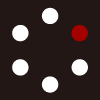
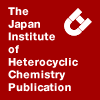
HETEROCYCLES
An International Journal for Reviews and Communications in Heterocyclic ChemistryWeb Edition ISSN: 1881-0942
Published online by The Japan Institute of Heterocyclic Chemistry
e-Journal
Full Text HTML
Received, 8th November, 2010, Accepted, 22nd November, 2010, Published online, 30th November, 2010.
DOI: 10.3987/COM-10-12100
■ A Simple and Efficient Method for Synthesis of Benzothiazepine Derivatives
Saori Itabashi, Rong Lu, and Tetsuo Miyakoshi*
Department of Applied Chemistry, School of Science and Technology, Meiji University, 1-1-1 Higashi-mita, Tama-ku, Kawasaki-shi, 214-8571, Japan
Abstract
A series of 1,5-benzothiazepines were synthesized using disulfides and α,β-unsaturated carbonyl or nitrile compounds as reaction substrates. After reductive cleavage of the S-S bond of disulfides, the resulting thiols were reacted with α,β-unsaturated carbonyl or nitrile compounds to generated seven-membered heterocyclic compounds. In the presence of ammonium thioglycolate, the Michael reaction occurred between disulfides (1) and 4-methyl-3-penten-2-one to give 2,2,4-trymethyl-3H-1,5-benzothiazepine derivatives in good yields. When ethyl acrylate or acrylonitrile was used as the Michael acceptor, 90-99% of (2-amino-phenylsulfanyl)propionitriles (3) and/or 92-99% of (2-amino-phenylsulfanyl)propionic acid ethyl esters (4) were produced. Subsequently, the 1,5-benzothiazepine compounds 5 and 6 were obtained due to the cyclization reaction.1,5-Benzothiazepines are heterocyclic compounds that have widespread physiological and pharmacological activities, such as anti-coagulants,1-3 elastase inhibitors,4 ACE inhibitors,5,6 and antiarrythmic7 activities, and are often used as sedatives and for treatment of cardiovascular disease.8 Because most of the 1,5-benzothiazepine compounds have high efficacy and low toxicity, this ring system has received much attention from many chemists and pharmacologists over the past decade.9-11 It has been extensively studied by a number of research groups, who have reported that the reaction between α,β-unsaturated carbonyl compounds and 2-aminobenzenethiols occurs in two steps involving the formation of the Michael adduct as an intermediate that readily undergoes dehydrative cyclization to give 1,5-benzothiazole.12-18 These methods use thiol, which have a peculiar odor and easily form disulfides in the reaction and most of these methods are impractical due to the low reaction efficiency and environmental toxicity.19-20 Although there are many reports of the synthesis of benzothiazole derivatives, no seven-membered heterocylic compounds were obtained.21-24 In addition, Katritzky reported synthesis of a 2,4-diary-2,3-dihydro-1,5-benzothiazepines,25 but it required at least 4 steps and became a seven-membered heterocylic compound with a closed ring via only when the methyl or methoxy groups were attached at the 6-position of the benzene ring.
In this study, we developed of a new method to synthesize 1,5-benzothiazepines in one-pot based on reductive cleavage of the S-S bond of disulfides using ammonium thioglycolate (ATG) as a catalyst, and the subsequent Michael reaction with the addition of α,β-unsaturated ketone. The reaction yields, mechanisms, and structures of the products are described.
The disulfides used in this study as the starting materials were synthesized from 2-aminobenzothiazoles, because these disulfides are not commercially available. The reaction process is described in the experimental section. After purification by column chromatography on silica gel, the pure compound 1 was obtained as shown in Scheme 1.
After the pure disulfides were obtained, the Michael addition reaction was carried out, as shown in Scheme 2. The compounds 2, 3, and 4 were obtained in good yields after purification by column chromatography on silica gel.
The results for compound 2 are summarized in Table 1. It can be observed from Table 1 that when the disulfide has an electron withdrawing group, only about 30% yield was obtained even after a prolonged reaction of 360 minutes. However, when triethylamine was used instead of ammonium hydroxide in the presence ammonium thioglycolate, and the concentration of the Michael acceptor was up to 5 equivalents, over 60% yields were obtained in the reaction time of 30 min (Entries 6-9).
