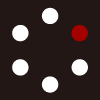
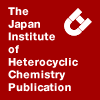
HETEROCYCLES
An International Journal for Reviews and Communications in Heterocyclic ChemistryWeb Edition ISSN: 1881-0942
Published online by The Japan Institute of Heterocyclic Chemistry
e-Journal
Full Text HTML
Received, 10th November, 2010, Accepted, 13th December, 2010, Published online, 17th December, 2010.
DOI: 10.3987/COM-10-12102
■ Efficient Synthesis of Functionalized Diazacyclopenta[c]pentalene with Multiple Intermolecular Interactions in Crystal
Konstantin V. Kudryavtsev*
Department of Chemistry, Lomonosov Moscow State University, Leninskie Gory 1-3, 119991, Moscow, Russia
Abstract
Atom economic synthesis of functionalized polysubstituted racemic diazacyclopenta[c]pentalene 7 has been developed using sequential organocatalytic 1,3-dipolar cycloaddition of azomethine ylide with maleic anhydride and three successive amide bonds formation induced by interaction of 4-fluorobenzylamine with heterocyclic anhydride 5. Enantiomers of 7 are connected by Cl-π, H···F and C=O···H intermolecular interactions in crystal.INTRODUCTION
Different classes of natural compounds are characterized by polycyclic molecular skeletons. Attaching of functional groups at definite positions of polycyclic scaffold could be responsible for specific biological activities of natural products and their derivatives through formation of selected ligand-target interactions.1 Non-aromatic complex carbon- and heteropolycyclic compounds are objects of diversity-oriented synthesis (DOS) since the possibility to regulate scaffold appendages along the spherical surface of ligand exists.2 Introduction of different functional groups and/or substituents in three-dimensional space of polycyclic molecular skeleton allows to generate additional diversity and to optimize discovered biological activity. The fusion of several non-aromatic rings provides geometrically well-defined structures that are important for both receptor fitting and covering of pharmaceutically relevant chemical space.3 Several synthetic strategies including cascade reactions1 and aza-annulations4 are utilized for effective construction of polycyclic skeleton of natural compounds. In this communication we report a three-step synthetic procedure toward the well-defined functionalized diazatricyclic scaffold 1, heterocyclic analog of angular triquinane 2,5 that is a constituent of a wide family of sesquiterpene natural products (Figure 1). The only published method of synthesis of heterocycles 3 possessing molecular scaffold 1 is based on interaction of N-allyl-N-phenylprolinamides with benzaldehydes.6
RESULTS AND DISCUSSION
Our approach to functionalized diazacyclopenta[c]pentalene scaffold included three operational steps (Scheme 1). At the first stage iminoester 4 was synthesized from 4-chlorobenzaldehyde and glutamate dimethyl ester hydrochloride. Cycloaddition of azomethine ylides generated from iminoesters and dipolarophiles is a widely used method of synthesis of functionalized pyrrolidines derivatives.7 Typically interaction of 1,3-dipoles with olefins occurs under Lewis or Bronsted acids catalysis or requires elevated temperatures.7
Recently we reported an amino acid catalyzed cycloaddition of activated dipolarophiles (maleimides, maleic anhydride) to azomethine ylides under mild conditions.8 Cycloadditions of 1,3-azadipoles with maleic anhydride are insufficiently studied because of the last reagent reactivity towards nucleophilic species and reagents. Our method8 overcomes these limitations and provides approach to heterocyclic anhydrides but in case of iminoester 4 leads to pyrrolidine tricarboxylic acid anhydride 5 contaminated with an amino acid catalyst. To perform reaction homogeneously we have used soluble in non-polar solvents N-tert-butoxycarbonyl glycine (N-BocGly) as a Bronsted acid catalyst of cycloaddition process9 and succeeded in good yield of functionalized pyrrolidine 5 (Scheme 1). Presumably N-BocGly protonates iminoester 4 at the first step and subsequent deprotonation of α-position leads to ylide A formation (Scheme 2). endo-Cycloaddition of maleic anhydride to the reactive species A gives rise to the stereoselective formation of four stereogenic centers in molecule 5. Relative cis-stereochemistry of protons at 6-, 6a- and 3a-positions of pyrrolidine ring of heterocyclic anhydride 5 is deduced from the 9.1 Hz value of 3J(H6-H6a) constant in compound 5 1H NMR spectrum10 and corresponds to our previous results on cycloaddition of maleic anhydride to other types of azomethines catalyzed by amino acids.8
