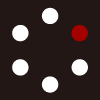
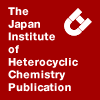
HETEROCYCLES
An International Journal for Reviews and Communications in Heterocyclic ChemistryWeb Edition ISSN: 1881-0942
Published online by The Japan Institute of Heterocyclic Chemistry
e-Journal
Full Text HTML
Received, 10th November, 2010, Accepted, 12th January, 2011, Published online, 13th January, 2011.
DOI: 10.3987/COM-10-S(P)1
■ Deuterium-Labeled Benzyladenine: Synthesis and Application as a Surrogate
Nkaelang Modutlwa, Hiroyuki Tada, Yoshiki Sugahara, Koichi Shiraki, Nobuyuki Hara, Yoshihiro Deyashiki, Tomohiro Maegawa, Yasunari Monguchi,* and Hironao Sajiki*
Laboratory of Medicinal Chemistry, Gifu Pharmaceutical University, 1-25-4 Daigakunishi, Gifu 501-1196, Japan
Abstract
N6-Benzyladenine (benzyladenine), a plant growth regulator, was efficiently deuterated by the hydrogen–deuterium (H–D) exchange reaction catalyzed by palladium on carbon-ethylenediamine complex [Pd/C(en)], while use of palladium on carbon (Pd/C) as a catalyst led to a low deuterium incorporation at room temperature or complete removal of the N6-benzyl group at 110 °C or higher temperature. The obtained benzyladenine-d5 was used as an internal standard (surrogate) for the quantification of residual benzyladenine in fruits, vegetables, cereals, and beans using LC/MS/MS. Satisfactory recovery of benzyladenine between 94.2 and 105.7% (100.4% on the average) was obtained. The agrochemical could be detected within the concentration range of 0.25–0.50 ng/g in agricultural products using the present quantification method.INTRODUCTION
N6-Benzyladenine (benzyladenine) has been used as a plant growth promoter of vegetables, cereals, and fruits, since it was found to be a synthetic cytokinin1 that activates cell division in plants, leading to the promotion of leaf growth,2,3 inhibition of leaf senescence and preservation of chlorophyll.4,5 Although the standard for the residual quantity of benzyladenine in agricultural products was established by Japan's Food Sanitation Law, the quantification method requires a cumbersome sample preparation and is often difficult to reach a high measurement sensitivity.
Recently, deuterium-labeled compounds have been employed as the internal standards, surrogates, for the quantitative analysis of pesticides in crops by gas or liquid chromatography (GC or LC) combined with mass spectrometry (MS).6–8 Although the 3H-, 13C-, and 15N-labeled benzyladenine derivatives9–14 were prepared, their use as a surrogate has not been well-studied, and the synthesis of deuterated benzyladenine has not been reported.
Existing methods for the preparation of deuterium labeled compounds are categorized into the following two types: multi-step synthetic methods starting from small deuterium-labeled precursors,15–17 and the post-synthetic hydrogen–deuterium (H–D) exchange of the unlabeled compounds.18–23 The latter methods are much more useful in terms of time and cost efficiency, since deuterium atoms could be introduced into the existing compounds. We have recently reported an efficient and chemoselective H–D exchange reaction catalyzed by activated carbon-supported transition-metal catalysts in the presence of deuterium oxide (D2O) and hydrogen gas (Figure 1). When palladium on carbon (Pd/C) was used as the catalyst, hydrogen atoms on the benzylic carbon of alkylbenzenes was effectively replaced with deuterium atoms at 50 °C.24,25 Raising the temperature to 110–160 °C could extend the deuterated portions to all the hydrogen atoms on the alkyl chains,26,27 and heteroaromatic nuclei including the base moieties of the nucleosides were also deuterated.28,29 The use of platinum on carbon (Pt/C) instead of Pd/C preferentially promoted the H–D exchange reaction on aromatic rings,30,31 while rhodium on carbon (Rh/C) catalyzed the deuteration of the non-activated alkanes bearing no functionalities.32 We have also found that ruthenium on carbon (Ru/C) efficiently catalyzed the chemoselective H–D exchange reaction on the carbon atoms adjacent to the hydroxyl groups of primary and secondary alcohols.33
The benzylamino group is quite labile under the hydrogenation conditions catalyzed by transition metals.34 The deuteration of benzyladenine by such H–D exchange reactions should therefore be challenging from the point of view of organic chemistry. In this paper, we demonstrated the efficient synthesis of deuterated benzyladenine and its application as the surrogate for the quantification of residual benzyladenine in agricultural products.
