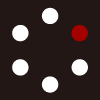
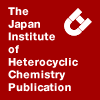
HETEROCYCLES
An International Journal for Reviews and Communications in Heterocyclic ChemistryWeb Edition ISSN: 1881-0942
Published online by The Japan Institute of Heterocyclic Chemistry
e-Journal
Full Text HTML
Received, 6th November, 2010, Accepted, 20th December, 2010, Published online, 14th January, 2011.
DOI: 10.3987/COM-10-12099
■ Investigations into the Nucleophilic meso-Substitution of F-BODIPYs and Improvements to the Synthesis of 4,4-Difluoro-4-bora-3a,4a-diaza-s-indacene
Sarah M. Crawford and Alison Thompson*
Department of Chemistry, Dalhousie University, Halifax, Nova Scotia, B3H 4J3, Canada
Abstract
A series of three F-BODIPYs, with varying levels of steric crowding about the meso-position were selected to investigate nucleophilic meso-substitution of F-BODIPYs. The synthesis of one of these F-BODIPYs, 4,4-difluoro-4-bora-3a,4a-diaza-s-indacene (totally unsubstituted dipyrrin skeleton), was optimized to give higher yields over routine literature procedures. This modified procedure involves oxidation of a dipyrromethane using p-chloranil, instead of DDQ, to give a dipyrrin which is then trapped in situ as its BF2 complex. Nucleophilic meso-alkylation of the series of F-BOIDPYs with n-butyllithium gave meso-butyl F-BODIPYs in moderate to good yields. This work represents a new, synthetically viable method for the synthesis of meso-alkylated F-BODIPYs. Extension of the nucleophilic substitution methodology to meso-arylation was possible. However, the reaction was unselective: substitution at boron, to give the boron-diaryl C-BOIDPYs, occurred preferentially to nucleophilic meso-substitution and thus a mixture of products was obtained.INTRODUCTION
Molecules containing the 4,4-difluoro-4-bora-3a,4a-diaza-s-indacene (F-BODIPY) framework have wide applications as dyes, fluorescent probes in biological systems, and materials for incorporation into electroluminescent devices.1,2 Both symmetrical and unsymmetrical F-BODIPYs are routinely synthesized in high yields from the isolated dipyrrins free-bases or HX salts or by trapping the dipyrrin in situ.3 However, dipyrrin free-bases and salts are historically difficult to manipulate and purify. Furthermore, there are only a few methods available to synthesize meso-substituted dipyrrins.
To produce meso-substituted dipyrrins (Figure 1), one can condense two equivalents of an α-unsubstituted pyrrole with a carboxylic acid,4 an acid chloride5 or an orthoformate6 (method 1) or oxidize a 5-unsubstituted dipyrromethane to the corresponding meso-unsubstituted dipyrrin (method 2).7 The first method is limited to the synthesis of symmetrical dipyrrins, while the second method is currently limited to the synthesis of dipyrrins with meso-aryl substituents and it requires the synthesis of the dipyrromethane starting material. Dipyrrins with meso-alkyl substituents can be synthesized from alkyl acid chlorides (method 1); however, the meso-alkyl dipyrrins are generally unstable and are thus trapped in situ using boron trifluoride diethyletherate and then isolated as their corresponding F-BODIPYs, with overall yields often below 20%.8-11
As shown in Figure 1, another potential strategy to meso-substituted dipyrrins involves substitution of meso-unsubstituted dipyrrins. However, examples of such direct meso-substitution of dipyrrins or dipyrrinato complexes are rare in the literature. meso-Cyano dipyrrins can be directly generated from meso-unsubstituted dipyrrins through cyanide anion attack at the meso-position to give the corresponding dipyrromethane, which can then be oxidized back to the dipyrrin.12 Oligopyrrolic bile pigments,13,14 containing one or more dipyrrin units, are reported to undergo meso-substitution in the presence of ethanethiole. A prodigiosin analogue,15 which contains a dipyrrin unit, was also reported to undergo photo-induced substitution with sulfur-based nucleophiles to give meso-substituted derivatives.
