca (C14H14N4O4); T= - 150 ℃, crystal dimensions 0.57 x 0.57 x 0.13 mm3 (a colorless platelet crystal), a=21.212 (2), b=5.9784 (4), c=23.791 (1) Å, β=104.188 (2) ゜, monoclinic, space group C2/c (#15), Z=6, µMoKα=0.77 cm-1, Mr=302.29, V=2925.1 (3) Å3, ρcalc=1.030 g/cm3, F (000)=948.00. 13090 reflections measured, 2753 observed (I > 2σ (I)), number of parameters 208. The structure was solved by direct method and was refined on SIR 97. The data/parameter ratio was 13.24. R=0.077, Rw=0.259, GOF=1.87, max/min residual density +0.70/-0.8 eÅ-3. All calculations were performed using the teXsan crystallographic software package of Molecular Structure Corporation.
13. I. Fleming (edited by K. Fukui and translated by Y. Takeuchi, and S. Tomoda), Frontier Orbitals and Organic Chemical Reactions, Sixth ed., Kodansha (1986) p. 83.
14. A. V. Dolzhenko, N. V. Kolotova, V. O. Kozminykh, W.-K. Chui, P. W. S. Heng, and V. N. Khrustalev, Heterocycles, 2004, 63, 55. CrossRef
15. A. Altomare, M. C. Burla, M. Camalli, G. L. Cascarano, C. Giacovazzo, A. Guagliardi, A. G. G. Moliterni, G. Polidori, and R. Spagna, J. Appl. Cryst., 1999, 32, 115. CrossRef