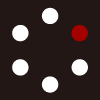
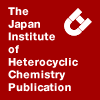
HETEROCYCLES
An International Journal for Reviews and Communications in Heterocyclic ChemistryWeb Edition ISSN: 1881-0942
Published online by The Japan Institute of Heterocyclic Chemistry
e-Journal
Full Text HTML
Received, 11th November, 2010, Accepted, 6th June, 2011, Published online, 8th June, 2011.
DOI: 10.3987/COM-10-S(P)2
■ New Hydrazine-Based Organocatalyst for Asymmetric Diels-Alder Reaction of 1,2-Dihydropyridines
Yuko Okuyama, Kenichi Osone, Hiroto Nakano, and Mitsuhiro Takeshita*
Tohoku Pharmaceutical University, 4-4-1 Komatsushima, Aoba-ku, Sendai 981-8558, Japan
Abstract
Chiral hydrazinoalcohol organocatalyst is designed and synthesized as a new organocatalyst for the enantioselective Diels-Alder reaction between 1,2-dihydropyridines and acrolein derivatives to produce an optical active isoquinuclidine derivative (up to 67% ee with up to 96% yield).The development of the new chiral organocatalysts for use in asymmetric catalytic reactions has drawn considerable interest in the last 10 years. Excellent covalent and non-covalent organocatalysts have been developed for use in a wide range of reactions.1 Quite recently, we also developed the oxazolidine organocatalyst2 1 that works as the efficient catalyst as with MacMillan’s imidazolidone-based catalyst3 in the asymmetric Diels-Alder (DA) reaction of 1,2-dihydropyridines4 3 which is an important reaction for the construction of chiral isoquinuclidines (2-azabicyclo[2.2.2]octanes) 4. The molecules 4 is valuable synthetic intermediates of biological active iboga-type alkaloids5 and oseltamivir phosphate (Tamiflu)6 (Scheme 1). Both the oxazolidine and the MacMillan catalysts have only a covalent site in the molecules, and the enantioselectivity in the DA reaction is controlled by the substituents at its both sides of the corresponding covalent site. In the present study, we planned to develop a new organocatalyst that has both covalent and non-covalent sites in the molecule (Figure 1). As the molecule, we paid attention to hydrazinoalcohol 2. Although the molecule 2 was used to form a chiral diazomethine ylide unit,7 the use of the molecule 2 as an organocatalyst has not been reported yet. Molecule 2 has both hydrazinyl covalent and hydroxyl non-covalent sites (Figure 1). As the advantage point of catalyst 2, strong nucleophilic hydrazinyl primary amino group might afford higher chemical yield in comparison with the reaction of diene while oxazolidine catalyst 1 having nucleophilic secondary amino group as a covalent site.
Furthermore, it is expected that the catalyst 2 might be able to control the attack of diene by both the hydrogen bonding interactions of the hydroxyl group with the carbonyl group on 1,2-dihydropyridines and the bulky substituent at β-position on 2 to afford high enantioselectivity in the reaction, although oxazolidine catalyst 1 controls the attack of diene only by substituents at its both sides of amino covalent site (Scheme 1). In this paper, we describe the newly designed hydrazinoalcohols in the asymmetric DA reaction of 1,2-dihydropyridines with acroleins as organocatalyst.
The catalysts 2a-c were prepared from the corresponding β-amino alcohols7 5a-c (Scheme 2). Thus, the condensation of 5a-c with benzaldehyde, followed by the treatment with NaBH4 afforded N-benzyl amino alcohols 6a-c. The nitrosoation of 6a-c followed by the reduction of the obtained 7a-c using LiAlH4 afforded the desired hydrazinoalcohol catalysts 2a-c in good to moderate yields (50-72%).
We first examined the DA reaction of common 1-phenoxycarbonyl-1,2-dihydropyridine 3a (2 equiv.) with acrolein 8 (1 equiv.). The reaction was carried out at room temperature or 0 °C in MeCN-H2O in the presence of 30 mol% of catalysts 2a-c and 30 mol% of CF3CO2H as the additive to give the DA adduct 9a, and its chemical and optical yields were determined by converting to the alcohol 10a (Scheme 3). The results are summarized in Table 1.