Compound 5 was synthesized from compound 3 by a Pinner condensation reaction. The results are summarized in Table 2.
As can be seen in Table 2 (entries 3, 4, and 5), the conversion yields seem to be a bit low based on the amount of starting sulfides consumed, although the reason is not clear. However, 1H-NMR analyses showed that the reaction proceeded with reasonably good yields (in parentheses).
Compound 6 was synthesized from compound 4 by an intramolecular condensation reaction using p-toluenesulfonic acid (p-TSA) as a catalyst. The results are summarized in Table 3.
It can be observed from Table 3 that the target products were obtained in high yields regardless of the substituent in the benzene ring.
From the above results, it can be concluded that we successfully synthesized three kinds of 1,5-benzothiazepine derivatives using a stable and odorless disulfide as a raw materials in this study. In the presence of ATG, compound 2 was obtained in a one-step reaction between disulfide and 4-methyl-3-penten-2-one in one-pot. On the other hand, compounds 5 and 6 were obtained in a two-step reaction via the intermediates of compounds 3 and 4, respectively. Because the reaction conditions are mild and easy to control, this reaction is a simple and efficient method for synthesis of seven-membered heterocylic compounds.
EXPERIMENTAL
The detailed progress synthesis of compound 1 is as follows: 9.31 mmol 2-aminobenzothiazole was added to an aqueous solution of 1680 mmol potassium hydroxide and 15 mL ethylene glycol, and then was stirred and refluxed for several hours at 140˚C. After completion of the reaction which was monitored by thin layer chromatography (TLC), the solution was diluted with water and neutralized with acetic acid. When the solution temperature had dropped to room temperature, CHCl3 was added to the solution, and it was stirred at room temperature for 30 min and separated. The CHCl3 layer was washed with brine 3 times and dried with anhydrous magnesium sulfate. The crude product was purified by column chromatography on silica gel, and the pure compound 1 was obtained.
After the pure disulfides were obtained, the Michael addition reaction was carried out. The process is as follows: 0.403 mmol compound 1 was dissolved in dry DMF and heated to 50˚C with nitrogen protection. After stirring for 10 min, the pH was adjusted to 11 with ammonia, then the appropriate ATG and 3 equivalents of the Michael acceptor were added to the solution, and it was continuously stirred until compound 1 was completely consumed, as monitored by TLC. After the reaction was finished, Et2O was added to the solution, and it was separated. The Et2O layer was washed with brine 3 times and dried with anhydrous magnesium sulfate. The crude product was purified by column chromatography on silica gel, and purified compounds 2, 3, and 4 were obtained.
Compound 5 was synthesized from compound 3 by a Pinner condensation reaction. Compound 3 (0.561 mmol) was dissolved in 5 mL 5 wt% HCl-EtOH solution, and then was stirred and refluxed for 3 h. After the reaction was finished, as monitored by TLC, the reaction solution was evaporated to remove EtOH. NaHCO3 was added to neutralize the solution and it was extracted with EtOAc 3 times, to yield the crude compound 5. The crude product was purified by column chromatography on silica gel.
Compound 6 was synthesized from compound 4 by an intramolecular condensation reaction using p-toluenesulfonic acid (p-TSA) as a catalyst. Compound 4 (0.444 mmol) was dissolved in 5 mL o-xylene, 0.2 equivalent of p-TSA was added, and then it was stirred and refluxed at 125˚C overnight. After the reaction which was monitored by TLC, was finished, NaHCO3 was added to neutralize the solution, and it was extracted with CHCl3 3 times, to yield the crude compounds 6. The crude product was purified by column chromatography on silica gel.