Direct meso-modification of F-BODIPYs has recently received interest and attempts at modification have utilized a meso-thioalkyl F-BODIPY as starting material.16 The meso-thioalkyl substituent was shown to undergo nucleophilic substitution with amines,16,17 and it was also coupled with aryl boronic acids, via the Liebeskind-Srögl cross-coupling reaction,18 to generate other meso-substituted F-BODIPYs. Although this approach represents a great improvement in the synthesis of meso-aryl F-BODIPYs, it is limited to the generation of symmetrical derivatives as the meso-thioalkyl F-BODIPY is generated by the reaction of thiophosgene with two equivalents of a substituted pyrrole to give the corresponding dipyrrolthione, which is then alkylated and trapped as its BF2 complex.16
meso-Unsubstituted porphyrins and their metal complexes are susceptible to nucleophilic substitution at the meso-position, and the resulting intermediate is oxidized in situ to give meso-aryl and meso-alkyl substituted porphyrins.19,20 Aryl and alkyl lithium reagents, with varying levels of functionalization, are the nucleophiles employed in these reactions. Interestingly, a small amount of a meso-butyl substituted BODIPY was isolated from the reaction of a meso-unsubstituted BODIPY with 2 equivalents of a perfluorinated aryl lithium reagent (prepared from reacting the perfluorinated bromobenzene with n-butyl lithium).21 Based on this knowledge, we aimed to develop a methodology for the generation of meso-substituted F-BODIPYs via nucleophilic substitution at the meso-position of meso-unsubstituted F-BODIPYs.
RESULTS AND DISCUSSION
An ideal synthetic route for the synthesis of a meso-substituted F-BODIPY would be to begin with a chemically robust meso-unsubstituted F-BODIPY, which would undergo nucleophilic substitution at the meso-position. Three F-BODIPYs (Figure 2), containing examples with increasing steric crowding flanking the meso-position, were selected as test compounds to investigate a methodology for the nucleophilic meso-modification of meso-unsubstituted F-BOIDPYs to give the corresponding meso-substituted F-BOIDPYs.
F-BODIPYs 2 and 3 were synthesized from the corresponding dipyrrins using traditional methods.22 The synthesis of F-BODIPY 1 has only very recently been reported.23-25 One synthesis involves a four-step procedure from pyrrole, in 35 % overall yield.16,23,26 The other two methods are one-pot reactions and involve trapping the unstable dipyrrin intermediate: both have reported yields under 10 %.24,25 In order for this one-pot method for the preparation of 1 to be synthetically viable as a starting point for preparing derivatized F-BODIPYs, the yields needed to be increased.
The one-pot procedure involving the oxidation of an unsubstituted dipyrrin was selected for optimization.25 The dipyrrin starting material (4) was synthesized using a literature method.27 A series of trials were conducted in order to optimize the reaction conditions, the oxidant used in the dipyrrin formation reaction, and the base used in the F-BODIPY formation reaction. The results of these trials are outlined in Scheme 1.
The bases used in the F-BODIPY formation were explored first (Trial 1 through Trial 3), using DDQ as an oxidant to generate the dipyrrin. When triethylamine was used as a base, none of the desired unsubstituted F-BODIPY (1) was formed; however, when DIPEA or DBU were used, 1 was isolated in very low yield (Trials 2 and 3) in our hands, even though the literature reports indicate yields between 8 and 10% when using DIPEA.24,25 DIPEA was thus selected as the base to use in further trials as it reproducibly gave the best yield (e.g. Trial 2). After the base for the F-BODIPY formation was selected, the oxidant for the dipyrrin formation was investigated. The isolated yield of the F-BODIPY 1 increased substantially when the oxidant was modified from DDQ to the milder p-chloranil (Trials 4-6). Using these modified conditions, the F-BODIPY 1 was generated in an average yield of 21% on a 100 mg scale.
With F-BODIPYs 1, 2 and 3 in hand, investigations proceeded regarding nucleophilic meso-substitution. Solutions of each compound were treated with n-butyllithium at -78 °C. The reaction mixture was slowly warmed to room temperature and then treated with DDQ. The meso-butyl substituted F-BODIPYs 5, 6,28 and 728 were isolated in moderate yields as shown in Scheme 2.
This transformation represents a new method for the synthesis of alkyl substituted F-BODIPYs in better yields than the existing published methods.8-11 In the only previous example of the synthesis of a meso-butylated BODIPY from the meso-unsubstituted analogue, the authors speculated that a perfluorinated aryl lithium reagent (prepared from reacting the perfluorinated bromobenzene with n-butyl lithium) deprotonated the meso-position of the BODIPY, followed by reaction of the resultant monoanionic species with the residual n-butylbromide.21 We postulate,28 based on color changes during the course of the reaction, that the alkylation addition occurs by the nucleophilic attack of the n-butyl anion at the meso-position to give a charged dipyrromethane-type intermediate, as shown in Figure 3.