The reaction catalyzed by β−Ph-2a did not afford satisfactory results for the enantioselectivity and chemical yield (entry 1: 3% ee, 17%). On the other hand, β−benzyl-2b gave the corresponding endo-DA adduct 10a in good enantioselectivity and fairly good chemical yield (entry 2: 66% ee, 93%). However, the use of bulkier β−tert-butyl-2c brought about a decrease in both enantioselectivity and chemical yield (entry 3: 55% ee, 46%).
The activity of the most effective catalyst 2b was then evaluated in the reaction consisting of 30 mol% catalyst with 1-benzyloxycarbonyl-1,2-dihydropyridine 3b (2 equiv.) and 8 (1 equiv.) (Scheme 3 and Table 1). The chemical and optical yields of the DA adduct was determined by converting to alcohol 10b. As the results, catalyst 2b afforded good enantioselectivity (65% ee) with excellent chemical yield (96%) (entry 4). Furthermore, the reaction at 0 ˚C under the same conditions using catalyst 2b also gave good enantioselectivity (67% ee) and excellent chemical yield (95%) (entry 5). From the above results, β−benzyl-2b is better than catalysts 2a,c as an organocatalyst for affording higher enantioselectivity in this DA reaction. Although catalyst 2b showed higher catalytic activity than oxazolidine catalyst for the chemical yield in this reaction, the enantioselectiviy was dissatisfaction. This might explain on the base of steric constraints imposed by the catalyst framework. Thus, it might be for less steric constraints of 2a-c for blocking one iminium face from attacking of diene in the reaction transition state. In addition, the effectiveness of the hydroxyl non-covalent site on catalyst 2 to the enantioselectivity was not also clarified.
We examined the effectiveness of acrorein derivative 11 using superior catalyst 2b (Scheme 4). The reaction of diene 3b with dienophile 11 was carried out at room temperature in the presence of 30 mol% of catalyst 2b to give only the endo-DA adduct 12 and those chemical and optical yields were determined by converting to the alcohol 13. Unfortunately, catalyst 2b did not show satisfactory asymmetric catalytic activity to afford only the corresponding endo-DA adduct 12 (42% ee, 30%).
In conclusion, new chiral hydrazinoalcohol organocatalysts 2a-c were explored. The catalysts were prepared from the corresponding β-amino alcohols in three steps. The DA reaction of 1,2-dihydropyridine 3b with acrolein 8 using the explored catalyst 2b provided the corresponding DA adduct 9b in good enantioselectivity (67% ee) and excellent chemical yield (95%). Further optimizations of the structure of the catalyst 2 for increasing enantioselectivty in this reaction are now in progress.
EXPERIMENTAL
IR spectra were measured with PERKIN ELMER 1725X spectrophotometer. 1H-NMR spectra were recorded on JEOL JNM-GSX 400 spectrometers with TMS as an internal standard. MS were taken on Hitachi RMG-6MG and JEOL-JNM-DX 303 spectrometers. Optical rotations were measured with JASCO-DIP-370 digital polarimeter.
