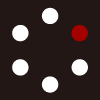
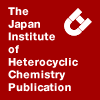
HETEROCYCLES
An International Journal for Reviews and Communications in Heterocyclic ChemistryWeb Edition ISSN: 1881-0942
Published online by The Japan Institute of Heterocyclic Chemistry
e-Journal
Full Text HTML
Received, 26th October, 2010, Accepted, 24th November, 2010, Published online, 26th November, 2010.
DOI: 10.3987/COM-10-12090
■ A Convenient Method for Synthesis of 5-Chloro-2-aryloxazole-4-carbaldehyde with Vilsmeier Reagent
Can Jin, Jun Chen, and Weike Su*
Key Laboratory of Pharmaceutical Engineering of Ministry of Educations, College of Pharmaceutical Sciences, Zhejiang University of Technology, Hangzhou 310014, China
Abstract
A convenient method for synthesis of 5-chloro-2-aryloxazole-4-carbaldehyde 3 in moderate to good yields had been developed via the Vilsmeier reaction of readily available N-arylglycine 2 at 100 oC, provided a novel route for the construction of nitrogen heterocycles. A series of these compounds were synthesized by this method and optimization of conditions was performed.In the previous work, our group1 had described a mild and efficient method for the synthesis of substituted 1,4-dichloroisoquinolines and 5-aryloxazole-4-carboxaldehydes2,3 from various phenacyl azides under Vilsmeier conditions. But there were few publications refer to the 5-chloro-2-aryloxazole-4-carbaldehyde. In 1993 Gerrit L'abbé et al.4 had reported that 5-chlorooxazole-4-carbaldehyde was afforded by 5-methoxyoxazole under Vilsmeier reaction which was from methyl benzoylaminoacetate dehydrated to by a known procedure.5 Furthermore, we got inspiration from an article6 about a novel synthesis of 2-butyl-5-chloro-3H-imidazole-4-carbaldehyde which is a key intermediate for the synthesis of the Angiotensin II Antagonist Losartan. In this article, Gareth J. Griffiths6 proposed that the synthesis of 2-butyl-5-chloro-3H-imidazole-4-carbaldehyde used (Pentanimidoylamino)acetic acid, and got a better yield. Hence, we had a try to synthesize the similar construction of oxazole derivatives from glycine. Fortunately, we found that a series of 5-chloro-2-aryloxazole-4-carbaldehyde compounds were gained with Vilsmeier reagent in good yield.
In our article, we prepared Vilsmeier reagent from bis(trichloromethyl) carbonate (BTC, triphosgene) and DMF. As is well known, Vilsmeier reagent has been found extensive application in the organic synthesis. It was used for the introduction of formyl group in active aromatic and heteroaromatic compounds.7 Subsequently, the utilizations had been exploited in chlorination,8 chlorformylation,9,10 aromatization11 and cyclizations.12 Meanwhile, bis(trichloromethyl) carbonate’s use had been proved much safer and more environmentally friendly13 than traditional reagents, such as phosgene, POCl3.
We had isolated the appropriate N-acyl derivatives 2a-l which were synthesized from commercially available glycine with aromatic acid chlorides in the presence of 10% aqueous NaOH.14-16 N-Arylglycine 2a-l were used as substrates to generate 5-chloro-2-aryloxazole-4-carbaldehyde 3a-l with BTC and DMF under appropriate conditions (Scheme 1).
Next, we investigated various condition including the effect of solvents and reaction conditions in the synthesis of 5-chloro-2-benzoyloxazole-4-carbaldehyde (3a). According to Table 1, after screening a variety of ratios, a 1:3:1 ratio of BTC /DMF /N-benzoylglycine (2a) was determined to be suitable. If the ratio of 2a was increased, the yield was reduced obviously (Table 1, Entry 5). In the same time, the reaction would be taken place much better at 100 oC than 60 oC or room temperature (Table 1, Entries 1-4). Moreover, to determine the most appropriate solvent, we chosen several kinds of solvents (toluene, CH2Cl2, MeCN, CCl4, THF), and toluene proved to be the best in all cases (Table 1, Entry 3). The work-up in toluene was much more comfortable than in other solvents (Table 1, Entries 7-10). Finally, we found the optimized conditions for this reaction as following: 1:3:1 mol ratio of BTC, DMF and N-benzoylglycine (2a) in dry toluene at 100 oC for 3 hours.
To extend the scope of generality of this procedure, a series of N-arylglycine were investigated. Based on the results in Table 1, we explored the scope of the substrates under the optimized conditions (Table 2). As expected, most of N-arylglycine worked well under the reaction condition. Obviously, the N-aryl- glycine possessing electron-donating functional groups were found to give higher yields than those of electron-withdrawing groups. Meanwhile, we found that steric hindrance of the substituent groups on the aromatic rings had enormous effect on the efficiency. The ortho-substituted aromatic ring of substrates hardly gave the products (Table 2, Entries 11-12).