RESULTS AND DISCUSSION
Synthesis of deuterated benzyladenine
The H–D exchange reaction of benzyladenine using 10% Pd/C as a catalyst at room temperature was first investigated. The deuteration hardly took place on the purine ring at room temperature (Table 1, Entry 1), while raising the temperature to 110 °C led to the complete and undesirable removal of the N6-benzyl group to give the corresponding adenine as the sole product (Entry 2). The hydrogenolysis of the benzylamino moiety was tolerated at room temperature by the replacement of the catalyst with 5% Pt/C, which is generally effective for the H–D exchange on the aromatic nuclei, but the deuterium efficiency was only 20% at the benzylic position and purine nucleus (Entry 3). The deuterium efficiency was improved to approximately 35% by increasing the temperature to 110 °C, although the partial debenzylation instead occurred (Entry 4).
We previously developed a Pd/C-ethylenediamine complex [Pd/C(en)] on the basis of the catalyst poisoning property of ethylenediamine, which possesses no catalytic activity for the hydrogenation of various reducible functionalities including benzyl ethers and benzyl alcohols.35,36 The Pd/C(en) was then employed as the catalyst in anticipation of the high deuterium incorporation without removal of the benzyl group.37 As a result, the N6-debenzylation was efficiently suppressed during the deuteration of benzyladenine at 110 °C with moderate deuterium contents (Entry 5). The obtained deuterium-labeled benzyladenine was again used as the substrate for the deuteration. Thus, it was stirred in D2O at 180 °C together with 5% Pt/C under a hydrogen atmosphere. The deuterium incorporation efficiency was slightly improved, but not enough for practical use (Entry 6).
A synergistic effect of the simultaneous use of Pd/C and Pt/C on the H–D exchange reactions was previously observed, which efficiently incorporates deuterium atoms into both aromatic and aliphatic moieties of a variety of substituted arenes.38 The combined use of 5% Pd/C(en) and 5% Pt/C was then examined (Table 2, Entries 7 and 8). The deuterium efficiency was improved at 110 °C compared to the single use of either 5% Pt/C or 5% Pd/C(en) (Entry 7 vs. Entries 4 and 5, respectively), and the debenzylation, which was observed in the 5% Pt/C-catalyzed reaction (Entry 4), was suppressed probably because of the catalyst poison effect by the coordinated ethylenediamine of 5% Pd/C(en) (Entry 7). The increase in the reaction temperature to 180 °C remarkably promoted the H–D exchange reaction, although the debenzylation was also observed (Entry 8). We finally found that the independent use of an increased amount of 5% Pd/C(en) (30% of the substrate weight) at 180 °C led to an excellent deuterium incorporation, but with the lowest acceptable yield level (Entry 9). The deuterated benzyladenine obtained from Entry 9 in Table 1 was employed for its quantitative analyses in agricultural products.
Quantitative analysis of benzyladenine
Selection of the surrogate
The total ion chromatogram (TIC) of the deuterated benzyladenine showed several ion peaks for m/z 230.1, 231.1, 232.2, and so on. The compound derived from the highest peak, m/z 231.1, was selected as the surrogate, which was supposed to be the protonated benzyladenine-d5. Its MS/MS spectrum indicated the following three ions: m/z 230.9 for [M+H]+ of benzyladenine-d5, 152.0 for [M–benzene], 94.0 for benzyl-d3 (Figure 2). The comparison with the MS/MS spectrum of the non-deuterated benzyladenine supported the structure of the surrogate as benzyladenine-d5 which has a total 5 deuterium atoms, i.e., 1 deuterium on the benzene ring, two on the benzyl site, and two on the adenine nucleus.
When the product ions of the precursor ion for m/z 225.9 of the protonated benzyladenine were monitored in Q3, the ion for m/z 91.0 was observed with the highest intensity. Therefore, the ion was assigned as the target ion and the ion for m/z 226.0 as the qualifier ion. The ion for m/z 94.0 with the highest sensitivity was determined as the internal standard by the similar monitoring of the product ion of the ion for m/z 230.9 of the deuterated benzyladenine.