General procedure for the preparation of hydrazinoalcohol organocatalysts 2a-c
To a solution of amino alcohols 5a-c (2.0 mmol) in MeOH (16 mL) was added benzaldehyde (0.20 mL, 2.0 mmol) and the mixture was stirred for 30 min. The solution was then cooled to at -10 ˚C and NaBH4 (113.5 mg, 3.0 mmol) was added over 1h. The mixture was stirred for 22h at rt. Water (10 mL) was added, the aqueous layer was extracted with CH2Cl2 (2 x 30 mL) and organic phases were dried over MgSO4 and evaporated to give a crude products 6a-c. The residue was purified by column chromatography (SiO2, CHCl3) to give a pure products 6a-c (a: 478 mg, 63%, b: 677 mg, 86%, c: 331 mg, 46%). An aqueous solution of NaNO2 (276 mg, 4 mmol in 3 mL H2O) was added dropwise over 1 h at 70 ˚C to a solution of 6 and conc. HCl (1.0 mmol) in H2O (10 mL). The mixture was stirred at 70 ˚C for 1.5 h, cooled and extracted with Et2O (60 mL). The organic phases was washed H2O (10 mL), dried over MgSO4, and the solvent was evaporated under a reduced pressure. The residue was purified by column chromatography (SiO2, CHCl3) to give a pure product 7a-c (a: 327 mg, 80%, b: 237 mg, 56%, c: 291 mg, 81%). LiAlH4 (420 mg, 11 mmol) was slowly added under Ar to a cooled solution of 7a-c (1 mmol) in anhydrous Et2O (30 mL) keeping the temperature under -10 ˚C. The mixture was stirred two additional hours at this temperature, cooled to -78 ˚C, quenched with H2O (0.42 mL), 15% aq NaOH (0.42 mL) and H2O (1.26 mL) filtered by Celite® and evaporated to give white crystals of 2a-c (a: 294 mg, 72%, b: 268 mg, 68%, c: 187 mg, 50%).
(S)-2-(N-Benzylamino)-1,1,3-triphenylpropan-1-ol (6b): White crystal (AcOEt), mp 75-77 °C, [α] D22 -28.84 (c1.04, CHCl3). IR (KBr) cm-1: 697, 749, 1651, 3448. 1H-NMR (CD3OD) δ: 7.73 (d, J = 7.7 Hz, 2H), 7.65 (d, J = 7.7 Hz, 2H), 7.10-7.35 (m, 14H), 6.59 (d, J = 7.3 Hz, 2H), 4.86 (brs, 1H), 3.94 (dd, J = 10.6 Hz, 2.9 Hz, 1H), 2.92-3.02 (m, 3H), 2.35 (dd, J = 14.5 Hz, 10.6 Hz, 1H), 1.43 (brs, 1H). 13C-NMR(CD3OD) δ: 147.59, 145.0, 139.6, 139.3, 129.05, 128.68, 128.21, 128.18, 128.16, 128.03, 126.85, 126.65, 126.48, 126.45, 126.02, 125.65, 78.09, 65.68, 53.75, 37.63. Ms m/z: 394. HRMS (EI) calcd for (C28H27NO) : 393.2093, found: 393.2096.
(S)-2-(N-Benzylamino)-3,3-dimethyl-1,1-diphenylbutan-1-ol (6c): White crystal (ether), mp 117 °C, [α] D20 -98.57 (c 0.70, CHCl3). IR (KBr) cm-1: 708, 746, 1599, 1651, 3358. 1H-NMR (CD3OD) δ: 7.84 (dd, J = 8.5 Hz, 1.2 Hz, 2H), 7.70 (dd, J = 8.5 Hz, 1.2 Hz, 2H), 7.32 (t, J = 7.7 Hz, 2H), 7.09-7.23 (m, 7H), 6.88 (dd, J = 7.2 Hz, 1.9 Hz, 2H), 4.76 (s, 1H), 3.57 (d, J = 11.6 Hz, 1H), 3.53 (s, 1H), 3.26 (d, J = 11.1 Hz, 1H), 0.79 (s, 9H). 13C-NMR(CD3OD) δ:150.03, 144.89, 140.23, 128.31, 128.26, 127.80, 127.51, 127.08, 126.83, 126.39, 126.25, 126.19, 79.82, 72.72, 56.12, 37.55, 29.20. Ms m/z: 360 [M+H]+. HRMS (FAB+) calcd for (C25H29NO +H)+ : 360.2249, found: 360.2330.