Furthermore, we compared the yields of product 3a which were prepared from the glycine and glycine methyl ester, respectively. Interestingly, N-benzoylglycine was found to give higher yield than those of N-benzoylglycine methyl ester as the raw material (Table 2, Entry 1).
For proving the results above, we selected several representational functional groups, including chloro, nitro, fluo, methoxy, methyl,t-Bu. In comparison, using N-benzoylglycine methyl ester instead of N-arylglycine as the substrate provides lower yields (Table 2, Entries 2, 4, 6, 7, 8, 10).
On the other hand, using POCl3 provides a bit higher yield probably due to the higher reactivity of the corresponding iminium salts. For instance, 76% yield of 5-chloro-2-benzoyloxazole-4-carbaldehyde (3a) was gained under POCl3/DMF system. However, 71% yield of it under BTC/DMF system. But, unfortunately, the ortho-substituted aromatic ring of substrates still could not be prepared under POCl3/DMF system (Table 2, Entries 11-12).
In conclusion, we have developed a novel and convenient method for the synthesis of 5-chloro-2-aryloxazole-4-carbaldehyde from N-arylglycine under BTC and DMF system. The structures of the compounds were established by 1H-NMR, 13C NMR, IR and MS (All unknown compounds were further confirmed by HRMS (EI)). This method offers significant advantages including mild conditions, more environmentally friendly bis(trichloromethyl) carbonate (BTC, triphosgene) instead of traditional reagents, and good yields.
EXPERIMENTAL
Melting points were determined by Büchi B-540 melting point apparatus and are uncorrected. The IR spectra (KBr pellet) were measured on a Nicolet Avatar-370 spectrometer. 1H NMR (400 MHz) and 13C NMR (100 MHz) spectra were recorded on a Varian 400-MHz spectrometer. Mass spectra were obtained on a Thermo Finnigan LCQ-Advantage spectrometer (ESI, APCI) or a Finnigan Trace DSQ spectrometer (EI, CI). HRMS was carried out on an APEX (Bruker) mass III spectrometer.
Typical procedure for the Preparation of N-aryl glycine 2a
To a solution of glycine (3.0 g, 40.0 mmol) in 10% aqueous sodium hydroxide solution (50 mL) was added benzoyl chloride (7.0 mL, 60.3 mmol), and stirred vigorously for 5 h at room temperature. Cool water (50 g) was added into the solution, then concentrated HCl was added dropwise until the mixture was acidified (pH 1-2). White crystalline solid as the resulting compound was obtained.
Typical procedure for the Preparation of 5-chloro-2-aryloxazole-4-carbaldehyde 3a
The Vilsmeier type reagent was prepared by adding dropwise of a solution of BTC (4.5 g, 15 mmol) in toluene (25 mL) into DMF (3.3 g, 45 mmol) in toluene (10 mL) at 0 oC, and then stirring at room temperature for 20 min. The compound was added into the Vilsmeier type reagent and heated to 90 oC for 4 h. The brown reaction mixture was cooled to room temperature and then poured cautiously into ice-water (60 cm3). The phases were separated and the aqueous phase was extracted with toluene (3×30 mL). The combined extracts were washed with water (3×30 mL) and evaporated. The residue was purified by column chromatography on silica gel using petroleum ether: EtOAc (10:1) as the eluent to get the compound.
5-Chloro-2-phenyloxazole-4-carbaldehyde (3a)
White solid. mp 92-94 oC (Lit.,6 mp 91-93 oC). IR (KBr): 3422, 3073, 1688, 1417 cm-1. 1H NMR (400 MHz, CDCl3) δ: 9.99 (s, 1H), 8.06 (d, 2H, J = 6.8 Hz), 7.48-7.56 (m, 3H). 13C NMR (100 MHz, CDCl3) δ: 182.1, 161.1, 143.0, 133.9, 131.8, 129.0, 126.7, 125.4. MS (EI): m/z (%) = 209 ([M++2], 11), 207(M+, 31), 172(100), 116(98), 89(96).
5-Chloro-2-(3-chlorophenyl)oxazole-4-carbaldehyde (3b)
Yellow solid. mp 132-133 oC. IR (KBr): 3435, 3067, 1694, 1556 cm-1. 1H NMR (400 MHz, CDCl3) δ: 9.96 (s, 1H), 8.04 (t, J = 8.0 Hz, 1H), 7.91-7.93 (dt, J1 = 7.6 Hz, J2 = 1.2 Hz, 1H), 7.47-7.50 (dt, J = 8.4 Hz, J = 1.6 Hz, 1H), 7.42 (t, J = 8.0 Hz, 1H). 13C NMR (100 MHz, CDCl3) δ: 181.7, 159.4, 143.0, 135.1, 133.8, 131.7, 130.2, 126.5, 124.7. MS (EI): m/z (%) = 243 ([M++2], 19), 241(M+, 30), 206(100), 150(91), 123(71). HRMS (EI): calcd for C10H5Cl2NO2[M+H]+: 240.9697, found: 240.9705.
