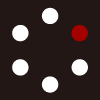
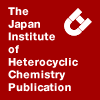
HETEROCYCLES
An International Journal for Reviews and Communications in Heterocyclic ChemistryWeb Edition ISSN: 1881-0942
Published online by The Japan Institute of Heterocyclic Chemistry
e-Journal
Full Text HTML
Received, 14th October, 2010, Accepted, 14th December, 2010, Published online, 27th December, 2010.
DOI: 10.3987/REV-10-685
■ Synthesis, Properties and Structures of Phosphorus-Nitrogen Heterocycles
Vasile Simulescu,* Eleonora Crasmareanu, and Gheorghe Ilia
Department of Organic Chemistry, Institute of Chemistry, Timisoara of the Romanian Academy, 24 Mihai Viteazu Bvd., 300223-Timisoara, Romania
Abstract
The synthesis, properties and structures of heterocyclic molecules containing phosphorous and nitrogen are described in this review. A systematic synthesis of a ring compound needs one or more bifunctional starting molecules that react in a special way to form new bonds and to obtain the ring. Oligomers or polymers can be obtained besides other products resulting from leaving groups necessary to form the new bonds. Hydrazine for example is one of the simplest bifunctional compounds that must be able to introduce two vicinal nitrogen atoms into a cycle, as it will be shown further.CONTENT
INTRODUCTION
1. Nonalternating inorganic heterocycles containing hydrazine as building block. Hydrazine derivatives of phosphoric and thiophosphoric acid
2. Monomethylhydrazine derivatives of phosphorus (III) and (V) compounds
3. Spirocycles containing two or more phosphorus atoms combination of hydrazine rings and cyclophosphazenes
4. Heterocycles containing phosphorus from N,N-bis[(–)-norpseudoephedrine]
5. Synthesis of novel chiral 1-phosphono-1,3-butadiene for asymmetric hetero Diels-Alder cycloadditions
INTRODUCTION
The role of aromatic heterocycles containing phosphorus and nitrogen has been recognized and established in the regulation of enzymatic activity, function, folding and stability of biological systems.1-6 In the Figure 1 are presented few structures of several aromatic heterocycles containing phosphorus.1
In addition, these heterocyclic compounds showed a significant role in the design of organic nanotubes, biological receptor models and ionophores was recognized as well.7,8 A lot of studies were performed on aromatic systems (benzene, tryptophan, phenylalanine, tyrosine etc).9-12
These heterocyclic systems (1-14) considered in the Figure 1, provide also a possibility to realize a large number of complexes with metals. The phospholes and phosphinines can form strong complexes with metal ions (Li+, Mg2+, Ca2+, Na+, K+).1 There are about a dozen crystal structures which have Li+ and Na+ bound to phosphorus systems.13,14
In this review further it will be shown several methods of synthesis and several properties of few heterocycles containing phosphorus and nitrogen.
1. Nonalternating inorganic heterocycles containing hydrazine as building block. Hydrazine derivatives of phosphoric and thiophosphoric acid
A lot of different possibilities to obtain heterocycles containing phosphorus were developed during the last decades. Here several examples will be described.
For example, using the phosphorus halogenated ester 15, the heterocycle 16 can be obtained (Figure 2) in around 13% yield, by a chemical process which occurs in anhydrous conditions using pyridine as base and tetrahydrofuran as solvent. The heterocycle 16 was purified after several recrystallisations.15
The 31P NMR and 1H NMR spectra and determinations of molecular weight were in good agreement with the assumed structure for the heterocycle 16. The melting point of it was around 259-260 °C. The reaction of heterocycle 16 with KOH in ethanol, under reflux, produces the carbon-free dipotassium-salt 17 (Figure 3).
This salt (17) can not be oxidized to the corresponding azo-compound 18 (Figure 3).16 No color change was observed, as in the case of noncyclic hydrazidophosphonates.17
On the other hand, the decomposition of it occurs under nitrogen.16 Resynthesizing the phenylester of the corresponding dithio-ring compound described by Tolkmith and Britton18 gave the same results. In this case two isomers can be isolated with preparative chromatography on silica gel.15 A cis- and trans-isomers (Z and E isomers) would be expected as well. Both isomers 19 and 20 were anticipated to possess a cyclohexane ring conformation (Figure 4). The trans-isomer 20 exists in two different conformations.