(S)-2-(N-Benzyl-N-nitrosoamino)-1,1,2-triphenylethanol (7a): Amorphous, [α] D22 -574.74 (c1.94, CHCl3). IR (film) cm-1: 697, 751, 1599, 1658, 3489. 1H-NMR (CD3OD) δ: 7.36-7.41 (m, 3H), 7.06-7.25 (m, 15H), 6.89-6.91 (m, 2H), 5.72 (d, J = 14.0 Hz, 1H), 5.64 (s, 1H), 4.43 (s, 1H), 3.53 (d, J = 14.0 Hz, 1H). 13C-NMR(CD3OD) δ: 145.03, 143.19, 134.03, 132.96, 130.03, 129.56, 128.92, 128.53, 128.24, 128.18, 127.90, 127.79, 127.56, 126.98, 126.76, 126.15, 125.44, 80.44, 71.64, 47.47. Ms m/z: 409 [M+H]+. HRMS (FAB+) calcd for (C27H24N2O2 +H)+ : 409.1838, found: 409.1916.
(S)-2-(N-Benzyl-N-nitrosoamino)-1,1,3-triphenylpropan-1-ol (7b): Amorphous, [α] D22 +84.61 (c1.04, CHCl3). IR (film) cm-1: 697, 750, 1599, 1701, 3489. 1H-NMR (CD3OD) δ: 7.66 (d, J = 8.2 Hz, 2H), 7.40 (t, J = 7.7 Hz, 2H), 7.09-7.29 (m, 10H), 6.95-7.00 (m, 4H), 6.37 (d, J = 7.2 Hz, 2H), 5.29 (d, J = 10.6 Hz, 1H), 4.16 (d, J = 15.0 Hz, 1H), 3.93 (d, J = 15.0 Hz, 1H), 3.43 (dd, J = 14.5 Hz, 11.6 Hz, 1H), 3.22 (dd, J = 14.5 Hz, 2.9 Hz, 1H), 1.58 (s, 1H). 13C-NMR(CD3OD) δ: 144.31, 143.99, 137.59, 132.77, 129.06, 128.85, 128.66, 128.43, 128.29, 127.42, 127.28, 126.97, 126.75, 125.41, 125.38, 80.58, 71.68, 50.21, 36.77. Ms m/z: 423 [M+H]+. HRMS (FAB+) calcd for (C28H26N2O2 +H)+ : 423.1994, found: 423.2073.
(S)-2-(N-Benzyl-N-nitrosoamino)-3,3-dimethyl-1,1-diphenylbutan-1-ol (7c): White crystal (ether), mp 154-156 °C, [α] D20 +80.99 (c1.21, CHCl3). IR (KBr) cm-1: 694, 742, 1597, 1650, 3447. 1H-NMR (CD3OD) δ: 7.75 (d, J = 7.7 Hz, 2H), 7.39 (t, J = 8.2 Hz, 2H), 6.87-7.30 (m, 11H), 4.98 (d, J = 1.9 Hz, 1H), 4.68 (d, J = 14.5 Hz, 1H), 4.43 (s, 1H), 4.31 (d, J = 15.5 Hz, 1H), 0.88 (s, 9H). 13C-NMR(CD3OD) δ: 147.21, 145.05, 134.09, 128.92, 128.43, 128.36, 128.31, 128.03, 127.49, 126.62, 126.36, 125.33, 124.68, 82.57, 79.31, 56.14, 39.13, 30.82. Ms m/z: 389 [M+H]+. HRMS (FAB+) calcd for (C25H28N2O2 +H)+ : 389.2151, found: 389.2228.
(S)-2-(1-Benzylhydrazinyl)-1,1,2-triphenylethanol (2a): Pale yellow solid, mp 95-97 °C, [α] D20 -70.58 (c 1.19, CHCl3). IR (KBr) cm-1: 698, 744, 1588, 3334. 1H-NMR (CDCl3) δ: 7.69 (dd, J = 8.2 Hz, 1.0 Hz, 2H), 7.52 (dd, J = 8.2 Hz, 1.5 Hz, 2H), 6.69-7.37 (m, 16H), 4.63 (s, 1H), 3.92 (d, J = 13.5 Hz, 1H), 3.13 (d, J = 13.0 Hz, 1H), 2.93 (brs, 2H). 13C-NMR(CD3OD) δ: 148.96, 145.47, 136.92, 134.10, 131.17, 129.11, 128.89, 128.48, 128.45, 128.40, 127.77, 127.54, 127.52, 127.51, 127.37, 127.26, 126.64, 126.50, 125.99, 125.93, 125.71, 82.18, 71.51, 65.32. Ms m/z: 395 [M+H]+. HRMS (FAB+) calcd for (C28H28N2O +H)+ : 395.2045, found: 395.2130.