The decrease in yield of the meso-butyl F-BODIPYs with decreasing substitution about the F-BODIPY is likely due to a decrease in the stability of boron-containing dipyrromethane-type intermediate with decreasing substitution, analogous to that of the corresponding dipyrrin series.29 Isolated yields of the meso-butylated F-BODIPYs 5, 6 and 7 increased with increasing substitution from 26%, for the least substituted, to 61%, for the most substituted. Nevertheless, this strategy provides a reasonable and reliable route to meso-butyl substituted F-BODIPYs.
We also investigated the meso-arylation of the F-BODIPYs 1, 2 and 3. We have previously reported that when the F-BODIPY 3 is treated phenyllithium the meso-phenyl product was not produced; however, the B-diphenyl C-BODIPY 8 was isolated in 22% yield.28 Interestingly, when the reaction with 10 eq phenyllithium was conducted at room temperature, in the absence of DDQ, a mixture of the C-BODIPY 8 and the C-BODIPY 9, with the desired meso-phenyl substituent, were generated as shown in Scheme 3. Presumably the substitution at boron is accompanied/followed by nucleophilic attack and then elimination at the meso-position.
Integration of the corresponding peaks in the 1H NMR spectrum, from the reaction carried out at room temperature, showed BODIPY 8 and BODIPY 9 to be isolated in a 0.2:1.0 ratio. The absence of F-BODIPY material in the reaction mixture indicates that nucleophilic attack at the boron centre appears to occur preferentially to nucleophilic attack at the meso-position in the case of the aryllithium reagent. This reactivity has been exploited to synthesize C-BODIPYs from meso-substituted F-BODIPYs although the products are generally isolated in yields below 50%.30 The absence of DDQ in this reaction also indicates that this transformation may be occurring through an alternate mechanism than the analogous meso-alkylation. Color observations are not useful in this case because the phenyllithium reagent itself is a red color and obscures any loss of color due to the dipyrrinato construct.
When F-BODIPY 1 and F-BODIPY 2 were treated with phenyllithium, mixtures of meso-unsubstituted C-BODIPYs and meso-phenyl C-BODIPYs were isolated in yields of 10% and 6%, respectively (Scheme 4).
The reaction of the F-BODIPY 1 with phenyllithium resulted in two products, which could not be fully characterized. The mass spectrum (ESI+) the mixture indicated the formation of a mixture of C-BODIPY 10 and C-BODIPY 11, as shown in Scheme 4. A comparison of the relative integrations of the pyrrolic hydrogen peaks in the proton NMR spectrum of the product indicated that C-BODIPY 10 and C-BODIPY 11 were present in a 1 to 0.6 ratio. The reaction of F-BODIPY 2 with phenyllithium gave the C-BODIPY 12 and the C-BODIPY 13 in a 1 to 0.7 ratio. Under the same conditions, the meso-arylated C-BODIPY 9 could not be isolated. This indicates that steric factors play a role in meso-arylation, with low temperature meso-arylation being favored when the meso-position is not blocked by nearby substituents; however, B-arylation is favored over meso-arylation in all cases when using phenyllithium.
To conclude, as the interest in and possible applications of BODIPYs grows, methods for the direct modification of F-BODIPYs will be needed. In order to investigate the meso-modification of F-BODIPYs we optimized a reported synthesis24,25 of the totally unsubstituted F-BODIPY such that 1 can now be routinely isolated in 20-30% yield. We have also developed a synthetically viable, alternative method for the production of meso-butylated F-BODIPYs by exploiting the nucleophilic attack of n-butyllithium at the meso-position of F-BODIPYs. Expansion of this methodology to other alkyllithium reagents is currently under investigation. meso-Arylation, using the same method, was not successful as nucleophilic attack at the boron centre was more favorable to nucleophilic attack at the meso-position and mixtures of products were isolated.
