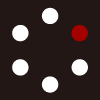
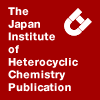
HETEROCYCLES
An International Journal for Reviews and Communications in Heterocyclic ChemistryWeb Edition ISSN: 1881-0942
Published online by The Japan Institute of Heterocyclic Chemistry
e-Journal
Full Text HTML
Received, 26th October, 2010, Accepted, 24th November, 2010, Published online, 26th November, 2010.
DOI: 10.3987/COM-10-12091
■ Palladium-Catalyzed Cyanation of Aryl Bromides Using Phosphine-Free Pyridylhydrazone Ligands
Takashi Mino,* Tomoko Koizumi, Masanori Shibuya, Kiminori Hirai, Masami Sakamoto, and Tsutomu Fujita
Faculty of Engineering, Chiba University, 1-33 Yayoi-cho, Inage-ku, Chiba 263-8522, Japan
Abstract
Palladium-catalyzed cyanation of aryl bromides with potassium hexacyanoferrate(II) trihydrate, K4[Fe(CN)6]·3H2O as the cyanide source gave benzonitrile derivatives using a catalytic amount of Pd(OCOCF3)2 in DMF at 110 °C with phosphine-free pyridyl-hydrazone ligand 2c in good yields.Benzonitrile derivatives are valuable intermediates in organic synthesis, such as dyes, pharmaceuticals, agrochemicals, and natural products, and can be easily transformed to benzoic acids, benzoic esters, amides, amines, and aldehydes. The traditional method for preparing benzonitriles from corresponding aryl bromides and iodides requires stoichiometric copper(I) cyanide under harsh conditions known as the Rosenmund-von Braun reaction.1 Therefore, some transition metal catalyzed methods have been developed, such as palladium-, nickel-, and copper-catalyzed methods using KCN,2 NaCN,3 Zn(CN)2,4 and trimethylsilyl cyanide5 as the cyanide source. However, most work has concentrated on the traditional inconvenient cyanide sources, which have severe drawbacks. To avoid these problems, potassium hexacyanoferrate(II) was rediscovered as a non-toxic cyanide source using Pd(OAc)2/DPPF under a N2 atmosphere by Beller;6 the reactions of aryl halides using Pd(OAc)2 with other phosphine ligands were also reported.7 However, phosphine ligands are often air-sensitive. Although phosphine-free palladium catalytic systems have also been reported,8 these reactions sometimes require harsh heating conditions or microwave irradiation conditions. And also dried potassium hexacyanoferrate(II) was used sometimes instead of hydrate under the reaction.8h
On the other hand, we recently demonstrated air-stable phosphine-free hydrazone as an effective ligand for palladium-catalyzed C-C bond formations.9 We now report the use of phosphine-free bishydrazone ligands 1 and pyridyl-hydrazone ligands 2 for a palladium catalyzed cyanation of aryl bromides with potassium hexacyanoferrate(II) trihydrate, K4[Fe(CN)6]·3H2O as the cyanide source under aerobic conditions at 110 °C.
Initially, we sought the optimal reaction conditions for the palladium-catalyzed cyanation of aryl bromides with potassium hexacyanoferrate(II) trihydrate, K4[Fe(CN)6]·3H2O as the cyanide source. 4-Bromotoluene was chosen as a model substrate with 2 mol% of Pd catalyst for 18 h under aerobic conditions at 110 °C (Table 1). Using 2 mol% of indoline-derived bishydrazone 1a as a ligand, we observed that cyanation in the presence of Pd(OAc)2 with 2 equiv. of K3PO4 as a base in DMF as a solvent proceeded to give p-tolunitrile (3a) in 75% yield with a small amount of 4,4’-dimethylbiphenyl (4) as a by-product (Table 1, Entry 1). Several commonly used bases were tested (Entries 1-4); K3PO4 preferred this reaction (Entry 1). The effect of various solvents was also investigated (Entries 1, and 5-7). The use of DMF as a solvent led to high yield for this reaction (Entry 1). We tested bishydrazone 1b and pyridyl-hydrazones 2a-d (Entries 9-12) and found that pyridyl-hydrazone 2c was a very effective ligand for this reaction (Entry 11). The effect of various palladium sources was also investigated (Entries 11, and 13-15). The use of Pd(OCOCF3)2 led to high yield for this reaction (Entry 15). We found the following optimized conditions; using the Pd(OCOCF3)2/pyridyl-hydrazone 2c system, the reaction proceeded with 83% in DMF at 110 °C (Entry 15).