The structures of all products of compounds 2, 5, and 6 were analyzed by IR, NMR, and single-crystal X-ray structure analysis (SMART APEX2 Ultra, Bruker). The detailed data of the typical compounds 2a, 5a, and 6a are shown below. The results agree with the published literature.26-28
3H-1,5-Benzothiazepine-2,2,4-trimethyl (Compound 2a)
Yield: 86%. Rf = 0.25 (eluent: n-hexane-EtOAc = 3:1, v/v). Mp 50-51˚C. IR (KBr, cm-1): 2962.13, 1633.41, 1455.99, 1365.84, 767.53, 740.05. 1H-NMR (500 MHz CDCl3): δ = 1.48 (s, 6H, H-10, H-11), 2.23 (s, 2H, H-3), 2.39 (s, 3H, H-12), 7.04 (t, J = 6.8 Hz, 1H, H-8), 7.10(d, J= 6.5 Hz, 1H, H-6), 7.36 (t, J = 7.5 Hz, 1H, H-7), 7.50 (d, J= 6.0 Hz, 1H, H-9). 13C-NMR (500 MHz CDCl3): δ = 29.5 (C-12), 30.8 (C-10, C-11), 47.6 (C-3), 61.1 (C-2), 124.1 (C-6), 124.405 (C-1a), 124.415 (C-11), 129.4 (C-7), 134.9 (C-9), 173.6 (C-4).
2,3H-1,5-Benzothiazepine-4-amine (Compound 5a)
Yield: 80%. Rf = 0.125 (eluent: EtOAc-EtOH = 7:1, v/v). Mp 118-119˚C. IR (KBr, cm-1): 34593.67, 1656.07, 1453.58, 1033.18, and 763.191 cm-1. 1H-NMR (500 MHz DMSO-d6): δ = 2.41 (t, J=6.9 Hz, 2H, H-3), 3.47 (t, J =6.9 Hz, 2H, H-2), 6.87 (m, 2H, H-6, H-8), 7.25 (m, 1H, H-7), 7.39 (dt, J =1.7, 8.0 Hz, 1H, H-8), 7.03 (br, 2H, NH2). 13C-NMR (500 MHz CDCl3): δ = 29.6 (C-3), 38.6 (C-2), 122.1, 124.5 (C-6, 8), 123.8 (C-1a), 129.4 (C-7), 134.3 (C-9), 152.4 (C-5a), 164.2 (C-9).
2,3-H-1,5-Benzothiazepine-4 (5H)-one (Compound 6a)
Yield: 82%. Rf = 0.25 (eluent: n-hexane-EtOAc = 1:1, v/v). Mp 193-194˚C. IR (KBr, cm-1): 1588.57, 1491.67, 1035.59, 791.15, 755.00, 460.42. 1H-NMR (500 MHz CDCl3): δ = 2.64 (t, 2H, H-3), 3.45 (t, J =7.5 Hz, 2H,H-2), 7.11 (d, J =5.0 Hz, 1H, H-6), 7.14 (t, J = 7.5 Hz, 1H, H-8), 7.36 (t, J =5.0 Hz, 1H, H-7), 7.61 (d, J=10.0 Hz, 1H, H-9), 8.02 (br, 1H, H-5 (NH)). 13C-NMR (500 MHz CDCl3): δ = 33.5 (C-3), 34.2 (C-2), 123.3 (C-6), 126.5 (C-8), 127.0 (C-1a), 129.8 (C-7), 135.5 (C-9), 141.4 (C-5a), 173.6 (C-4).
ACKNOWLEDGEMENTS
This work was partly supported financially by the Research Project Grant A from the Institute of Science and Technology, Meiji University.