EXPERIMENTAL
All 1H NMR (500 MHz), 13C NMR (125 MHz), and 11B NMR (160 MHz) spectra were recorded using a Bruker Avance AV-500 spectrometer.31 Chemical shifts are expressed in parts per million (ppm) using the solvent signal [CDCl3 (1H 7.26 ppm; 13C 71.16 ppm)] as an internal reference for 1H and 13C and BF3•OEt2 as an external reference for 11B. Splitting patterns are indicated as follows: br, broad; s, singlet; d, doublet; t, triplet; q, quartet; m, multiplet. All coupling constants (J) are reported in Hertz (Hz). Mass spectra were obtained using ion trap time-of-flight (ESI) instruments. Column chromatography was performed using 230-400 mesh ultra pure silica or 150 mesh Brockmann III activated, basic aluminum oxide, as indicated. Spectral data for compounds 1,23-25 2,3,22,28,31 3,22,28 4,27 6,28 7, 28 and 828 have been previously reported in the literature.
4-Bora-3a,4a-diaza-s-indacene (1)
Following a modified literature procedure,25 a suspension of 2,3,5,6-tetrachloro-p-benzoquinone (174 mg, 0.71 mmol) in DCM (7.5mL) under a nitrogen atmosphere was added drop-wise to a stirred solution of di(1H-pyrrol-2-yl)methane27 (100 mg, 0.68 mmol) in DCM (7.5 mL) at -80 ˚C under nitrogen. Once the addition was complete, the solution was stirred at -80 ˚C for 1 h. Diisopropylethylamine (0.71 mL, 4.08 mmol) was added drop-wise to the reaction mixture followed by BF3•OEt2 (0.68 mL, 6.12 mmol) and the reaction mixture was stirred for 3 h while warming to -30 oC. The reaction mixture was filtered through celite and the solvent was removed in vacuo. The crude solid was purified over silica gel eluting with 50% DCM in hexanes. The combined fractions were concentrated in vacuo and then purified over silica gel eluting with 15% ethyl acetate in hexanes and the combined fractions were then concentrated in vacuo to give 1 as a red solid (30 mg, 23%). δH (500 MHz, CDCl3) 7.90 (s, 2H), 7.42 (s, 1H), 7.16 (d, J = 4.0, 2H), 6.55 (d, J = 4.5, 2H); δC (125 MHz, CDCl3) 145.2, 135.0, 131.5 (q, J = 2), 131.4, 118.9 (q, J = 2); δB (160 MHz, CDCl3) 0.15 (t, J = 30); m/z ESI+ found 215.0564 [M+Na]+ calculated for C9H7BF2N2Na 215.0568. 13C NMR data matches that previously reported.23-25
8-Butyl-4-bora-3a,4a-diaza-s-indacene (5)
n-Butyllithium (1.9 mL of a 1.6 M solution in hexanes, 3.1 mmol) was slowly added under a nitrogen atmosphere to a round-bottom flask containing a solution of 1 (60 mg, 0.31 mmol) in THF (10 mL) at -78 ˚C. The solution was stirred while slowly warming to -30 ˚C. At -30 ˚C, methanol (2 mL) was added drop-wise followed by a 0.1 M aqueous solution of HCl (2 mL) and the mixture was stirred for 10 min. The mixture was removed from the cooling bath and 2,3-dichloro-5,6-dicyano-p-benzoquinone (541 mg, 2.4 mmol) was added and the reaction mixture was stirred for 3 h at room temperature. The reaction mixture was diluted with DCM (75 mL) and water (150 mL). The layers were separated and the organic layer was washed with water (3 x 150 mL). The organic layer was dried over anhydrous Na2SO4 and the solvent was removed in vacuo. The crude solid was dissolved in DCM and the solution was filtered through a pad of silica eluting with DCM. Purification over silica gel eluting with 50% DCM in hexanes gave 5 as an orange solid (20 mg, 26%). δH (500 MHz, CDCl3) 7.85 (s, 2H), 7.27-7.28 (m, 2H), 6.53-6.54 (m, 2H), 2.95-2.92 (m, 2H), 1.81-1.75 (m, 2H), 1.47 (sextet, J = 7.5, 2H), 0.97 (t, J = 7.5, 3H); δC (125 MHz, CDCl3) 151.4, 143.4, 135.3, 127.9, 118.1, 36.1, 31.3, 23.3, 13.9; δB (160 MHz, CDCl3) 0.02 (t, J = 30); m/z ESI+ found 271.1178 [M+Na]+ calculated for C13H15BF2N2Na 271.1194.