(S)-2-(1-Benzylhydrazinyl)-1,1,3-triphenylpropan-1-ol (2b): White crystal (ether), mp 79-81 °C, [α] D20 -76.63 (c 2.14, CHCl3). IR (KBr) cm-1: 697, 746, 1601, 1656, 3332. 1H-NMR (CDCl3) δ: 7.65 (m, 4H), 7.10-7.33 (m, 14H), 6.67 (m, 2H), 4.20 (dd, J = 9.2 Hz, 2.9 Hz, 1H), 3.72 (d, J = 14.0 Hz, 1H), 3.22 (dd, J = 15.0 Hz, 11.8 Hz, 1H), 3.11 (d, J = 13.5 Hz, 1H), 2.92-2.96 (m, 3H). 13C-NMR(CD3OD) δ: 148.98, 146.10, 141.77, 137.83, 129.10, 128.50, 128.46, 128.42, 128.36, 128.27, 128.20, 127.67, 127.22, 126.27, 126.11, 126.02, 125.98, 125.76, 125.38, 82.47, 70.70, 66.13, 30.53. Ms m/z: 409 [M+H]+. HRMS (FAB+) calcd for (C28H28N2O +H)+ : 409.2202, found: 409.2272.
(S)-2-(1-Benzylhydrazinyl)-3,3-dimethyl-1,1-diphenylbutan-1-ol (2c): White crystal (ether), mp 115 °C, [α] D20 +5.35 (c 0.56, CHCl3). IR (KBr) cm-1: 702, 746, 1604, 1656, 3326. 1H-NMR (CD3OD) δ: 7.80 (d, J = 7.7 Hz, 2H), 7.01-7.32 (m, 13H), 4.22 (d, J = 14.0 Hz, 1H), 3.92 (s, 1H), 3.58 (d, J = 14.0 Hz, 1H), 2.66 (brs, 2H), 1.00 (s, 9H). 13C-NMR(CD3OD) δ: 150.83, 147.98, 138.71, 128.26, 127.95, 127.88, 127.18, 126.01, 125.83, 125.71, 83.28, 75.78, 68.81, 40.51, 30.97. Ms m/z: 375 [M+H]+. HRMS (FAB+) calcd for (C25H30N2O +H)+ : 375.2358, found: 375.2433.
General procedure for the enantioselective Diels-Alder reaction of 3a,b with 8 or 11
A solution of catalysts 2a-c (0.03 mmol) and CF3CO2H (0.03 mmol) in MeCN/H2O (95/5 v/v, 0.5 mL) was added distillated acroleins 8 or 11 (0.3 mmol) and under argon. To the reaction solution, 1,2-dihydropyridines 3a or 3b (0.6 mmmol) was added, and stirred at rt or 0 ˚C for 40 h. The reaction was quenched by water. The aqueous layer was extracted with AcOEt and organic phases were dried over MgSO4. After the solution was evaporated, EtOH (1.0 mL) and NaBH4 (2.0 mg, 0.05 mmol) was added and the mixture was stirred at rt for 1 h. The reaction mixture was diluted with water and extracted with AcOEt. The combined organic extracts were washed with brine, dried over MgSO4, removed under reduced pressure to give a crude DA adduct 10a, 10b or 13. The residue was purified by preparative TLC (SiO2, n-hexane : AcOEt = 1 : 1) to afford the DA adduct 10a, 10b or 13 in quantitative yield. The enantiomeric excess (ee) was determined by HPLC [DAICEL Chiralcel AD-H, 0.5 mL/min, n-hexane : 2-propanol = 85 : 15, tr (major) = 21.64 min, tr (minor) = 23.09 min for endo-10a, DAICEL Chiralcel AS-H, 0.5mL/min, n-hexane : 2-propanol = 93 : 7, tr (major) = 43.94 min, tr (minor) = 54.67 min for endo-10b, AD-H, 0.5 mL/min, n-hexane : 2-propanol = 85 : 15, tr (major) = 37.50 min, tr (minor) = 40.02 min for endo-13].
