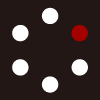
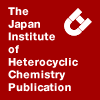
HETEROCYCLES
An International Journal for Reviews and Communications in Heterocyclic ChemistryWeb Edition ISSN: 1881-0942
Published online by The Japan Institute of Heterocyclic Chemistry
e-Journal
Full Text HTML
Received, 16th November, 2010, Accepted, 17th December, 2010, Published online, 24th December, 2010.
DOI: 10.3987/REV-10-687
■ Synthetic Routes towards Thiazolo[1,3,5]triazines (Review)
Anton V. Dolzhenko*
School of Pharmacy, Curtin University of Technology, GPO Box U1987, Perth, Western Australia 6845, Australia
Abstract
The present review summarizes information on the synthetic approaches to thiazolo[3,2-a][1,3,5]triazines and polyfused systems bearing this heterocyclic core since the first report on this structure in 1887. The methods allowing access to the heterocyclic systems comprising isomeric thiazolo[3,4-a][1,3,5]triazine scaffold are also included in the review. Data concerning potential applications of the thiazolo[1,3,5]triazines, particularly as biologically active agents are discussed.Dedicated to Professor Viktor E. Kolla with my best wishes on the occasion of his 85th birthday
CONTENTS
1. INTRODUCTION
2. SYNTHESIS OF THIAZOLO[3,2-a][1,3,5]TRIAZINES AND THEIR POLYFUSED ANALOGUES
2.1. Synthesis of thiazolo[3,2-a][1,3,5]triazines by annelation of the 1,3,5-triazine ring onto a thiazole scaffold.
2.1.1. Synthesis of thiazolo[3,2-a][1,3,5]triazines using Mannich condensation.
2.1.2. Synthesis of thiazolo[3,2-a][1,3,5]triazines using multicomponent reactions of thiazole derivatives with heterocumulenes.
2.1.3. Synthesis of thiazolo[3,2-a][1,3,5]triazines using other multicomponent reactions of 2-aminothiazoles and their derivatives.
2.1.4. Synthesis of thiazolo[3,2-a][1,3,5]triazines via reactions of 2-aminothiazoles with C-N-C triatomic synthons.
2.1.5. Synthesis of thiazolo[3,2-a][1,3,5]triazines via formal [4+2] cycloaddition.
2.1.6. Synthesis of thiazolo[3,2-a][1,3,5]triazines via 1,3,5-triazine ring annelation on 2-substituted thiazoles using one-carbon inserting reagents.
2.1.7. Synthesis of thiazolo[3,2-a][1,3,5]triazines via intramolecular cyclization of 2-substituted thiazoles with the formation of 1,3,5-triazine ring.
2.2. Synthesis of thiazolo[3,2-a][1,3,5]triazines by annelation of the thiazole ring onto a 1,3,5-triazine scaffold.
2.3. Synthesis of thiazolo[3,2-a][1,3,5]triazines via formation of the thiazole and 1,3,5-triazine ring.
3. HETEROCYCLIC SYSTEMS COMPRISING THIAZOLO[3,4-a][1,3,5]TRIAZINE CORE
4. CONCLUSION
5. REFERENCES
1. INTRODUCTION
In this review, we summarized existing methods of the synthesis of the compounds comprising thiazolo[3,2-a][1,3,5]triazine (A) and thiazolo[3,4-a][1,3,5]triazine (B) nuclei (Figure 1) as well as their biological activity and applications. Information on the preparation of related fused heterocyclic system (C) with 1,2-thiazole (isothiazole) instead of 1,3-thiazole ring is not available in the literature.
The synthesis of first thiazolo[3,2-a][1,3,5]triazine derivative was described by Rathke in 1887.2,3 He reported the reaction of thioammeline (1) with 1,2-dibromoethane that afforded 2 (Scheme 1). However, this work was not continued and it took more than 70 years for thiazolo[3,2-a][1,3,5]triazines to come back to the scene. Until now, a number of effective synthetic procedure have been developed for the preparation of diversely substituted thiazolo[3,2-a][1,3,5]triazines. They are discussed in Section 2 of this review.