Recovery of the benzyladenine from agricultural products
Ion suppression effects of the acetonitrile extracts from agricultural products by mass spectrometry were first investigated (Table 2).39 The ratio of the MS peak area of the matrix standards extracted from grapefruit and lemon to that of the solvent standards were 0.84 and 0.83, respectively. Benzyladenine added on these products was not well recovered (83.4 and 75.7%, respectively) by the external standard method. The good correlation between the ratio of the matrix standards to solvent standards and the recovery ratio of grapefruit and lemon suggests that the low recovery should be attributed to the ion suppression effect. On the other hand, the use of benzyladenine-d5 as the internal standard improved the recovery from grapefruit and lemon up to 98.7 and 94.2%, respectively. Furthermore, the surrogate method was applicable for the recovery tests of all 15 products without any loss in sensitivity. The limit of detection (S/N = 3) of benzyladenine in fruits and vegetables was 0.25 ng/g, and 0.50 ng/g in cereals and beans.
CONCLUSIONS
Deuterium-labeled benzyladenine was successfully prepared by the H–D exchange reaction of benzyladenine using the Pd/C(en)–D2O–H2 system. The conditions achieved a high deuterium efficiency on the purine nuclei and benzylic position with minimal removal of the N6-benzyl group. The 1H NMR spectrum and LC/MS/MS analysis demonstrated that five hydrogen atoms of benzyladenine were replaced with deuterium atoms. The deuterium-labeled benzyladenine was successfully employed as the surrogate to quantify the residual benzyladenine in agricultural products using LC/MS/MS with a high sensitivity and reproducibility.
EXPERIMENTAL
Synthesis of deuterated benzyladenine
General. The 1H and 2H NMR spectra were recorded by a JEOL AL-400 or EX-400 spectrometer (400 MHz for 1H NMR, 61 MHz for 2H NMR). The chemical shifts (δ) are given in parts per million referenced to tetramethylsilane or residual solvent (2.49 ppm/DMSO). The deuterium content was determined using the internal standard 1,4-dimethoxybenzene. The EI mass spectra were taken by a JEOL JMS-SX 102A spectrometer. The analytical thin-layer chromatography (TLC) was carried out on pre-coated Silica Gel 60 F254 plates (Merck, Art 5715) and visualized with UV light. Column chromatography was performed using Merck silica gel 60 (230–400 mesh). 10% Pd/C, 5% Pd/C(en), and 5% Pt/C were obtained from the N.E. Chemcat Corporation, Wako Pure Chemical Industries, Ltd., and Sigma-Aldrich Corporation, respectively. Deuterium oxide (99.9% isotopic purity) was purchased from Cambridge Isotope Laboratories, Inc., or Division of Spectra Gases, Inc. Benzyladenine (99.9% purity) was obtained from Hayashi Pure Chemical Industries, Ltd. All other reagents were obtained from commercial sources and used without further purification.
General procedures for the H–D exchange reaction (Table 1)
Method A: A mixture of benzyladenine (113 mg, 0.500 mmol) and catalyst (23.0 mg, 20 wt % of benzyladenine) in D2O (1–2 mL) under a hydrogen atmosphere in a stainless sealed tube was stirred at 110–180 °C (oil bath). After 24 h, the mixture was diluted with MeOH (5 mL) at room temperature and passed through a membrane filter (Millipore Millex-LG, 0.45 μm) to remove the catalyst. The catalyst collected on the filter was washed with MeOH (2 × 5 mL), and the filtrate was concentrated in vacuo. The residue was purified by silica-gel column chromatography (CHCl3:MeOH = 10:1).
Method B: A mixture of benzyladenine (113 mg, 0.500 mmol) and catalyst (23.0 mg, 20 wt % of benzyladenine) in D2O (1–2 mL) under a hydrogen atmosphere in a 15 mL-test tube was stirred at room temperature. After 24 h, the mixture was diluted with MeOH (5 mL) and passed through a membrane filter (Millipore Millex-LG, 0.45 μm) to remove the catalyst. The catalyst collected on the filter was washed with MeOH (2 × 5 mL), and the filtrate was concentrated in vacuo. The residue was then purified by silica-gel column chromatography (CHCl3:MeOH = 10:1).