5-Chloro-2-(4-chlorophenyl)oxazole-4-carbaldehyde (3c)
Yellow solid. mp 145-146 oC. IR (KBr): 3432, 3091, 1698, 1544 cm-1. 1H NMR (400 MHz, CDCl3) δ: 9.95 (s, 1H), 7.98 (d, J = 7.2 Hz, 2H), 7.46 (d, J = 8.8 Hz, 2H). 13C NMR (100 MHz, CDCl3) δ: 181.7, 159.9, 147.6, 138.0, 133.8, 129.3, 128.2, 127.8. MS (EI): m/z (%) = 241(M+, 20), 240([M+-1], 36), 222(100), 221(50). HRMS (EI): calcd for C12H8NO3 [M+H]+: 240.9697, found: 240.9705.
5-Chloro-2-(4-nitrophenyl)oxazole-4-carbaldehyde (3d)
White solid. mp 142-143 oC. IR (KBr): 3447, 3104, 1699, 1525 cm-1. 1H NMR (400 MHz, CDCl3) δ: 9.99 (s, 1H), 8.35 (d, J = 7.2 Hz, 2H), 8.24 (d, J = 8.8 Hz, 2H). 13C NMR (100 MHz, CDCl3) δ: 181.6, 158.5, 149.3, 143.8, 134.2, 130.5, 127.4, 124.2. MS (EI): m/z (%) = 253([M++1], 30), 252(M+, 100), 251(30). HRMS (EI): calcd for C10H5ClN2O4 [M+H]+: 251.9938, found: 251.9939.
5-Chloro-2-m-tolyloxazole-4-carbaldehyde (3e)
White solid. mp 101-102 oC. IR (KBr): 2923, 2574, 1687, 1589 cm-1. 1H NMR (400 MHz, CDCl3) δ: 9.94 (s, 1H), 7.81-7.96 (m, 2H), 7.28-7.41 (m, 2H), 2.41 (s, 3H). 13C NMR (100 MHz, CDCl3) δ: 181.8, 162.6, 147.5, 144.6, 134.4, 132.0, 130.5, 128.6, 127.2, 123.8, 21.3. MS (EI): m/z (%) = 203([M++2], 12), 221(M+, 34), 136(42), 119(100), 91(75). HRMS (EI): calcd for C11H8ClNO2 [M+H]+: 221.0244, found: 221.0249.
5-Chloro-2-p-tolyloxazole-4-carbaldehyde (3f)
White solid. mp 107-108 oC. IR (KBr): 3434, 2846, 1699, 1550 cm-1. 1H NMR (400 MHz, CDCl3) δ: 9.98 (s, 1H), 7.95 (d, J = 8.2 Hz, 2H), 7.30 (d, J = 8.2 Hz, 2H), 2.43 (s, 3H). 13C NMR (100 MHz, CDCl3) δ: 182.1, 161.4, 142.5, 133.8, 126.7, 122.6, 21.6. MS (EI): m/z (%) = 223([M++2], 15), 221(M+, 41), 186(55), 130(100), 103(88). HRMS (EI): calcd for C11H8ClNO2 [M+H]+: 221.0244, found: 221.0249.
5-Chloro-2-(4-fluorophenyl)oxazole-4-carbaldehyde (3g)
Brown solid. mp 133-134 oC. IR (KBr): 3000, 2677, 1682, 1430 cm-1. 1H NMR (400 MHz, CDCl3) δ: 9.98 (s, 1H), 8.07 (dt, J = 7.2 Hz, J = 2.0 Hz, 2H), 7.17-7.22 (dt, J = 8.8 Hz, J = 2.0 Hz, 2H), 13C NMR (100 MHz, CDCl3) δ: 182.0, 166.1, 163.6, 133.9, 129.1, 129.0, 121.8, 116.5, 116.3, MS (EI): m/z (%) = 227([M++2], 10), 225(M+, 28), 190(100), 134(86), 107(90). HRMS (EI): calcd for C10H5ClFNO2 [M+H]+: 224.9993, found: 225.0002.