X-ray structure determinations of the both isomers (19 and 20) provide interesting results. The trans-isomer adopts the expected centrosymmetric chair conformation with S atom in the equatorial positions and the cis-isomer is in a twist-conformation that possesses a two symmetry axis. Both (S atom and phenoxy–groups) are in the “isoclinals positions” (Figure 4).15
2. Monomethylhydrazine derivatives of phosphorus (III) and (V) compounds
Another interesting heterocycles are the compounds derivate from hydrazine, containing as well phosphorous and nitrogen atoms in the ring (for example the structure 21, Figure 5).15
Because of the fast proton exchange processes of the NH groups there is no possibility of monitoring conformational changes in solution by NMR-spectroscopy of molecules with this type of structures (21). The results of the X-ray diffraction determinations could be singular and maybe due to crystal packing effects too. But in N-methyl-substituted compounds, it could be expected that the NMR-spectra would reveal some more information.
The chemical reaction of monomethylhydrazine with phenoxy-thiophosphoryl-dichloride 22 (Figure 6) in the presence of base in excess, produces a mixture of three dihydrazides isomers (23, 24 and 25). These dihydrazides were isolated by chromatographic method.15
The molar ratio of 23:24:25 compounds is around 10:2:1, at a temperature between 0 °C and 40 °C. At lower temperatures (below 0 °C) only the compound 23 is formed. The identity of the isomers is easily recognized with NMR-spectroscopy.
From the compounds 23 and 24 other two heterocycles (26 and 27, Figure 7) containing phosphorus and nitrogen in the cycle could be synthesized. The process occurs at low temperature by the reaction of 23 and/or 24 in the presence of excess base. The symmetrical compound 26 exists also in the cis- and the trans-conformations. The same it was found in the case of the asymmetrical ring compound 27.15 The isomers of 27 can be separated with good results by column chromatography. They have the melting range between 135 °C and 161 °C. In the same time the mixture of the both isomers 26 and 27 has a melting range between 115 °C and 157 °C.
These compounds (26 and 27) could be also obtained by the dimerisation reaction of 28 (Figure 8), a monochloro-monohydrazido derivative.
3. Spirocycles containing two or more phosphorus atoms combination of hydrazine rings and cyclophosphazenes
Bifunctional compounds such as alkane-diols, diamines or aminoalcohols for instance yield spirocompounds. Hexachlorocyclotri(phosphazene) 29 is a known and easily accessible molecule that could reacts with organic amines (30) to form usually vicinal disubstituted products (31a and 31b, Figure 9).15 At room temperature in THF as solvent, the compound with the structure 31a (4,4,6,6-tetrachloro 6-phenoxy-cyclotri(phosphazene)-2-spiro-3-cyclodi(phosphadiazane)-6-sulfide) could be synthesized in about 70% yield. In the same time the compound with the structure 31b could be obtained as well.