References
1. M. Bakthadoss and G. Murugan, Synth. Commun., 2008, 38, 3406. CrossRef
2. K. S. Atwal, S. Z Ahmed, D. M. Floyd, S. Moreland, and A. Hedberg, Med. Chem. Lett., 1993, 13, 2797. CrossRef
3. T. Suzuki, H. Kurosawa, K. Naito, M. Otsuka, M. Ohashi, and O. Takaiti, Eur. J. Pharm., 1991, 194, 195. CrossRef
4. J. W. Skiles, R. Sorcek, S. Jacober, C. Miao, P. W. Mui, D. McNeil, and A. S. Rosenthal, Bioorg. Med. Chem. Lett., 1993, 3, 77. CrossRef
5. J. Slade, J. L. Stanton, D. Ben-David, and G. C. Mazzenga, J. Med. Chem., 1985, 28, 1517. CrossRef
6. F. Micheli, F. Degiorgis, A. Feriani, A. Patio, A. Pozzan, P. Zarantonello, and S. Pierfausto, J. Comb. Chem., 2001, 3, 224. CrossRef
7. K. Yamada, T. Shimamura, and H. Nakajima, Jpn. J. Pharmacol, 1973, 23, 321. CrossRef
8. J. B. Bariwal, K. D. Upadhyay, A. T. Manveer, J. C. Trivedi, J. S. Singh, K. S. Jain, and A. K. Shah, Eur. J. Med, Chem., 2008, 43, 2279. CrossRef
9. G. Grandolini, L. Perioli, and V. Ambrogi, Eur. J. Med. Chem., 1999, 34, 701. CrossRef
10. R. Di Santo and R. Costi, Il Farmaco, 2005, 60, 385. CrossRef
11. Y. Sugawara and S. Nakamura, J. Pharm. Biomed. Anal., 1994, 12, 1397. CrossRef
12. G. Sharma, R. Kumar, and A. K. Chakraborti, Tetrahedron Lett., 2008, 49, 4269. CrossRef
13. K. Arya and A. Danadia, Bioorg. Med. Chem. Lett., 2008, 18, 114. CrossRef
14. W. D. Stephens and L. Field, J. Org. Chem., 1959, 24, 1576. CrossRef
15. A. Solankee, K. Kapadia, P. Solankee, Y. Prajapati, H. Patel, and S. College, Indian J. Chem., 2008, 47B, 473.
16. X. Yang, L. Buzon, E. Hamanaka, and K. Liu, Tetrahedron, 2000, 11, 4447. CrossRef
17. C. L. Lee, Y. Lam, and S.-Y. Lee, Tetrahedron Lett., 2001, 42, 109. CrossRef
18. S. V. Karthikeyan and S. Perumal, Tetrahedron, 2007, 48, 2261. CrossRef
19. N. Karodia, X. Liu, P. Ludley, D. Pletsas, and G. Stevenson, Tetrahedron, 2006, 62, 11039. CrossRef
20. A. Kamimura, H. Mitsudera, Y. Omata, K. Matsuura, M. Shirai, and A. Kakehi, Tetrahedron, 2002, 58, 9817. CrossRef
21. I. Hutchinson, M. S. Chua, H. L. Browne, V. Trapani, T. D. Bradshaw, A. D. Westwell, and M. F. G. Stevens, J. Med. Chem., 2001, 44, 1446. CrossRef
22. M. Wang, M. Z. Gao, B. H. Mock, K. D. Miller, G. W. Sledge, G. D. Hutchins, and Q. H. Zheng, Bioorg. Med. Chem., 2006, 14, 8599. CrossRef
23. C. Wu, J. Wei, K. Gao, and Y. Wang, Bioorg. Med. Chem., 2007, 15, 2789. CrossRef
24. S. Aiello, G. Wells, E. L. Stone, H. Kadri, R. Bazzi, D. R. Bell, M. F. G. Stevens, C. S. Matthews, T. D. Bradshaw, and A. D. Westwell, J. Med. Chem., 2008, 51, 5135. CrossRef
25. A. R. Katritzky, B. V. Rogovoy, C. Chassaing, V. Vvedensky, B. Forood, B. Flatt, and H. Nakai, J. Heterocycl. Chem., 2000, 37, 1655. CrossRef
26. T. Erker, Liebigs Ann., 1989, 6, 601.
27. J. S. Walia, A. S. Walia, A. C. Lankin, R. C. Peterson, and J. Singh, J. Heterocycl. Chem., 1985, 22, 111. CrossRef
28. K. Rutkauskas and Z. I. Beresnevicius, Chem. Heterocycl. Comp., 2006, 42, 227. CrossRef