Entry 1. Method B. 10% Pd/C (23.0 mg, 20% of substrate weight) was used as the catalyst instead of 5% Pt/C. Yield: deuterated benzyladenine, 47.5 mg (42%); adenine, 26.9 mg (24%, D efficiency was not determined).
Entry 2. Method A. 10% Pd/C (24.0 mg, 20% of substrate weight) was used instead of 5% Pt/C. The reaction was carried out at 110 °C. Debenzylation took place.
Entry 3. Method B. Yield: deuterated benzyladenine, 97.5 mg (86%).
Entry 4. Method A. The reaction was carried out at 110 °C. Yield: deuterated benzyladenine, 68.0 mg (60%); adenine, 22.8 mg (20%, D efficiency was not determined).
Entry 5. Method A. 5% Pd/C(en) [23.0 mg, 20% of substrate weight] was used as the catalyst. The reaction was carried out at 110 °C. Yield: deuterated benzyladenine, 104 mg (92%).
Entry 6. Method A. The reaction was carried out at 180 °C. Yield: deuterated benzyladenine, 92.3 mg (82%).
Entry 7. Method A. 5% Pd/C(en) [23.0 mg, 20% of substrate weight] and 5% Pt/C (23.0 mg, 20% of substrate weight) were used. The reaction was carried out at 110 °C. Yield: deuterated benzyladenine, 92.9 mg (82%).
Entry 8. Method A. 5% Pd/C(en) [23.0 mg, 20% of substrate weight] and 5% Pt/C (23.0 mg, 20% of substrate weight) were used. The reaction was carried out at 180 °C. Yield: deuterated benzyladenine, 79.1 mg (70%); deuterated adenine, 23.7 mg [21%, 94% deuterium efficiency (average of the 2 and 8 positions) ].
Entry 9. Method A. 5% Pd/C(en) [34.0 mg, 30% of substrate weight] was used as the catalyst. The reaction was carried out at 180 °C. Yield: deuterium benzyladenine, 53.0 mg (47%); deuterated adenine, 27.1 mg [24%, 96% deuterium efficiency (average of the 2 and 8 positions) ]. 1H NMR (DMSO-d6, 1,4 dimethoxybenzene as an internal standard) δ 8.16–8.09 (s, 0.12H), 7.35–7.19 (m, 4.17H), 4.68 (s, 0.06H). 2H NMR (DMSO) δ 8.16 (br s), 4.71 (br s).
Quantification of benzyladenine
Materials
Acetonitrile, MeOH, and NH4OAc were purchased from Kanto Chemical Co., Inc. The membrane and glass filters were obtained from Millipore (Millipore Millex-LG SLLG H13 NL, 0.20 μm) and Advantec (GA-100, 1.0 μm), respectively. The deuterated benzyladenine was prepared by the method described in the general procedure for the H–D exchange reaction (Table 1), Entry 9.
Apparatus and conditions
HPLC apparatus and conditions
An Agilent 1200 LC (SL) system with an Agilent ZORBAX Eclipse Plus C18 column (2.1 mm i.d. × 150 mm, 1.8 µm particle size) was used. The HPLC conditions were as follows: Flow rate, 0.2 mL/min; Column temperature, 40 °C; Injection volume, 5 µL; Mobile phase, Solvent A: 5 mmol/L aqueous NH4OAc solution, Solvent B: 5 mmol/L NH4OAc solution in MeOH, Gradient profile, 0 min, A:B = 85:15; 1 min, A:B = 60:40, 3.5 min, A:B = 60:40; 6 min, A:B = 50:50, 8 min, A:B = 45:55; 17.5 min, A:B = 5:95, 30 min, A:B = 5:95.
Mass spectrometry (MS) analysis
Table 3. MRM parametersQ1 Mass (Da)Q3 Mass (Da)Dwell (msec)DP (V)CE (eV)CXP (V)benzyladenine225.9226.010071914225.991.0100713314benzyladenine-d5230.9230.910076944230.994.0100763516Quantification was performed using an Applied Biosystems API4000 Q TRAPTM MS/MS system. Multiple reaction monitoring (MRM) analyses were conducted in the positive electrospray ionization (ESI) mode. The ESI conditions were as follows: curtain gas, 30 psi; ion source gas 1, 80 psi; ion source gas 2, 30 psi; ion spray voltage, 4500 V; temperature, 700 °C. MRM parameters are indicated in Table 3.