Polyfunctional nature of bicyclic anhydride 5 prompted us to study it’s reactivity toward nitrogen nucleophiles. There are four possible positions for nucleophilic attack in 5 according to number of carbonyl functions (Scheme 2). The most reactive functionalities should be anhydride carbonyls (Scheme 2, routes a and b) and in case b primary nucleophilic interaction with anhydride could be followed by cyclization at 4-carboxymethyl group leading to annulation at 3a,4-bond of starting heterocycle 5. Inramolecular interaction of 5-nitrogen and distal carboxymethyl group (Scheme 2, route c) could provide annulation at 4,5-bond of starting heterocycle 5. Considered lactamization may take place spontaneously11a or under elevated temperatures11b and has been used by us for construction of pyrrolizidin-3-one scaffold.11
Treatment of THF solution of anhydride 5 with equimolar amount of 4-fluorobenzylamine led to expected consumption of starting materials (Scheme 1). The progress of the reaction was monitored by 1H NMR of concentrated at room temperature probes. After the changes in the reaction mixture ceased we observed a predominant product in 1H NMR. Thorough analysis of obtained 1H NMR data let us to assign structural formula 6 to the major product (Scheme 1). Formation of substituted octahydropyrrolo[3,4-b]pyrrole 6 corresponds to the discussed above tandem 3a,4-annulation of anhydride 5 induced by 4-fluorobenzylamine (Scheme 2, route b). The second step of this process occurred spontaneously at remarkably mild conditions: formation of the imide bridge in our recent approach towards 3,6-diazabicyclo[3.2.1]octane scaffold took place at elevated temperatures and in the presence of copper salt catalyst.12 Subsequent heating of the mixture contained predominantly compound 6 in acetonitrile led to the isolation after purification of a major component – functionalized diazacyclopenta[c]pentalene 7 (Scheme 1).
Structure of the novel polysubstituted diazatricycle 7 was unambiguously determined by X-ray analysis (Figure 2).13 Epimerization of β-carboxylic function took place apparently during the lactamization step at elevated temperature, all other stereochemical outcomes accord with considered reactions pathways. Detailed examination of solid state structure of 7 revealed two types of interesting intermolecular interactions in crystal (Figures 3, 4). The first type of intermolecular contacts comprises rarely observed CAr–F···H–Cα–N contact14 and more frequent Cl–π interaction15 with both halogen atoms belonging to one enantiomeric molecule 7 and connecting to the benzylic part of another (Figure 3). The distance between F(1) and H(16AB) atoms is significantly below the sum of the van der Waals radii (2.67Å) and amounts to 2.20 Å (Figure 3). The chlorine atom Cl(1) is located 3.50 Å from aromatic carbon C(19A) (Figure 3). This distance practically coincides with 3.45 Å sum of chlorine-carbon van der Waals radii and appropriate interaction could provide up to 2 kcal/mol energetic benefit.15a Both types of observed in 7 non-covalent bonding exampled in complexes of thrombin with small-molecule inhibitors. CAr–F···H–Cα–C=O contact has been observed in inhibitor-enzyme complex for 4-fluorobenzyl fragment of an inhibitor and Asn98 in the S3-pocket of the thrombin active site.14 Chlorine–π contacts are characteristic for chloroaryl fragments of small-molecule inhibitors interacting with Tyr228 in the S1-pocket of the thrombin active site.15 We have tested diazacyclopenta[c]pentalene 7 on thrombin inhibition activity and unfortunately found only poor enzyme inactivation.16 Another type of intermolecular interactions in crystal of 7 represents canonical hydrogen bonds between carbonyl oxygen atoms and carboxylic protons (Figure 4). Two mirror enantiomers of fused diazatricycle 7 are connected by two practically linear O···H–O interactions forming 16-membered circular chain (Figure 4).