References
1. (a) J. Seayad and B. List, Org. Biomel. Chem., 2005, 3, 719; CrossRef (b) P. I. Dalko and L. Moisan, Angew. Chem. Int. Ed., 2004, 43, 5138; CrossRef (c) W. Notz, F. Tanaka, and C. F. Barbas, III, Acc. Chem. Res., 2004, 37, 580; CrossRef (d) B. List, Synlett, 2001, 1675; CrossRef (e) H. Yang and R. G. Carter, Synlett, 2010, 2827. CrossRef
2. H. Nakano, K. Osone, M. Takeshita, E. Kwon, C. Seki, H. Matsuyama, N. Takano, and Y. Kohari, Chem. Commun., 2010, 46, 4827. CrossRef
3. K. A. Ahrendt, C. J. Borths, and D. W. C. MacMillan, J. Am. Chem. Soc., 2000, 122, 4243. CrossRef
4. (a) N. Takenaka, Y. Huang, and V. H. Rawal, Tetrahedron, 2002, 58, 8299; CrossRef (b) H. Nakano, N. Tsugawa, and R. Fujita, Tetrahedron Lett., 2005, 46, 5677; CrossRef (c) H. Nakano, N. Tsugawa, K. Takahashi, and Y. Okuyama, R. Fujita, Tetrahedron, 2006, 62, 10879. CrossRef
5. (a) M. E. Kuehne and I. Marko, in Syntheses of Vinblastine-type Alkaloids. The Alkaloids. Antitumor Bisindole Alkaloids from Catharanthus roseus (L.), ed. by A. Brossi and M. Suffness, Academic: San Diego, 1990, Vol. 37, pp. 77-131; CrossRef (b) P. Popik and P. Skolnick, in Pharmacology of Ibogaine and Ibogaine-related Alkaloids. The Alkaloids. Chemistry and Biology, ed. by G. A. Cordell, Academic: San Diego, 1999, Vol. 52, pp. 197-231; (c) S. D. Glick, I. M. Maisonneuve, and K. K. Szumlinski, in Mechanisms of Action of Ibogaine: Relevance to Putative Therapeutic Effects and Development of a Safer Iboga Alkaloid Congener. The Alkaloids, ed. by K. R. Alper, S. D. Glick, and G. A. Cordell, Academic: San Diego, 2001, Vol. 56, pp. 39-53; (d) G. Buchi, D. L. Coffen, K. Kocsis, P. E. Sonnet, and F. E. Ziegler, J. Am. Chem. Soc., 1996, 88, 3099; CrossRef (e) C. Marazano, M. LeGoff, J. Fourrey, and B. C. Das, J. Chem. Soc., Chem. Commun., 1981, 389; CrossRef (f) S. Raucher and B. L. Bray, J. Org. Chem., 1987, 109, 442; (g) M. T. Reding and T. Fukuyama, Org. Lett., 1999, 1, 973. CrossRef
6. (a) N. Satoh, T. Akiba, S. Yokoshima, and T. Fukuyama, Angew. Chem. Int. Ed., 2007, 46, 5734; CrossRef (b) N. Satoh, T. Akiba, S. Yokoshima, and T. Fukuyama, Tetrahedron, 2009, 65, 3239. CrossRef
7. F. Roussi, A. Chauveau, M. Bonin, L. Micouin, and H.-P. Husson, Synthesis, 2000, 8, 1170. CrossRef