To evaluate the scope and limitations of this procedure, the reactions of various aryl bromides were investigated (Table 2). Using 1-bromo-4-alkylbenzenes and p-bromobiphenyl led to good yields of corresponding benzonitriles (Entries 1-4) including such fragrance compounds as p-isopropylbenzonitrile (cumin nitrile) (3b). Moreover, aryl bromides with methoxy and phenoxy groups at the para-position also led to good yields (Entries 5 and 6). On the other hand, aryl bromides with electron-withdrawing groups, such as p-bromobenzophenone and 1-bromo-4-chlorobenzene, led to moderate yields (Entries 7 and 8). We also investigated the reaction of 3-substituted and 2-substituted aryl bromides (Entries 9-13). Although the reaction with o-bromoanisole gave a small amount of homocoupling product instead of corresponding product (Entry 13), the other reactions gave corresponding products in moderate to good yields. Using 1-bromonaphthalene also led to good yield of the corresponding nitrile (Entry 14).
In conclusion, we found that the palladium-catalyzed cyanation of aryl bromides with potassium hexacyanoferrate(II) trihydrate, K4[Fe(CN)6]·3H2O as the cyanide source gave benzonitrile derivatives using a catalytic amount of Pd(OCOCF3)2 in DMF at 110 °C under aerobic conditions with phosphine-free pyridyl-hydrazone ligand 2c in good yields.
EXPERIMENTAL
General
1H and 13C NMR spectra were recorded on a Bruker DPX-300 spectrometer. Chemical shifts are reported in δ ppm referenced to an internal SiMe4 standard. Infrared (IR) spectra were obtained using a JASCO FT/IR 230 spectrophotometer. Mass spectra were recorded on a GCMS-QP 5050. Hydrazone ligands 19c,d and 29b,c,d,e were prepared according to the literature methods.
General procedure of palladium-catalyzed cyanation of aryl bromides.
To a mixture of K4[Fe(CN)6]·3H2O (169.5 mg, 0.4 mmol), K3PO4 (424 mg, 2.0 mmol), Pd(OCOCF3)2 (6.69 mg, 20 µmol), and ligand 2c (4.26 mg, 20 µmol) in DMF (2.0 mL) was added aryl bromide (1.0 mmol) at room temperature under an air. The mixture was stirred at 110 °C. After 18 h, the mixture was diluted with EtOAc and water. The organic layer was washed with brine, dried over MgSO4, and concentrated under reduced pressure. The residue was purified by silica gel chromatography (hexane or hexane/EtOAc = 20-100/1).
p-Tolunitrile (3a)2a: 73% as a white solid; mp 25-26 °C; 1H NMR (CDCl3) δ: 2.42 (s, 3H), 7.27 (d, J = 8.0 Hz, 2H), 7.54 (d, J = 8.2 Hz, 2H); 13C NMR (CDCl3) δ: 21.8, 109.3, 119.1, 129.8, 132.0, 143.6; IR (KBr): (CN) 2226 cm-1; EI-MS m/z (rel intensity): 117 (M+, 100).
p-Isopropylbenzonitrile (3b)10: 71% as a colorless liquid; 1H NMR (CDCl3) δ: 1.26 (d, J = 6.9 Hz, 6H), 2.88-3.07 (m,1H), 7.32 (d, J = 8.4 Hz, 2H), 7.58 (d, J = 8.4 Hz, 2H); 13C NMR (CDCl3) δ: 23.5, 34.4, 109.6, 119.2, 127.3, 132.2, 154.3; IR (neat): (CN) 2227 cm-1; EI-MS m/z (rel intensity): 145 (M+, 24).