In conclusion we have developed short and convenient synthetic approach towards functionalized octahydro-2,5a-diazacyclopenta[c]pentalene (1) scaffold. It was demonstrated the effectiveness of application of N-tert-butoxycarbonyl α-amino acid as a homogeneous organocatalyst of 1,3-dipolar cycloaddition of activated dipolarophile to azomethine. The method is characterized by good atom economy with only one molecule of water and two molecules of methanol excreted during the whole synthetic cycle. Different intermolecular interactions in functionalized diazacyclopenta[c]pentalene 7 monocrystal could be used in crystal engineering and structure-based design of enzymes inhibitors.
EXPERIMENTAL
Reagents were obtained from Alfa Aesar and used without further purification unless otherwise stated. Solvents were dried using standard procedures. Reactions were monitored by thin layer chromatography (TLC) on precoated silica gel plates (Sorbfil) with a UV indicator. Melting points were determined in open capillary and are uncorrected. 1H NMR and 13C NMR spectra were recorded with a Bruker Avance 400 MHz spectrometer. The chemical shifts (δ) are reported in parts per million upfield using residual signals of solvents as internal standards. Coupling constants (J values) were measured in hertz (Hz). Combustion analyses were performed with a Carlo Erba CHN analyzer.
Dimethyl 2-(4-chlorobenzylideneamino)pentanedioate (4)
Under argon atmosphere 5.02 ml (3.643 g, 36 mmol) of NEt3 were added to a suspension of 7.620 g (36 mmol) of glutamic acid dimethyl ester hydrochloride and 7.222 g (60 mmol) of MgSO4 in 100 mL of CH2Cl2. After 1 h stirring at rt 4-chlorobenzaldehyde (4.217 g, 30 mmol) was added and the resulted mixture was stirred at rt overnight. The solid was removed by filtration and the filtrate was washed once with H2O. The aqueous phase was extracted once with 30 mL of CH2Cl2 and the combined organic layers were washed with brine. The organic phase was dried over Na2SO4, filtered and concentrated. The product was isolated as yellowish oil, 8.900 g (100%), and used in the next step without additional purification. 1H NMR (400 MHz; CDCl3): δ 8.26 (s, 1H, CH=), 7.72 (d, J = 8.4, 2H, Ar), 7.40 (d, J = 8.4, 2H, Ar), 4.06 (dd, J = 8.0, 5.0, 1H, CHN), 3.76 (s, 3H, OCH3), 3.66 (s, 3H, OCH3), 2.43-2.21 (m, 4H, CH2CH2).
Methyl (3aR*,4S*,6R*,6aS*)-6-(4-chlorophenyl)-4-(3-methoxy-3-oxopropyl)-1,3-dioxohexahydro-1H-furo[3,4-c]pyrrole-4-carboxylate (5)
Iminoester 4 (4.500 g, 15.1 mmol) was dissolved in 20 mL of CH2Cl2 under air atmosphere and CH2Cl2 solutions of 1.480 g of maleic anhydride (15.1 mmol) and 0.264 g N-BocGly (1.5 mmol) were added sequentially. The mixture was stirred for 24 h at rt. The solution was concentrated under reduced pressure, and the residue was recrystallized from hexane/CH2Cl2. 5.083 g (85%), colorless crystals, mp 96-99 °C. 1H NMR (400 MHz; DMSO-d6): δ 7.42-7.37 (m, 4H, Ar), 4.70 (d, J = 9.1, 1H, H6), 3.98 (dd, J = 9.1, 7.8, 1H, H6a), 3.75 (d, J = 7.8, 1H, H3a), 3.72 (s, 3H, OCH3), 3.58 (s, 3H, OCH3), 2.58-2.52 (m, 1H, CH2), 2.18-2.14 (m, 2H, CH2), 2.10-2.02 (m, 1H, CH2). 13C NMR (100 MHz; DMSO-d6): δ 173.07, 171.89, 171.23, 170.31, 137.99, 132.61, 129.44 (2C), 128.54 (2C), 70.40, 59.87, 55.64, 52.61, 51.92, 50.38, 29.60, 28.54. Anal. Calcd for C18H18ClNO7: С, 54.62; Н, 4.58; N, 3.54. Found: С, 54.79; Н, 4.55; N, 3.81.