During last decade, significant interest has been developed towards the biological activity investigation of compounds with the thiazolo[3,2-a][1,3,5]triazine heterocyclic core. The attempted herein systematization of the available synthetic methods for the preparation of these compounds aims to facilitate further investigation in this emerging field of research.
There no data on the synthesis of compounds possessing two-ring system B, thus Section 3 covers the preparation of more complex polyfused systems comprising thiazolo[3,4-a][1,3,5]triazine structure (B).
2. SYNTHESIS OF THIAZOLO[3,2-a][1,3,5]TRIAZINES AND THEIR POLYFUSED ANALOGUES
The general methods of synthesis of thiazolo[3,2-a][1,3,5]triazines can be categorized into: (1) annelation of the 1,3,5-triazine ring onto a thiazole scaffold, (2) annelation of the thiazole ring onto a 1,3,5-triazine scaffold and (3) formation of both rings in the same reaction.
2.1. Synthesis of thiazolo[3,2-a][1,3,5]triazines by annelation of the 1,3,5-triazine ring onto a thiazole scaffold.
The annelation of the 1,3,5-triazine ring onto a thiazole scaffold is the most explored and developed approach for the synthesis of thiazolo[3,2-a][1,3,5]triazines. It is further subdivided in this review on the basis of the reaction type and structure of the building blocks.
2.1.1. Synthesis of thiazolo[3,2-a][1,3,5]triazines using Mannich condensation.
The Mannich reaction of 2-aminothiazolin-4-one (3) and its 5-substituted derivatives with formaldehyde and variety of amines was extensively explored. The substitution at position 5 of 2-aminothiazolin-4-one (3) and nature of the amine used in the reaction were found to determine structure of the product. Thus, reaction of 3 with formaldehyde and methylamine or aromatic amines resulted in the formation of Mannich bases 5 (Scheme 2).4,5
However, when t-buthylamine or benzylamine were used in this reaction, hydroxymethylation at position 5 of 3 took place together with 1,3,5-triazine ring closure providing 6c and 6e.6 Additionally, spiro-fused compound 7 was isolated from the reaction of 3 with formaldehyde and t-buthylamine. The hydrolysis of 6c and 7 was investigated in details by Ramsh et al.7 The aminomethylation of amidine moiety of 4 afforded 3-alkyl and 3-aralkyl substituted 6 (Scheme 2).8 Compounds of this type, e.g. 6d were among leads for the development of agents selectively targeting Fanconi anemia pathway-deficient tumors.9
The pathway of the reaction of 5-alkyl substituted 2-aminothiazolin-4-ones (8) with formaldehyde and amines was found to depend on the amine structure and the substitution at position 5 of 8. In the reaction with β-naphthylamine, the products of the aminomethylation (9) were obtained exclusively, while their hydroxymethylated analogues (10) were formed using t-buthylamine (Scheme 3).4,6,8 The reaction of methyl and ethyl substituted 8 with formaldehyde and aniline provided 10b and 9b, respectively.6
Similar reaction of 5-arylidene substituted 2-aminothiazolin-4-ones (11) was used for the preparation of a series of corresponding Mannich bases 12 (Scheme 4).4,6,10 One of this type of compounds, CCT196755, was identified as an inhibitor of phospholipase C-γ with IC50 = 15 M in the functional cell based assay.11
Analogously, 13 reacted with formaldehyde and methylamine to produce 14 (Scheme 5).8 These compounds were also found to be potential agents against Fanconi anemia pathway-deficient tumors.9
2.1.2. Synthesis of thiazolo[3,2-a][1,3,5]triazines using multicomponent reactions of thiazole derivatives with heterocumulenes.
The pathways of multicomponent reactions of thiazoles with heterocumulenes were found to involve the cycloaddition, which may be accompanied by other processes depending on structure of the substrate and reaction conditions. The most common heterocumulenes used in the reaction were isocyanates.