Preparation of standard solutions
Solvent standard (Solvent STD)
A 5.0 ng/mL benzyladenine solution in a mixed solvent of acetonitrile and H2O (1:1).
Matrix standard (Matrix STD)
Acetonitrile (1 mL) was added on the surface of each vegetable (20 g), fruit (20 g), cereal (10 g), or beans (10 g). To the cereal and beans was also added H2O (20 mL). After 30 min, acetonitrile (approximately 130 mL) was added, and the agricultural product was homogenized for over 3 min. The mixture was then filtered using a glass filter (1.0 μm), and the filtrate was diluted with acetonitrile to 200 mL. The solution (2 mL) was concentrated in vacuo to dryness, and a 10 ng/mL benzyladenine solution (2.0 mL) in a mixed solvent of acetonitrile and H2O (1:1) to the residue. The resulting solution was diluted with acetonitrile–H2O (1:1) to 4.0 mL.
Preparation of sample solution
The acetonitrile solutions of benzyladenine-d5 (4.0 mg/L, 1 mL) and of benzyladenine (2.0 mg/L, 1 mL) were added on the surface of each vegetable (20 g), fruit (20 g), cereal (10 g), or beans (10 g). To the cereal and beans was also added H2O (20 mL). After 30 min, acetonitrile (approximately 130 mL) was added, and the agricultural product was homogenized for over 3 min. The mixture was then filtered using a glass filter (1.0 μm), and the filtrate was diluted with acetonitrile to 200 mL. The solution (1 mL) was diluted with H2O to 2 mL, and passed through a membrane filter (0.20 μm). The filtrate was used for the MRM analysis using LC/MS/MS.
ACKNOWLEDGEMENTS
We thank the N.E. Chemcat Corporation and Wako Pure Chemical Industries, Ltd., for the catalyst gifts.
References
1. D. S. Letham and C. O. Miller, Plant Cell Physiol., 1965, 6, 355.
2. A. C. Leopold and M. Kawase, Amer. J. Bot., 1964, 51, 294. CrossRef
3. R. A. Scott and J. L. Lieverman, Plant Physiol., 1956, 31, 321. CrossRef
4. P. M. Bessey, Exp. Sta. Rep., 1960, 189, 5.
5. F. W. Zink, J. Agric. Food Chem., 1961, 9, 304. CrossRef
6. M. Barreda, F. J. Lopez, M. Villarroya, J. Beltran, J. M. Garcia-Baudin, and F. Hernandez, J. AOAC Int., 2006, 89, 1080.
7. T. Otake, N. Itoh, Y. Aoyagi, M. Matsuo, N. Hanari, S. Otsuka, and T. Yarita, J. Agric. Food Chem., 2009, 57, 8208. CrossRef
8. E. Ueno, H. Oshima, I. Saito, H. Matsumoto, Y. Yoshimura, and H. Nakazawa, J. AOAC Int., 2004, 87, 1003.
9. J. E. Fox, J. L. Erion, and J. D. McChesney, Phytochemistry, 1979, 18, 1055. CrossRef
10. O. V. Lushkina, G. V. Sidorov, and N. F. Myasoedov, Bioorg. Khim., 1993, 19, 113.
11. G. V. Sidorov and N. F. Myasoedov, J. Labelled Compd. Radiopharm., 1994, 34, 353. CrossRef
12. J. Filippi and J. Guern, B. Soc. Chim. Fr., 1965, 2617.
13. M. Del Carmen, G. Barrio, D. I. C. Scopes, J. B. Holtwick, and N. J. Leonard, Pro. Natl. Acad. Sci. USA, 1981, 78, 3986. CrossRef
14. N. J. Leonard and T. R. Henderson, J. Am. Chem. Soc., 1975, 97, 4990. CrossRef
15. T. Fujii, T. Saito, K. Kizu, H. Hayashibara, Y. Kumazawa, S. Nakajima, and T. Fujisawa, Chem. Pharm. Bull., 1991, 39, 301.