4,4-Diphenyl-1,3,5,7-tetramethyl-2,6-diethyl-8-H-4-bora-3a,4a-diaza-s-indacene (8) and 4,4- diphenyl-1,3,5,7-tetramethyl-2,6-diethyl-8-phenyl-4-bora-3a,4a-diaza-s-indacene (9)
Phenyllithium (4.6 mL of a 1.8 M solution in di-n-butyl ether, 8.2 mmol) was slowly added under a nitrogen atmosphere to a round-bottom flask containing a solution of 3 (250 mg, 0.82 mmol) in diethyl ether (15 mL) at 25 ˚C. The solution was allowed to stir at room temperature for 18 h. Methanol (5 mL) was added drop-wise and the reaction mixture was concentrated in vacuo to give an orange oil. The crude oil was purified over silica gel eluting with 4% EtOAc in hexanes to give a mixture of 8 and 9 as an orange solid (28 mg 8, 7%, and 142 mg 9, 29%). δH (500 MHz, CDCl3) 7.49-7.47 (m, 3H, 9), 7.42-7.40 (m, 4H, 9), 7.36-7.34 (m, 2H, 9), 7.30-7.16 (m, 8.5H, 8 and 9), 7.13 (s, 0.20H, 8), 2.33 (q, J = 7.0, 0.84H, 8), 2.22 (m, J = 7, 5H, 8 and 9), 1.77-1.76 (m, 6.7H, 8 and 9), 1.31 (s, 6H, 9), 0.99 (t, J = 7, 1.3H, 8), 0.90 (t, J = 7, 6H, 9); δC (125 MHz, CDCl3) 154.0, 153.1, 140.8, 137.1, 135.4, 134.1, 133.9, 133.8, 132.9, 131.6, 130.9, 129.0, 128.9, 128.5, 127.28, 127.26, 125.7, 125.6, 119.5, 19.5, 17.7, 17.5, 14.9, 14.7, 14.5, 12.1, 9.5; δB (160 MHz, CDCl3) -0.34 (broad s); m/z ESI+ [M+Na]+ 443.3, 519.3. Although this mixture could not be separated, assignments are based on those of a pure sample of the C-BODIPY 8.28
4,4-Diphenyl-8H-4-bora-3a,4a-diaza-s-indacene (10) and 4,4-diphenyl-8-phenyl-4-bora-3a,4a- diaza-s-indacene (11)
Phenyllithium (0.65 mL of a 1.8 M solution in di-n-butyl ether, 1.2 mmol) was slowly added under a nitrogen atmosphere to a round-bottom flask containing a solution of 1 (56 mg, 0.29 mmol) in THF (11 mL) at -45 ˚C. The solution was stirred for 30 min and then the cooling bath was removed and the mixture was stirred at room temperature for 1 h. A 0.1 M aqueous solution of HCl (4 mL) was added drop-wise and the reaction mixture was stirred for 5 min. 2,3-Dichloro-5,6-dicyano-p-benzoquinone (658 mg, 10 mmol) was added and the reaction mixture was stirred for 1 h at room temperature. The reaction mixture was filtered through a pad of Brockman III neutral alumina, dried over Na2SO4, and concentrated in vacuo to give a brown oil. The crude oil was filtered through silica gel eluting with 15% EtOAc in hexanes and the combined fractions were concentrated in vacuo to give an orange solid. Purification over silica using a gradient of 1% EtOAc in hexanes to 3% EtOAc in hexanes, and concentration in vacuo gave a mixture of 10 (3.4 mg, 4 %) and 11 (2.1 mg, 2%) as an orange solid. δH (500 MHz, CDCl3) 7.55-7.54 (m, 1H), 7.52-7.51 (m, 0.5H), 7.50-7.49 (m, 0.5H), 7.40-7.39 (m, 0.5H), 7.31 (d, J = 4, 0.50H), 7.25-7.17 (m, 4H), 7.13-7.11 (m, 2H), 7.07-7.01 (m, 6H), 6.91-6.87 (m, 2H), 6.59 (d, J = 4.5, 0.5H), 6.53 (d of d, J = 2.0, 4.0, 1H), 6.40 (d of d, J = 1.5, 4.0, 0.5H); δC (125 MHz, CDCl3) 145.9, 144.5, 136.9, 135.0, 132.8, 132.4, 131.4, 130.6, 129.8, 129.3, 129.2, 128.4, 128.1, 127.6, 127.2, 127.1, 126.4, 126.0, 125.3, 121.2, 117.92, 117.86; δB (160 MHz, CDCl3) 0.80 (broad s); m/z ESI+ found 331.1375 [M+Na]+ calculated for C21H17BN2Na 331.1382 (10) and found 407.1675 [M+Na]+ calculated for C27H21BN2Na 407.1695 (11).