Several cycloaddition reactions of 2-substituted thiazolines with isocyanic acid and its derivatives were used for the synthesis of thiazolo[3,2-a][1,3,5]triazin-2,4-diones. Thus, the reaction of thiazolines 15 with two molecules of isocyanic acid resulted in the annelation of 2,4-dioxotriazine ring affording 16 (Scheme 6).12 In the biological screening, 16 showed activity against Walker’s carcinoma 256 when tested in rats.
In a similar way, adducts 17 were formed upon treatment of 15 with aryl isocyanates (Scheme 7).13
2-Aminothiazoline (18) reacted with excess of phenyl isothiocyanate affording thiazolo[3,2-a][1,3,5]triazine 20, which was rationalized to form via the triazine ring closure of intermediate 1:2 adduct 19 with subsequent elimination of hydrogen sulfide (Scheme 8).14 The structure of 20 was unequivocally established using X-ray crystallography.15 An attempt to obtain similar product using 1-naphthyl isothiocyanate instead of phenyl isothiocyanate was unsuccessful.
Another thiazoline derivative, formamidine 21, reacted with aryl isocyanates in mild conditions via the [4+2] cycloaddition (vide infra Scheme 58).16 However, heating 21 with excess of aryl isocyanates in benzene afforded 1:2 adduct 22 (Scheme 9).16 Using higher temperature and excess of aryl isocyanates resulted in the formation of 23. In this case, the synthetic pathway might be rationalized by [4+2] cycloaddition of presumable intermediate - thiazolin-2-yl isocyanate (24) and aryl isocyanate molecule.
It was shown in the same work,16 that formamidine 25 reacted with aryl isocyanates affording the formation of 3-arylthiazolo[3,2-a][1,3,5]triazin-2,4-diones (26) in all of the reaction conditions applied (Scheme 10).
Similarly, benzofused analogues 28 were obtained from 27 and aryl isocyanates (Scheme 11).16
Instead of expected [4+2] cycloaddition (vide infra Scheme 60), heating 2-(4-dimethylaminobenzylidenamino)benzothiazole (29a) with phenyl isocyanate or phenyl isothiocyanate in inert solvent resulted in the formation of the corresponding 2-benzothiazolyl isocyanate or 2-benzothiazolyl isothiocyanate (30) (Scheme 12).17 In case of the excess of phenyl isocyanate, the cycloaddition afforded 28a. If no excess of phenyl isocyanate was available, 2-benzothiazolyl isocyanate as well as its thio-analogue (30) dimerized to 31 (Scheme 12). The electronodonor properties of the dimethylamino group were proposed to be responsible for the reaction pathway. However, the basicity of this group seems to be a more important factor determining the product structure.
3-Arylthiazolo[3,2-a][1,3,5]triazin-2,4-diones (26 and 28) were also prepared by heating corresponding acetamides 32 and 33 with aryl isocyanates in pyridine (Schemes 13 and 14).18
2-Aminothiazole 34 was reported19 to react with aryl isocyanates in pyridine affording the formation of 3-arylthiazolo[3,2-a][1,3,5]triazin-2,4-diones (35) (Scheme 15).
Benzofused analogues 37 were synthesized from 2-aminobenzothiazoles (36) (Scheme 16).20 The compounds 37 underwent biological screening against Mycobacterium tuberculosis H37RV. The most active compound (37, R1 = OEt, R2 = Ph) showed inhibition of M. tuberculosis at 0.12 μg/ml.
Another series of tricyclic thiazolo[3,2-a][1,3,5]triazines (39), which possessed anthelmintic activity against Nematospiroides dubius, was prepared from amines 38 using similar method (Scheme 17).19
The reactions of 2-thiazolyliminotriphenylphosphorane (40) with various heterocumulenes were studied in details by Bödeker et al.21,22 Phenyl isocyanate was found to react with 40 affording two thiazolo[3,2-a][1,3,5]triazines 42 and 43 (Scheme 18).21 The formation of both products was suggested to proceed via intermediate carbodiimide 41, which depending on the reaction condition undergoes either [4+2] cycloaddition with another molecule of phenyl isocyanate providing 42 or dimerization to 43. Using excess of phenyl isocyanate and mild reaction conditions allowed preparation of 42 in good yield, whereas prolonged heating equimolar quantities of 40 and phenyl isocyanate resulted in the isolation of 43, exclusively.