The structure of the products 31a and 31b was proved by several spectroscopic methods (IR, NMR and MS). The hexachlorocyclotri(phosphazene) 29 also reacts directly with the absolute hydrazine to yield the dispiro-system 32 (Figure 10). The process occurs in a temperature range between –80 °C and 20 °C. The reaction process it takes 72 hours to be complete.15
Also in the literature are described heterocyclic compounds containing seven-membered ring (33, Figure 11). The compound 33 almost resembles a cyclophosphazene, but contains a N-N single-bond in the ring. It is described in the literature with its X-ray structure.15
The number of atoms which form the cycle could vary. For instance a four-membered hydrazine-ring could be formed in a (2–2) cycloaddition reaction of a phosphene (34) with an N-substituted azodicarbonimide (35). The resulting bicyclic molecule (36) may be considered a diphosphane hydrazine derivative (Figure 12).19
The corresponding phosphonic acid derivatives as diazaphosphiridine-3-oxides 38 are also known. They have been obtained from the N-chlorinated diamides 37 by HCl elimination (Figure 13).20 Moreover, the monosubstituted phosphanes (such as supermesitylphosphane for example) react with the diethylester of azodicarbonic acid to yield the diazaphosphoridine as intermediate. This compound spontaneously isomerizes to the corresponding diiminophosphorane.21
Another way to obtain such heterocycles with phosphorus and nitrogen in the ring is describes in the Figure 14. This is the formation of a five-membered ring by oxidation of a diamide of the phosphonic acid. This means that a new N-N bond is formed during the ring closure (39a and 39b). The hydrazine is not present in one of the starting materials, but is formed during the ring synthesis.22
Similar compounds containing phosphorus (III) and N-N single bonds in the ring have been synthesized below the room temperature, from iminophosphanes and organic azides with bulky substituents (the structure 40, Figure 15).23
Above the ambient temperature, these compounds lose nitrogen and are transformed into the corresponding bis(imino)phosphoranes.23-26 It should be mentioned here that as early as 1921, a reaction of triphenylphosphane with hydrogen azide led to a compound, that was described as a salt [(C6H5)3P-NH2]-N3,27 later turned out to be a 2–3 cycloaddition product (the structure 41, Figure 16) of triphenylphosphaneimine and hydrogen azide.28
A cyclic dimmer product obtained from the reaction of N-trimethylsilylpyrazole and phosphoruspentafluoride 42 (Figure 17) has a tricyclic structure (43, Figure 18).29
The ring adopts a flat boat conformation. The phosphorus atoms are octahedral coordinated.29 The by-product 43 proved to be an eight-membered ring molecule containing a P–O–P unit in the ring. The X-ray structure shows the central ring having a boat chair conformation (Figure 18).30
It could be mentioned also that quite a lot of interesting chemistry on the borderline between inorganic and organic chemistry has been published related to cyclic hydrazine derivatives, for instance Schiff–base derivatives of thiophosphoric acid dihydrazides and dendrimers and other polymers containing these and similar building blocks. Since the compounds are no “inorganic” ring systems in a rigid sense, no details are given here, but a few main publications are cited.31-37
4. Heterocycles containing phosphorus from N,N-bis[(–)-norpseudoephedrine]
The chemical reaction from the N,N-bis[(−)-norpseudoephedrine] oxalyl and P[NMe2]3 produces the epimer helix of tricyclic phosphorane 44 (Figure 19).38,39 The phosphorane 44 react with borane and produces further a mixture of two isomeric compounds: the structures 45 and 46. These phosphorane derivatives with structures 45 and 46 presented in the Figure 19, in borane excess finally could produce two reduced isomers: 47 and 48.39
The non-symmetrical optically active oxalyl derivative was reacted with dialkyldichlorotin compounds providing optically active binuclear diorganotin compounds, with a delocalized pentacyclic framework and two non-equivalent pentacoordinated (TBP) tin atoms.40 The metallic atoms are strongly coordinated by the oxygen atoms from the amide; the relevance is due to their stable rigid structures, suitable models for NMR and X-ray diffraction studies of hypervalent metallic atoms.