4,4-Diphenyl-1,3-dimethyl-2-ethyl-8H-4-bora-3a,4a-diaza-s-indacene (12) and 4,4-diphenyl-1,3- dimethyl-2-ethyl-8-phenyl-4-bora-3a,4a-diaza-s-indacene (13)
Phenyllithium (2.2 mL of a 1.8 M solution in di-n-butyl ether, 4.0 mmol) was slowly added under a nitrogen atmosphere to a round-bottom flask containing a solution of 2 (100 mg, 0.40 mmol) in THF (15 mL) at -45 ˚C. The solution was stirred for 30 min and then the cooling bath was removed and the mixture was stirred at room temperature for 1 h. A 0.1 M aqueous solution of HCl (5 mL) was added drop-wise and the reaction mixture was stirred for 5 min. 2,3-Dichloro-5,6-dicyano-p-benzoquinone (908 mg, 10 mmol) was added and the reaction mixture was stirred for 1 h at room temperature. The reaction mixture was filtered through a pad of Brockmann III neutral alumina, dried over Na2SO4, and concentrated in vacuo to give a brown oil. The crude oil was purified over silica gel eluting with 2% EtOAc in hexanes to give a mixture of 12 and 13 as a bright orange solid. The mixture was purified over silica gel eluting with a gradient of hexanes to 2% EtOAc in hexanes. Concentration in vacuo gave a mixture of 12 and 13 as a bright orange solid (14 mg 12, 10% and 10 mg 13, 6%). The crude mixture of 12 and 13 was again purified over silica eluting with a gradient of hexanes to 2% EtOAc in hexanes. Concentration of the pure fractions in vacuo gave 12 as an orange solid and 13 as an orange solid.
4,4-Diphenyl-1,3-dimethyl-2-ethyl-8 H-4-bora-3a,4a-diaza-s-indacene (12)
δH (500 MHz, CDCl3) 7.29 (s, 1H), 7.23-7.14 (m, 11H), 6.89 (d of d, J = 1.0, 4.0, 1H), 6.31 (d of d, J = 1.0, 4.0, 1H), 2.41 (q, J = 7.5, 2H), 2.26 (s, 3H), 1.84 (s, 3H), 1.06 (t, J = 7.5, 3H); δC (125 MHz, CDCl3) 139.6, 138.0, 136.1, 134.2, 133.3, 132.0, 127.4, 126.0, 124.3, 123.6, 114.9, 105.6, 17.7, 15.0, 14.7, 9.6 (1 C missing); δB (160 MHz, CDCl3) 0.19 (broad s); m/z ESI+ found 387.1985 [M+Na]+ calculated for C25H25BN2Na 387.2003.
4,4-Diphenyl-1,3-dimethyl-2-ethyl-8-phenyl-4-bora-3a,4a-diaza-s-indacene (13)
δH (500 MHz, CDCl3) 7.48-7.46 (m, 3H), 7.39-7.37 (m, 2H), 7.28-7.23 (m, 8H, overlaps with CHCl3 solvent signal), 7.21-7.17 (m, 3H), 6.36 (d of d, J = 1.0, 4.0, 1H), 6.24 (d of d, J = 1.0, 4.0, 1H), 2.34 (q, J = 7.5, 2H), 1.85 (s, 3H), 1.50 (s, 3H), 1.00 (t, J = 7.5, 3H); δC (125 MHz, CDCl3) 160.3, 142.4, 139.2, 139.0, 135.6, 135.4, 134.2, 133.5, 133.3, 129.2, 128.9, 128.3, 127.4, 125.9, 124.1, 114.6, 17.6, 15.3, 14.7, 12.7 (1 C missing); δB (160 MHz, CDCl3) - 0.09 (broad s); m/z ESI+ found 463.2288 [M+Na]+ calculated for C31H29BN2Na 463.2316.
ACKNOWLEDGEMENTS
This work was supported by the Natural Sciences and Engineering Research Council (NSERC) of Canada.
References
1. A. Loudet and K. Burgess, Chem. Rev., 2007, 107, 4891. CrossRef
2. G. Ulrich, R. Ziessel, and A. Harriman, Angew. Chem. Int. Ed., 2008, 47, 1184. CrossRef
3. L. Wu and K. Burgess, Chem. Comm., 2008, 4933. CrossRef
4. B. Tu, C. Wang, and J. Ma, Organic Preparations and Procedures International: The New Journal for Organic Synthesis, 1999, 31, 349.