The reaction of 40 with diphenylcarbodiimide provided 44 (Scheme 19).22 The proposed mechanism involved the initial formation of intermediate 41 followed by the cycloaddition of another diphenylcarbodiimide molecule. The low yield of 44 was associated with the polymerization observed during the reaction.
The treatment of 2-thiazolyliminotriphenylphosphorane (40) with carbon disulfide afforded the formation of 47 (Scheme 20).21 It was proposed that two molecules of 40 and one molecule of carbon disulfide via intermediacy of 2-thiazolyl isothiocyanate (45) formed di(2-thiazolyl)carbodiimide (46), which underwent subsequent dimerization providing 47. Similarly, benzofused analogue 48 was also prepared, but with lower yield.
2.1.3. Synthesis of thiazolo[3,2-a][1,3,5]triazines using other multicomponent reactions of 2-aminothiazoles and their derivatives.
The pseudo-four-component reaction of 2-aminothiazole (49) with phosgene afforded 3-(2-thiazolyl)thiazolo[3,2-a][1,3,5]triazin-2,4-dione (51) (Scheme 21).23 The mechanism of the reaction was based on the dimerization of in situ formed 2-thiazolyl isocyanate (50). Analogous benzofused dimer 31a was prepared in the same way with the 21% yield (cf. Scheme 12).
When 2-aminobenzothiazoles (36) were heated with excess of dimethylcyanamide, fused triazines 52 were formed instead of expected guanidines (Scheme 22).24 Two molecules of 36 and two molecules of dimethylcyanamide are involved in the condensation.
Benzothiazoazacyanines (bis-benzothiazolo[3,2-a:3’,2’-d][1,3,5]triazin-12-ium iodides) 53 were conveniently prepared from 2-aminobenzothiazoles (36) by the reaction with diiodomethane at high temperature (Scheme 23).25,26 Recently, the binding of 53 to a variety of nucleic acid sequences was investigated. With poor affinity to the duplex DNA strands, compounds 53 exhibited tight binding to G-quadruplex27 and poly(A).28
13-(4-Methoxyphenyl)-13H-bis-benzothiazolo[3,2-a:3’,2’-d][1,3,5]triazin-12-ium bromides (55), structurally related to 53, were synthesized by treatment of 2-aminobenzothiazoles (36) with 54 (Scheme 24).29 When equimolar mixture of 36 with R = Me and R = MeO was used in the reaction, the product with unsymmetrical substitution was isolated in the yield of 22% together with about 10% of each symmetrical compounds 55b and 55c.
The three-component solvent-free reaction of 2-amino-4-arylthiazoles (56) with aromatic aldehydes and ammonium thiocyanate was reported30 to afford thiazolo[3,2-a][1,3,5]triazines 57 (Scheme 25). Using microwave irradiation substantially improved yields of 57 and shortened the reaction time. The products (57) were further effectively glycosylated at N-3.
Another three-component solvent-free synthesis of thiazolo[3,2-a][1,3,5]triazines 59 was performed using thiazole Schiff bases 58, ammonium acetate and aromatic aldehydes (Scheme 26).31 This methodology was also extended to C-nucleosides 59 by application of aldoses instead of benzaldehydes.32 In all cases, microwave irradiation was more efficient than conventional heating due to shorter reaction time and higher yields of 59. Moreover, under the microwave irradiation conditions, the reaction diastereoselectivity was dramatically improved from 51-60% to 93-99%.