16. R. K. Hill, N. D. Ledford, and L. A. Renbaum, J. Labelled Compd. Radiopharm., 1985, 22, 143. CrossRef
17. M. Sako, T. Hayashi, K. Hirota, and Y. Maki, Chem. Pharm. Bull., 1992, 40, 1656.
18. V. Derdau, J. Atzrodt, J. Zimmermann, C. Kroll, and F. Brückner, Chem. Eur. J., 2009, 15, 10397. CrossRef
19. C. B. Lebrilla and W. F. Maier, J. Am. Chem. Soc., 1986, 108, 1606. CrossRef
20. G. M. Rubottom and E. J. Evain, Tetrahedron, 1990, 46, 5055. CrossRef
21. B. Rybtchinski, R. Cohen, Y. Ben-David, J. M. R. Martin, and D. Milstein, J. Am. Chem. Soc., 2003, 125, 11041. CrossRef
22. T. A. Weil, S. Friedman, and I. Wender, J. Org. Chem., 1974, 39, 48. CrossRef
23. J. Zhou and J. F. Hartwig, Angew. Chem. Int. Ed., 2008, 47, 5783. CrossRef
24. T. Kurita, K. Hattori, S. Seki, T. Mizumoto, F. Aoki, Y. Yamada, K. Ikawa, T. Maegawa, Y. Monguchi, and H. Sajiki, Chem. Eur. J., 2008, 14, 664. CrossRef
25. H. Sajiki, K. Hattori, F. Aoki, K. Yasunaga, and K. Hirota, Synlett, 2002, 1149. CrossRef
26. H. Esaki, F. Aoki, M. Umemura, M. Kato, T. Maegawa, Y. Monguchi, and H. Sajiki, Chem. Eur. J., 2007, 13, 4052. CrossRef
27. H. Sajiki, F. Aoki, H. Esaki, T. Maegawa, and K. Hirota, Org. Lett., 2004, 6, 1485. CrossRef
28. H. Esaki, F. Aoki, T. Maegawa, K. Hirota, and H. Sajiki, Heterocycles, 2005, 66, 361. CrossRef
29. H. Sajiki, H. Esaki, F. Aoki, T. Maegawa, and K. Hirota, Synlett, 2005, 1385. CrossRef
30. N. Ito, H. Esaki, T. Maesawa, E. Imamiya, T. Maegawa, and H. Sajiki, Bull. Chem. Soc. Jpn., 2008, 81, 278. CrossRef
31. H. Sajiki, N. Ito, H. Esaki, T. Maesawa, T. Maegawa, and K. Hirota, Tetrahedron Lett., 2005, 46, 6995. CrossRef
32. T. Maegawa, Y. Fujiwara, Y. Inagaki, H. Esaki, Y. Monguchi, and H. Sajiki, Angew. Chem. Int. Ed., 2008, 47, 5394. CrossRef
33. T. Maegawa, Y. Fujiwara, Y. Inagaki, Y. Monguchi, and H. Sajiki, Adv. Synth. Catal., 2008, 350, 2215. CrossRef
34. P. G. M. Wuts and T. W. Greene, 'Greene's Protective Groups in Organic Synthesis (4th ed.),' John Wiley & Sons, Inc., Hoboken, 2006. CrossRef
35. H. Sajiki, K. Hattori, and K. Hirota, J. Org. Chem., 1998, 63, 7990. CrossRef
36. K. Hattori, H. Sajiki, and K. Hirota, Tetrahedron, 2001, 57, 4817. CrossRef
37. The H–D exchange reaction of benzylamine derivatives using homogeneous Ir complex, D2 gas in DMF or DMA regioselectively proceeded on the ortho-positions in benzene rings without removal of ammonia. M. J. Hickey, J. R. Jones, L. P. Kingston, W. J. S. Lockley, A. N. Mather, B. M. McAuley, and D. J. Wilkinson, Tetrahedron Lett., 2003, 44, 3959. CrossRef
38. N. Ito, T. Watahiki, T. Maesawa, T. Maegawa, and H. Sajiki, Adv. Synth. Catal., 2006, 348, 1025. CrossRef
39. T. M. Annesley, Clinical Chemistry, 2003, 49, 1041. CrossRef