(3aR*,4R*,5R*,9aS*)-5-(4-Chlorophenyl)-2-(4-fluorobenzyl)-1,3,7-trioxooctahydro-7H-pyrrolo[3,4-g]pyrrolizine-4-carboxylic acid (7)
Anhydride 5 (1.000 g, 2.53 mmol) was dissolved in 25 mL of dry THF and 0.300 mL (0.320 g, 2.53 mmol) of 4-fluorobenzylamine were added to the solution. The mixture was stirred for 72 h at rt, concentrated under reduced pressure, and the residue was redissolved in 25 mL of MeCN. The solution was heated at 82 °C for 24 h, concentrated and the residue was recrystallized from CH2Cl2/MeOH. 0.703 g (61%), colorless crystals, mp 282-283 °C. 1H NMR (400 MHz; DMSO-d6): δ 13.36 (br.s, 1H, COOH), 7.15 (d, J = 8.6, 2H, Ar), 7.04-7.02 (m, 2H, Ar), 7.01-6.94 (m, 4H, Ar), 5.42 (s, 1H, H5), 4.27-4.16 (m, 2H, CH2Ar), 3.76 (s, 1H, H4), 3.67 (s, 1H, H3a), 2.99 (ddd, J = 16.4, 11.2, 9.2, 1H, H8), 2.58 (dd, J = 12.8, 9.2, 1H, H8), 2.45-2.39 (m, 1H, H9), 2.30 (dd, J = 12.8, 11.2, 1H, H9). 13C NMR (100 MHz; DMSO-d6): δ 178.06, 177.69, 175.66, 173.24, 161.94 (J 243.7), 137.34, 132.90, 131.23, 130.72, 130.64, 128.94 (2C), 127.04 (2C), 115.68, 115.47, 73.10, 61.57, 53.93, 52.41, 41.88, 32.89, 29.73. Anal. Calcd for C23H18ClFN2O5: С, 60.47; Н, 3.97; N, 6.13. Found: С, 60.69; Н, 4.05; N, 6.01.
Crystal structure determination of compound 7.
The single crystal of 7 of approximate dimensions 0.40 x 0.20 x 0.20 mm was mounted in inert oil on the top of glass fibre and transferred on the Bruker SMART APEX II diffractometer. Crystal data: C23H18ClFN2O5, M = 456.84, monoclinic, a = 13.6113(18), b = 12.5729(18), c = 12.4174(16) Å, β = 108.234(4)°, V = 2018.3(5) Å3, space group P21/c, Z = 4, Dc = 1.503 g/cm3, F(000) = 944, µ(Mo-Kα) = 0.239 mm-1. Total of 18287 reflections (4187 unique, Rint = 0.0457) were measured using graphite monochromatized Mo-Kα radiation (λ = 0.71073 Å) at 100 K. Data were collected in the range 1.58 < θ < 26.54 (-17 ≤ h ≤ 17, -15 ≤ k ≤ 15, -15 ≤ 1 ≤ 15). The structure was solved by direct methods17 and refined by full matrix least-squares on F2. All H atoms (except HO2) were placed in calculated positions and refined using a riding model. The final residuals were: R1 = 0.0527, wR2 = 0.0981 for 3076 reflections with I > 2σ(I) and 0.0784, 0.1079 for all data and 289 parameters. Goof = 1.108, maximum Δρ = 0.487 e/Å3.