All of these compounds are interesting derived heterocycles from the family of ephedrines. These ligands have been used to obtain linear and cyclic organic derivatives, heterocycles and complexes with metal ions, all having high potential as optically active compounds. Their use as fine reagents for asymmetric synthesis or optically active catalytic agents in industry is a strong motivation for the study of these simple but in the same time relevant molecules. Much investigation is still needed in order to obtain a better understanding of the stereochemistry and of the dynamic behavior in the molecules resulting from chiral organic ligands and other elements different from carbon.38
5. Synthesis of novel chiral 1-phosphono-1,3-butadiene for asymmetric hetero Diels-Alder cycloadditions41
The chiral 1-phosphonodienes bearing a bicyclic (R,R)-1,3,2-dioxaphospholane or a (R,R)-1,3,2-diazaphospholidine auxiliary are important dienes for the asymmetric Diels–Alder processes. They showed a very high reactivity towards model nitroso and azodicarboxylate dienophiles. Aminophosphonic acids and their related compounds represent an important class of pharmacologically active molecules.41,42 These described chemical synthesis regarding α-aminophosphonic derivatives, despite the fact that the interest for β-, γ- and δ-homolog is still growing. As a part of a research program41,43 dedicated to the synthesis of aminophosphonic compounds, it was studied the hetero Diels-Alder (HDA) cycloadditions of achiral 1-phophonodienes with activated nitrogen containing dienophile (nitroso and azodicarboxylate compounds).44
The HDA reaction is very compatible with the asymmetric development.45,46 This led to consider the asymmetric HDA cycloadditions of nitrogencontaining dienophiles and chiral phosphonodienes as an entry towards aminophosphonic chirons. Several examples of chiral phosphonodienophiles are illustrated by Wyatt,47,48 and King49 with chirality either directly surrounding the phosphorous atom itself or being placed elsewhere on the chiral auxiliary. It exist only one previous report by Wyatt, in which the use of a chiral 4-phenyl-1-phosphonodiene was mentioned, versus a cyclic azodicarboxylate dienophile (4-phenyl-1,2,4-triazolin-3,5-dione) leading to the corresponding cycloadduct (with good yield but also with low stereoselectivity).47
Two different methods could be used to obtain a chiral phosphonodiene (Figure 20):
- the direct introduction of the chirality on the phosphorus atom (diene 49) (Figure 20).
- the introduction of the chirality on an adjacent atom, with a nitrogen relay nearby the phosphorus atom (e.g., diene 50) (Figure 20).
The first method (the direct introduction of the chirality on the phosphorus atom) was abandoned, because the synthesis of diene 50 produces in fact a mixture of diastereoisomers, impossible to be separated. In the second method, it was used C2-symmetry auxiliaries (bicyclic 1,3,2-dioxaphospholane)50 and 1,3,2-diazaphospholidines51 derived from (R,R)-1,2-dihydroxy- and (R,R)-1,2-diaminocyclohexane.
Few other chiral dienes (51a–d) have been selected for a study of their hetero Diels–Alder reactions with the dienophiles 52a–c, to obtain the isomers 53 (R) and 54 (S). All of these structures (51–54) are presented in the Figure 21, where where R’ is H in 51a, Me in 51b, Pr in 51c and Bn in 51d, R’’ is Me in 52a, 2–Tol in 52b and COOMe in 52c, and Y is O in 52a and 52b, and NR’’ in 52c.
For the both conformers of dienes 51a–d, the substituents attached to the nitrogen atoms were found in a pseudo–equatorial position. In agreement with the previous observations (Bennani and Hanessian)51 there is an obvious differentiation of the preferred orientation of R’ groups on either size of the chiral auxiliary.
By increasing the hydrophobicity of the R’ groups of the diene, a stronger sterical hindrance appears and an increase in the selectivity was observed. For the dienophile 52a the selectivity increased twice passing from diene 51a to diene 51d, while for dienophile 52b the increase in selectivity was more pronounced when going from diene 51a to diene 51d.
The cycloadditions of dienophile 52c with dienes 51a–d clearly showed a synergy effect due to the steric hindrance of both the R’ and R’’ substituents. The highest level of stereo selectivity was obtained with diene 51d.
This prediction has been confirmed. It was synthesized in two steps the chiral diene 51d, from the compound 55, in 62% yield (Figure 22). The structure of the product 51d was confirmed by X-ray diffraction analysis.
Experimentally, under classical thermal conditions, the reaction of diene 51d with 52b led to a 1:1 mixture of separable diastereomers 56 and 57, after 12 hours in refluxing (Figure 22). This can be attributed to a low energetic discrimination between the both conformers of chiral diene 51d and to the high asynchronicity of bond formation with the nitroso-partner.41 The selectivity was not influenced by the polarity of the solvent.41
CONCLUSIONS
The present paper makes an overview of literature data from the last decades, for different synthesis methods and properties of several classes of heterocycles containing phosphorus in the ring. Many compounds described here contain phosphorus and nitrogen in the cycle.