p-tert-Butylbenzonitrile (3c)7c: 72% as a colorless liquid; 1H NMR (CDCl3) δ: 1.33 (s, 9H), 7.48 (d, J = 8.6 Hz, 2H), 7.59 (d, J = 8.6 Hz, 2H); 13C NMR (CDCl3) δ: 30.9, 35.3, 109.3, 119.1, 126.1, 132.0, 156.6; IR (neat): (CN) 2227 cm-1; EI-MS m/z (rel intensity): 159 (M+, 21).
p-Phenylbenzonitrile (3d)2b: 70% as a white solid; mp 81-82 °C; 1H NMR (CDCl3) δ: 7.39-7.53 (m, 3H), 7.56-7.62 (m, 2H), 7.66-7.75 (m, 4H); 13C NMR (CDCl3) δ: 110.8, 118.9, 127.2. 127.7, 128.6, 129.1, 132.6, 139.1, 145.6; IR (KBr): (CN) 2225 cm-1; EI-MS m/z (rel intensity): 179 (M+, 100).
p-Methoxybenzonitrile (3e)2b: 84% as a white solid; mp 52-53 °C; 1H NMR (CDCl3) δ: 3.87 (s, 3H), 6.92-6.99 (m, 2H), 7.64-7.57 (m, 2H); 13C NMR (CDCl3) δ: 55.5, 104.0, 114.7, 119.2, 134.0, 162.8; IR (KBr): (CN) 2217 cm-1; EI-MS m/z (rel intensity): 133 (M+, 100).
p-Phenoxybenzonitrile (3f)11: 75% as a colorless liquid; 1H NMR (CDCl3) δ: 6.98-7.08 (m, 4H), 7.20-7.27 (m, 1H), 7.38-7.44 (m, 2H), 7.58-7.62 (m, 2H); 13C NMR (CDCl3) δ: 105.7, 117.8, 118.8, 120.4, 125.1, 130.2, 134.1, 154.7, 161.6; IR (neat): (CN) 2225 cm-1; EI-MS m/z (rel intensity): 195 (M+, 100).
4-Cyanobenzophenone (3g)12: 47% as a white solid; mp 104-105 °C; 1H NMR (CDCl3) δ: 7.52 (t, J = 7.8 Hz, 2H), 7.62-7.68 (m, 1H), 7.78-7.81 (m, 4H), 7.87-7.88 (m, 2H); 13C NMR (CDCl3) δ: 115.6, 118.0, 128.6, 130.0, 130.2, 132.1, 133.3, 136.3, 141.2, 195.0; IR (KBr): (CN) 2227 cm-1, (CO) 1647 cm-1; EI-MS m/z (rel intensity): 207 (M+, 44).
p-Chlorobenzonitrile (3h)2a: 34% as a white solid; mp 87-88 °C; 1H NMR (CDCl3) δ: 7.45-7.49 (m, 2H), 7.59-7.63 (m, 2H); 13C NMR (CDCl3) δ: 110.7, 118.0, 129.7, 133.4, 139.5; IR (neat): (CN) 2225 cm-1; EI-MS m/z (rel intensity): 137 (M+, 100).
m-Tolunitrile (3i)4b: 64% as a colorless liquid; 1H NMR (CDCl3) δ: 2.34 (s, 3H), 7.32-7.50 (m, 4H); 13C NMR (CDCl3) δ: 21.1, 112.2, 119.0, 129.0, 129.3, 132.5, 133.6, 139.2; IR (neat): (CN) 2229 cm-1; EI-MS m/z (rel intensity): 117 (M+, 100).
m-Methoxybenzonitrile (3j)2a: 65% as a colorless liquid; 1H NMR (CDCl3) δ: 3.84 (s, 3H), 7.11-7.17 (m, 2H), 7.22-7.25 (m, 1H), 7.34-7.41 (m, 1H); 13C NMR (CDCl3) δ: 55.5, 113.1, 116.8, 118.7, 119.3, 124.5, 130.3, 159.6; IR (neat): (CN) 2230 cm-1; EI-MS m/z (rel intensity): 133 (M+, 100).