ACKNOWLEDGEMENTS
This research was kindly supported by Russian Foundation for Basic Research (grants 08-04-01800-a and 09-03-92011-HHC_a) to K.V.K. Ms. Alexandra O. Borissova is acknowledged for X-ray investigation of compound 7.
References
1. M. Ihara, Chem. Pharm. Bull., 2006, 54, 765. CrossRef
2. M. D. Burke and S. L. Schreiber, Angew. Chem. Int. Ed., 2004, 43, 46. CrossRef
3. R. Messer, C. A. Fuhrer, and R. Haner, Curr. Opin. Chem. Biol., 2005, 9, 259. CrossRef
4. A. K. Lawrence and K. Gademann, Synthesis, 2008, 331. CrossRef
5. G. Mehta and A. Srikrishna, Chem. Rev., 1997, 97, 671. CrossRef
6. M. A. Marx, A.-L. Grillot, C. T. Louer, K. A. Beaver, and P. A. Bartlett, J. Am. Chem. Soc., 1997, 119, 6153. CrossRef
7. R. Grigg, Chem. Soc. Rev., 1987, 16, 89; CrossRef O. Tsuge and S. Kanemasa, 'Advances in Heterocyclic Chemistry: Recent Advances in Azomethine Ylide Chemistry,' Vol. 45, ed. by A. R. Katritzky, Academic Press, Inc., London, 1989, pp. 231-349.
8. K. V. Kudryavtsev and A. A. Zagulyaeva, Russ. J. Org. Chem., 2008, 44, 378. CrossRef
9. This is the first published example of application of N-acylated α-amino acids as a Bronsted acid catalyst for 1,3-dipolar cycloaddition. We did not observe any appreciable conversion of 4 and maleic anhydride mixture in CH2Cl2 during 24 h in the absence of N-BocGly. Gong et al. used chiral phosphoric acids as organocatalysts of azomethine ylides and dimethyl maleate asymmetric cycloaddition: X.-H. Chen, W.-Q. Zhang, and L.-Z. Gong, J. Am. Chem. Soc., 2008, 130, 5652. CrossRef
10. S. Cabrera, R. G. Arrayas, and J. C. Carretero, J. Am. Chem. Soc., 2005, 127, 16394. CrossRef
11. K. V. Kudryavtsev, N. V. Nukolova, O. V. Kokoreva, and E. S. Smolin, Russ. J. Org. Chem., 2006, 42, 412; CrossRef K. V. Kudryavtsev, Russ. Chem. Bull. Int. Ed., 2008, 57, 2364. CrossRef
12. K. V. Kudryavtsev, Russ. J. Org. Chem., 2010, 46, 372. CrossRef
13. Crystallographic data (excluding structure factors) for the compound 7 have been deposited with the Cambridge Crystallographic Data Centre as supplementary publication no. CCDC-761400. Copies of the data can be obtained free of charge on application to CCDC, 12 Union Road, Cambridge CB2 1EZ, UK [Fax: int.code + 44(1223)336-033; E-mail: deposit@ccdc.cam.ac.uk]..
14. J. A. Olsen, D. W. Banner, P. Seiler, B. Wagner, T. Tschopp, U. Obst-Sander, M. Kansy, K. Muller, and F. Diederich, ChemBioChem, 2004, 5, 666. CrossRef
15. Y. N. Imai, Y. Inoue, I. Nakanishi, and K. Kitaura, Protein Science, 2008, 17, 1129; CrossRef B. Baum, M. Mohamed, M. Zayed, C. Gerlach, A. Heine, D. Hangauer, and G. Klebe, J. Mol. Biol., 2009, 390, 56. CrossRef
16. Compound 7 at 3.7 mM concentration inhibits 57% of thrombin activity in buffer solution.
17. G. M. Sheldrick, 'SHELXTL v. 5.10, Structure Determination Software Suite', Bruker AXS, Madison, Wisconsin, USA, 1998.