5-Chloro-2-(4-methoxyphenyl)oxazole-4-carbaldehyde (3h)
White solid. mp 110-111 oC. IR (KBr): 3423, 2925, 1699, 1589 cm-1. 1H NMR (400 MHz, CDCl3) δ: 9.94 (s, 1H), 7.98 (d, J = 9.2 Hz, 2H), 6.97 (d, J = 9.2 Hz, 2H), 3.87 (s, 1H). 13C NMR (100 MHz, CDCl3) δ: 181.8, 162.3, 161.1, 147.3, 133.7, 128.8, 128.4, 117.9, 114.4, 55.5. MS (EI): m/z (%) = 239([M++2], 33), 237(M+, 100), 180(62), 119(27). HRMS (EI): calcd for C11H8ClNO3 [M+H]+: 237.0193, found: 237.0198.
2-(4-Bromophenyl)-5-Chlorooxazole-4-carbaldehyde (3i)
Brown solid. mp 149-150 oC. IR (KBr): 3446, 2923, 1698, 1542 cm-1. 1H NMR (400 MHz, CDCl3) δ: 9.95 (s, 1H), 7.90 (d, J= 8.8Hz, 2H), 7.63 (d, J= 8.4Hz, 2H). 13C NMR (100 MHz, CDCl3) δ: 181.7, 160.0, 142.9, 133.9, 132.2, 127.9, 124.1. MS (EI): m/z (%) = 285(M+, 42), 283(30), 282(100), 277(45). HRMS (EI): calcd for C10H5BrClNO2 [M+H]+: 284.9192, found: 284.9205.
2-(4-tert-Butylphenyl)-5-Chlorooxazole-4-carbaldehyde (3j)
White solid. mp 65-66 oC. IR (KBr): 3381, 2966, 1704, 1547 cm-1. 1H NMR (400 MHz, CDCl3) δ: 9.95 (s, 1H), 7.96 (d, J= 8.8Hz, 2H), 7.49 (d, J= 8.8Hz, 2H), 1.35 (s, 9H). 13C NMR (100 MHz, CDCl3) δ: 181.8, 161.0, 155.2, 142.5, 133.7, 126.7, 126.3, 125.8, 35.1, 31.1. MS (EI): m/z (%) = 263(M+, 33), 261(100), 169(35). HRMS (EI): calcd for C14H14ClNO2 [M+H]+: 263.0713, found: 263.0721.
ACKNOWLEDGEMENTS
We are grateful to the National Natural Science Foundation of China (No 20876147) and Natural Science Foundation of Zhejiang Province (No Y4090346) for financial support.
References
1. R. E. Chen, X. H. Zhou, W. H. Zhong, and W. K. Su, Org. Prep. Proced. Int., 2008, 40, 580. CrossRef
2. V. J. Majo and P. T. Perumal, J. Org. Chem., 1998, 63, 7137. CrossRef
3. V. J. Majo and P. T. Perumal, Tetrahedron Lett., 1997, 38, 6890. CrossRef
4. G. L'abbé, Anna-Maria, Ilisiu, W. Dehaen, and S. Toppet, J. Chem. Soc., Perkin Trans. 1, 1993, 2259.
5. J. W. Cornforth in The Chemistry of Penicillin, Princeton University Press, Princeton N.J., 1949, 671.
6. G. J. Griffiths, M. B. Hauck, R. Imwinkelried, J. Kohr, C. A. Roten, and G. C. Stucky, J. Org. Chem., 1999, 64, 8087. CrossRef
7. V. J. Traynelis, J. Miskel, and J. Sowa, J. Org. Chem., 1957, 22, 1269. CrossRef
8. E. Cadis, A. V. Cheorghe, and L. Fey, Rev. Chim. (Bucharest), 1987, 38, 264.
9. A. R. Katritrky, C. M. Marson, and Q. W. Zao, J. Org. Chem., 1987, 52, 2730. CrossRef
10. G. Barman and J. K. Ray, Tetrahedron Lett., 2010, 51, 297. CrossRef
11. B. Raju and G. S. Krishna, Indian J. Chem., Sect. B, 1987, 26B, 892.
12. A. Ermili, A. J. Castro, and P. A. Westfall, J. Org. Chem., 1965, 30, 339. CrossRef
13. W. K. Su, Y. Y. Weng, C. Zheng, Y. Zhang, F. Shi, B. Hong, Z. W. Chen, J. J. Li, and Z. H. Li, Org. Prep. Proced. Int., 2009, 41, 96.
14. M. A. Mesaik, S. Rahat, K. M. Khan, Zia-Ullah, M. I. Choudhary, S. Murad, Z. Ismail, Atta-ur-Rahman, and A. Ahmad, Bioorg. Med. Chem., 2004, 12, 2050.
15. S. E. Yoo, S. H. Lee, and N. J. Kim, Tetrahedron Lett., 1988, 29, 2195. CrossRef
16. E. G. Mata and O.E. Mascaretti, Tetrahedron Lett., 1988, 29, 6893. CrossRef