3,5-Dimethylbenzonitrile (3k)7c: 77% as a white solid; mp 42-43 °C; 1H NMR (CDCl3) δ: 2.35 (s, 6H), 7.22 (s, 1H), 7.26 (s, 2H); 13C NMR (CDCl3) δ: 21.0, 112.0, 119.2, 129.6, 134.6, 139.0; IR (KBr): (CN) 2230 cm-1; EI-MS m/z (rel intensity): 131 (M+, 63).
o-Tolunitrile (3l)7c: 64% as a colorless liquid; 1H NMR (CDCl3) δ: 2.56 (s, 3H), 7.23-7.37 (m, 2H), 7.44-7.53 (m, 1H), 7.57-7.64 (m, 1H); 13C NMR (CDCl3) δ: 20.4, 112.7, 118.1, 126.2, 130.2, 132.5, 132.6, 141.9; IR (neat): (CN) 2225 cm-1; EI-MS m/z (rel intensity): 117 (M+, 100).
1-Cyanonaphthalene (3n)7c: 77% as a brown solid; mp 30-31 °C; 1H NMR (CDCl3) δ: 7.50-7.73 (m, 3H), 7.91-7.95 (m, 2H), 8.09 (d, J = 8.4 Hz, 1H), 8.24 (d, J = 8.4 Hz, 1H); 13C NMR (CDCl3) δ: 110.2, 117.8, 124.9, 125.1, 127.5, 128.58, 128.63, 132.3, 132.6, 132.9, 133.3; IR (KBr): (CN) 2218 cm-1; EI-MS m/z (rel intensity): 153 (M+, 100).
References
1. (a) M. Tercel, G. J. Atwell, S. Yang, R. J. Stevenson, K. J. Botting, M. Boyd, E. Smith, R. F. Anderson, W. A. Denny, W. R. Wilson, and F. B. Pruijn, J. Med. Chem., 2009, 52, 7258; CrossRef (b) L. Hu, M. L. Kully, D. W. Boykin, and N. Abood, Bioorg. Med. Chem. Lett., 2009, 19, 3374; CrossRef (c) M. Kohout, J. Svoboda, V. Novotna, D. Pociecha, M. Glogarova, and E. Goreka, J. Mater. Chem., 2009, 19, 3153; CrossRef (d) J. N. Moorthy, A. L. Koner, S. Samanta, A. Roy, and W. M. Nau, Chem. Eur. J., 2009, 15, 4289; CrossRef (e) Y.-B. Men, J. Sun, Z.-T. Huang, and Q.-Y. Zheng, Angew. Chem. Int. Ed., 2009, 48, 2873; CrossRef (f) M. Vilches-Herrera, J. Miranda-Sepulveda, M. Rebolledo-Fuentes, A. Fierro, S. Luehr, P. Iturriaga-Vasquez, B. K. Cassels, and M. Reyes-Parada, Bioorg. Med. Chem., 2009, 17, 2452; CrossRef (g) R. Barattin and A. Gourdon, Eur. J. Org. Chem., 2009, 1022; CrossRef (h) For a review, see: G. P. Ellis and T. M. Romney-Alexander, Chem. Rev., 1987, 87, 779. CrossRef
2. (a) H.-J. Cristau, A. Ouali, J.-F. Spindler, and M. Taillefer, Chem. Eur. J., 2005, 11, 2483; CrossRef (b) C. Yang and J. M. Williams, Org. Lett., 2004, 6, 2837; CrossRef (c) M. Sundermeier, A. Zapf, M. Beller, and J. Sans, Tetrahedron Lett., 2001, 42, 6707. CrossRef
3. (a) J. Zanon, A. Klapars, and S. L. Buchwald, J. Am. Soc. Chem., 2003, 125, 2890; CrossRef (b) R. K. Arvela, N. E. Leadbeater, H. M. Torenius, and H. Tye, Org. Biomol. Chem., 2003, 1, 1119. CrossRef