5. A. Treibs, M. Strell, I. Strell, and D. Grimm, Liebigs Ann. Chem., 1978, 289.
6. C. B. Reese and H. Yan, Tetrahedron Letters, 2001, 42, 5545. CrossRef
7. C. Bruckner, V. Karunaratne, S. J. Rettig, and D. Dolphin, Can. J. Chem., 1996, 74, 2182. CrossRef
8. F. Amat-Guerri, M. Liras, M. L. Carrascoso and R. Sastre, Photochem. Photobiol., 2003, 77, 577. CrossRef
9. Z. Li, E. Mintzer, and R. Bittman, J. Org. Chem., 2006, 71, 1718. CrossRef
10. C. Peters, A. Billich, M. Ghobrial, K. Hogenauer, T. Ullrich, and P. Nussbaumer, J. Org. Chem., 2007, 72, 1842. CrossRef
11. M. Verdoes, U. Hillaert, B. I. Florea, M. Sae-Heng, M. D. P. Risseeuw, D. V. Filippov, G. A. v. d. Marel, and H. S. Overkleeft, Bioorg. Med. Chem. Lett., 2007, 17, 6169. CrossRef
12. G. Sathyamoorthi, J. H. Boyer, T. H. Allik, and S. Chandra, Heteroat. Chem., 1994, 5, 403. CrossRef
13. H. Falk and H. Flîdl, Monats. Chem., 1986, 117, 57. CrossRef
14. M. Holzl, C. Klampfl, and K. Grubmayr, Monats. Chem., 2005, 136, 755. CrossRef
15. J. T. Tomlinson, G. Park, J. A. Misenheimer, G. L. Kucera, K. Hesp, and R. A. Manderville, Org. Lett., 2006, 8, 4951. CrossRef
16. T. V. Goud, A. Tutar, and J.-F. Biellmann, Tetrahedron, 2006, 62, 5084. CrossRef
17. C. F. A. Gomez-Duran, I. Garcia-Moreno, A. Costela, V. Martin, R. Sastre, J. Banuelos, F. L. Arbeloa, I. L. Arbeloa, and E. Pena-Cabrera, Chem. Comm., 2010, 5103. CrossRef
18. E. Peña-Cabrera, A. Aguilar-Aguilar, M. González-Domínguez, E. Lager, R. Zamudio-Vázquez, J. Godoy-Vargas, and F. Villanueva-García, Org. Lett., 2007, 9, 3985. CrossRef
19. X. Feng and M. O. Senge, J. Chem. Soc. Perkin Trans. 1, 2001, 1030. CrossRef
20. M. O. Senge, Acc. Chem. Res., 2005, 38, 733. CrossRef
21. C. Bonnier, W. E. Piers, A. A.-S. Ali, A. Thompson, and M. Parvez, Organometallics, 2009, 28, 4845. CrossRef
22. E. Vos de Wael, J. A. Pardoen, J. A. van Koeveringe, and J. Lugtenburg, Recl. Trav. Chim. Pays-Bas, 1977, 96, 306. CrossRef
23. I. J. Arroyo, R. Hu, G. Merino, B. Z. Tang, and E. Peña-Cabrera, J. Org. Chem., 2009, 74, 5719. CrossRef
24. A. Schmitt, B. Hinkeldey, M. Wild, and G. Jung, J. Fluoresc., 2009, 19, 755. CrossRef
25. K. Tram, H. Yan, H. A. Jenkins, S. Vassiliev and D. Bruce, Dyes and Pigments, 2009, 82, 392. CrossRef
26. P. S. Clezy and G. A. Smythe, Aust. J. Chem., 1969, 22, 239. CrossRef
27. Q. M. Wang and D. W. Bruce, Synlett, 1995, 12, 1267. CrossRef
28. S. M. Crawford and A. Thompson, Org. Lett., 2010, 12, 1424. CrossRef
29. T. E. Wood and A. Thompson, Chem. Rev., 2007, 107, 1831. CrossRef
30. G. Ulrich, C. Goze, S. Goeb, P. Retailleau, and R. Ziessel, New J. Chem., 2006, 30, 982. CrossRef
31. T. E. Wood, B. Berno, C. S. Beshara, and A. Thompson, J. Org. Chem., 2006, 71, 2964. CrossRef