The work in the field of heterocycles with phosphorus atoms in the ring contributes to the general image of the ring structures and the ring conformations. The importance of hydrazine and their derivatives as ring building has been described as well.
The combination of different ligands derived from ephedrine produces new heterocyclic compounds, optically active. These heterocycles can be used as fine reagents for asymmetric synthesis or optically active catalytic agents in the industry. This is a strong reason to study more these compounds, in order to understand their stereochemistry and their behavior.
All of these compounds containing phosphorus described are new interesting heterocycles with many applications.
References
1. D. Vijay and G. N. Sastry, J. Phys. Chem. A, 2006, 110, 10148. CrossRef
2. L. McFail-Isom, X. Shui, and L. D. Williams, Biochemistry, 1998, 37, 17105. CrossRef
3. C. W. Bock, A. K. Katz, G. D. Markham, and J. P. Glusker, J. Am. Chem. Soc., 1999, 121, 7360. CrossRef
4. J. J. R. Frausto da Silva and R. J. P. Williams, 'The Biological Chemistry of the Elements. The Inorganic Chemistry of Life', Clarendon Press, Oxford, 1991.
5. J. P. Gallivan and D. A. Dougherty, Proc Natl Acad Sci U S A, 1999, 96, 9459. CrossRef
6. D. A. Dougherty, Science, 1996, 271, 163. CrossRef
7. S. K. Kim, S. Hu, P. Tarakeshwar, and J. Y. Lee, Chem. Rev., 2000, 100, 4145. CrossRef
8. H. S. Choi, S. B. Suh, S. J. Cho, and K. S. Kim, Proc Natl Acad Sci USA, 1998, 95, 12094. CrossRef
9. A. S. Reddy and G. N. Sastry, J. Phys. Chem. A, 2005, 109, 8893. CrossRef
10. C. Ruan and M. T. Rodgers, J. Am. Chem. Soc., 2004, 126, 14600. CrossRef
11. R. C. Dunbar, J. Phys. Chem. A, 2000, 104, 8067. CrossRef
12. F. Costanzo, R. G. Della Valle, and V. Barone, J. Phys. Chem. B, 2005, 109, 23016. CrossRef
13. (a) S. Choua, H. Sidorenkova, T. Berclaz, M. Geoffroy, P. Rosa, N. Mezailles, L. Ricard, F. Mathey, and P. Le Floch, J. Am. Chem. Soc., 2000, 122, 12227; CrossRef (b) G. B. Deacon, E. E. Delbridge, G. D. Fallon, C. Jones, D. E. Hibbs, M. B. Hursthouse, B. W. Skelton, and A. H. White, Organometallics, 2000, 19, 1713. CrossRef
14. D. Gudat, V. Bajorat, S. Hap, M. Nieger, and G. Schroder, J. Inorg. Chem., 1999, 38, 1169.
15. U. Engelhardt, Coord. Chem. Rev., 2002, 235, 53. CrossRef
16. R. Appel and G. Berger, Chem. Ber., 1958, 91, 1339. CrossRef
17. H. Bock and G. Rudolf, Chem. Ber., 1961, 94, 1497. CrossRef
18. H. Tolkmith and E. C. Britton, J. Org. Chem., 1959, 24, 705. CrossRef
19. L. Weber, H. Bastian, R. Boese, H. G. Stammler, and B. Neumann, Chem. Ber., 1992, 125, 1821. CrossRef
20. H. Quast, M. Heuschmann, and M. O. Abdel-Rahman, Liebigs. Ann. Chem., 1981, 5, 943. CrossRef
21. J. Navech and M. Revel, Tetrahedron Lett., 1986, 27, 2863. CrossRef
22. U. Engelhardt, M. Kretschmann, A. Simon, and B. Scheffler, Phosphorus Sulfur., 1994, 93, 385. CrossRef
23. (a) M. Kretschmann and U. Engelhardt, unpublished results; (b) M. Kretschmann, Diplomarbeit: Fachber. Chemie, Freie Univ. Berlin, 1992.