4. (a) F. G. Buono, R. Chidambaram, R. H. Mueller, and R. E. Waltermire, Org. Lett., 2008, 10, 5325; CrossRef (b) A. Littke, M. Soumeillant, R. F. Kaltenbach, R. J. Cherney, C. M. Tarby, and S. Kiau, Org. Lett., 2007, 9, 1711; CrossRef (c) H. R. Chobanian, B. P. Fors, and L. S. Lin, Tetrahedron Lett., 2006, 47, 3303; CrossRef (d) M. Hatsuta and M. Seki, Tetrahedron, 2005, 61, 9908; CrossRef (e) R. S. Jensen, A. S. Gajare, K. Toyota, M. Yoshifuji, and F. Ozawa, Tetrahedron Lett., 2005, 46, 8645; CrossRef (f) K. M. Marcantonio, L. F. Frey, Y. Liu, Y. Chen, J. Strine, B. Phenix, D. J. Wallace, and C. Y. Chen, Org. Lett., 2004, 6, 3723. CrossRef
5. M. Sundermeier, S. Mutyala, A. Zapf, A. Spannenberg, and M. Beller, J. Organomet. Chem., 2003, 684, 50. CrossRef
6. T. Scharema, A. Zapf, and M. Beller, Chem. Commun., 2004, 1388. CrossRef
7. (a) T. Scharema, R. Jackstell, T. Schulz, A. Zapf, A. Cotté, M. Gotta, and M. Beller, Adv. Synth. Catal., 2009, 351, 643; CrossRef (b) T. Scharema, A. Zapf, W. Mägerlein, N. Müller, and M. Beller, Tetrahedron Lett., 2007, 48, 1087; CrossRef (c) O. Grossman and D. Gelman, Org. Lett., 2006, 8, 1189. CrossRef
8. (a) K. Chattopadhyay, R. Dey, and B. C. Ranu, Tetrahedron Lett., 2009, 50, 3164; CrossRef (b) Y. Ren, Z. Liu, S. He, S. Zhao, J. Wang, R. Niu, and W. Yin, Org. Process Res. Dev., 2009, 13, 764; CrossRef (c) N. S. Nandurkar, and B. M. Bhanage, Tetrahedron, 2008, 64, 3655; CrossRef (d) S. Velmathi and N. E. Leadbeater, Tetrahedron Lett., 2008, 49, 4693; CrossRef (e) G. Chen, J. Weng, Z. Zheng, X. Zhu, Y. Cai, J. Cai, and Y. Wan, Eur. J. Org. Chem., 2008, 3524; CrossRef (f) Y.-Z. Zhu and C. Cai, Eur. J. Org. Chem., 2007, 2401; CrossRef (g) S. A. Weissman, D. Zewge, and C. Chen, J. Org. Chem., 2005, 70, 1508; CrossRef (h) T. Schareina, A. Zapf, and M. Beller, J. Organomet. Chem., 2004, 689, 4576. CrossRef
9. (a) T. Mino, H. Shindo, T. Kaneda, T. Koizumi, Y. Kasashima, M. Sakamoto, and T. Fujita, Tetrahedron Lett., 2009, 50, 5358; CrossRef (b) T. Mino, K. Kajiwara, Y. Shirae, M. Sakamoto, and T. Fujita, Synlett, 2008, 2711; CrossRef (c) T. Mino, Y. Shirae, T. Saito, M. Sakamoto, and T. Fujita, J. Org. Chem., 2006, 71, 9499; CrossRef (d) T. Mino, Y. Shirae, Y. Sasai, M. Sakamoto, and T. Fujita, J. Org. Chem., 2006, 71, 6834; CrossRef (e) T. Mino, Y. Shirae, M. Sakamoto, and T. Fujita, J. Org. Chem., 2005, 70, 2191; CrossRef (f) T. Mino, Y. Shirae, M. Sakamoto, and T. Fujita, Synlett, 2003, 882. CrossRef
10. R. Popielarz and D. R. Arnold, J. Am. Chem. Soc., 1990, 112, 3068. CrossRef
11. Q. Zhang, D. Wang, X. Wang, and K. Ding, J. Org. Chem., 2009, 74, 7187. CrossRef
12. H. Xu, K. Ekoue-Kovi, and C. Wolf, J. Org. Chem., 2008, 73, 7638. CrossRef