24. E. Niecke and H. G. Schafer, Chem. Ber., 1982, 115, 185. CrossRef
25. E. Niecke, V. von der Gonna, and M. Nieger, Chem. Ber., 1990, 123, 2329. CrossRef
26. E. Niecke and G. Gudat, Angew. Chem., 1991, 30, 217. CrossRef
27. E. Niecke, M. Frost, M. Nieger, V. von der Gonna, A. Ruban, and W. Schoeller, Angew. Chem., 1994, 33, 2111. CrossRef
28. H. Staudinger and E. Hauser, Helv. Chim. Acta, 1921, 4, 861. CrossRef
29. J. E. Leffer, U. Honsberg, Y. Tsuno, and I. Forsblad, J. Org. Chem., 1961, 26, 4810. CrossRef
30. M. Well, P. G. Jones, and R. Schmutzler, Fluorine Chem., 1991, 53, 261. CrossRef
31. U. Engelhardt and B. Stromburg, Acta Crystallogr., 1993, Sect. C 49, 1643.
32. J.-P. Majoral, M. Revel, R. Kraemer, H. Germa, and J. Navech, J. Heterocycl. Chem., 1977, 14, 749. CrossRef
33. D. Colombo, A.-M. Caminade, and J.-P. Majoral, J. Inorg. Chem., 1991, 30, 3365. CrossRef
34. J.-P. Majoral, M. Badri, A.-M. Caminade, M. Delmas, and A. Gaset, J. Inorg. Chem., 1991, 30, 344. CrossRef
35. B. Oussaid, B. Garrigues, A.-M. Caminade, and J.-P. Majoral, Phosphorus Sulfur., 1992, 73, 41. CrossRef
36. M. Slany, M. Bardaji, M.-J. Casanove, A.-M. Caminade, J.-P. Majoral, and B. Chaudet, J. Am. Chem. Soc., 1995, 117, 9764. CrossRef
37. J.-P. Majoral and A.-M. Caminade, Chem. Rev., 1999, 99, 845. CrossRef
38. R. Contreras, A. Flores-Parra, H. C. Lopez-Sandoval, and N. Barba-Behrens, Coordin. Chem. Rev., 2007, 251, 1852. CrossRef
39. M. Tlahuextl, F. J. Martinez-Martinez, M. J. Rosales-Hoz, and R. Contreras, Phosphorus Sulfur., 1997, 123, 5. CrossRef
40. V. M. Jimenez-Perez, H. Noth, A. Ariza-Castolo, A. Flores-Parra, and R. Contreras, J. Organomet. Chem., 2006, 691, 1584. CrossRef
41. J.-C. Monbaliu, B. Tinant, D. Peeters, and J. Marchand-Brynaert, Tetrahedron Lett., 2010, 51, 1052. CrossRef
42. V. Kukhar and H. Hudson, Aminophosphonic and Aminophosphinic Acids: Chemistry and Biological Activity, Wiley: New York, 2000.
43. J.-C. Monbaliu, R. Robiette, D. Peeters, and J. Marchand-Brynaert, Tetrahedron Lett., 2009, 50, 1314. CrossRef
44. J.-C. Monbaliu and J. Marchand-Brynaert, Tetrahedron Lett., 2008, 49, 1839. CrossRef
45. F. Fringuelli and A. Taticchi, ʻThe Diels-Alder Reactionʼ, Wiley: New York, 2002.
46. H. Yamamoto and M. Kawasaki, Bull. Chem. Soc. Jpn., 2007, 80, 595. CrossRef
47. P. B. Wyatt, C. Villalonga-Barber, and M. Motevalli, Tetrahedron Lett., 1999, 40, 149. CrossRef
48. E. W. C. Cheng, R. T. Mandalia, M. Motevalli, B. Mothia, Y. Patanwadia, and P. Wyatt, Tetrahedron, 2006, 62, 12398. CrossRef
49. R. W. Ware and S. B. King, J. Org. Chem., 2000, 65, 8725. CrossRef
50. M. Maffei and G. Buono, Tetrahedron, 2003, 59, 8821. CrossRef
51. Y. Bennani and S. Hanessian, Tetrahedron, 1996, 52, 13837. CrossRef