2.1.4. Synthesis of thiazolo[3,2-a][1,3,5]triazines via reactions of 2-aminothiazoles with C-N-C triatomic synthons.
The large number of readily available 2-aminothiazoles makes them popular as building blocks for the construction of thiazolo[3,2-a][1,3,5]triazines. A variety of the -C-N-C- triatomic synthons were applied to build properly substituted 1,3,5-triazine ring on the 2-aminothiazole scaffold. However, regiochemistry of the ring closure was not always discussed and the structure assignments were often uncertain.
The isocyanates and their analogues, bearing at the nitrogen atom another reactive electrophilic group, represent one type of the commonly used -C-N-C- triatomic synthons. The nature of the electrophilic group attached to the isocyanate determines the substitution on the triazine ring as well as affects the regioselectivity of the reaction.
Two alternative pathways are possible for the reactions of isocyanates with 2-aminothiazoles depending on the site of electrophilic attack of the isocyanate group. The reaction of 2-aminothiazole (49) with isocyanates 60 in the presence of base might theoretically proceed via addition of isocyanate group to exo- or endocyclic nitrogen atom thus resulting in the formation of two regioisomeric products 61 and 62, respectively (Scheme 27). Initially, this reaction was reported33,34 to afford 3,4-dihydrothiazolo[3,2-a][1,3,5]triazin-2-ones (61), but no evidence were provided in support of the product identity. Later, structure 62 was assigned for the products of similar reactions on the basis of the chemical shift of the CF3 signal in the 19F NMR spectra.35 These data also seem to be insufficient for unambiguous structure assignments.
The product of the reaction of 2-aminothiazole (49) with l-hydroxy-2,2,2-trichloroethyl isocyanate (63) was reported36 as similar to 61 4-trichloromethyl-3,4-dihydrothiazolo[3,2-a][1,3,5]triazin-2-one (64) (Scheme 28). The regioisomeric structure was not discussed. The hydroxyl of 63 acted as a leaving group instead of chloride in 60.
The benzofused analogue of 64 – tricyclic structure 66 was obtained from 2-aminobenzothiazole (36) and l,2,2,2-tetrachloroethyl isocyanate (65) (Scheme 29).37
Two isomeric structures 2-methoxythiazolo[3,2-a][1,3,5]triazin-4-one (68) and 2-methoxy- thiazolo[3,2-a][1,3,5]triazin-4-one (69) were proposed for the product of the reaction of 2-aminothiazole (49) with methoxydifluoromethyl isocyanate (67) (Scheme 30).38
The chemical shift of the CF3 signal in the 19F NMR spectra was used as an evidence supporting formation of thiazolo[3,2-a][1,3,5]triazin-4-ones 71 in similar reaction of 49 with 1,1-dichloro substituted alkyl isocyanates (70) (Scheme 31).35
The reaction of 2-aminothiazole (49) with phenoxycarbonyl isocyanate (72) was reported39,40 to produce thiazolo[3,2-a][1,3,5]triazin-2,4-dione (73), which was used for the preparation of potent 5-HT2-receptor antagonists, e.g. 74 (Scheme 32).39-41
2-Aminothiazoles with alkylated amino group (75) reacted with 72 affording mesoionic thiazolo[3,2-a][1,3,5]triazin-2,4-diones 76 (Scheme 33).42
Gotthardt and Blum43 reported the synthesis of benzofused mesoionic thiazolo[3,2-a][1,3,5]triazin-2,4-dione 78 by treatment of amine 77 with phenoxycarbonyl isocyanate (72) (Scheme 34). The reactivity of 78 was also investigated.
Two alternative pathways were reported for the reactions of N-benzoyl isothiocyanate (79, R = Ph) with 2-aminothiazoline (18) (Scheme 35). Klayman and Woods44 obtained 80 and confirmed the regiochemistry of the reaction by spectral data and chemical properties of the product. Together with 80 (10%), two other products i.e. N-benzoyl-N’-(2-thiazolin-2-yl)thiourea (28%) and thiocyanate of 2-benzamido-2-thiazoline (14%) were isolated from the reaction. Later, another structure 81 was assigned for the product of the reaction of 18 with N-aroyl isothiocyanates (79) formed in situ from the corresponding acid chloride and ammonium thiocyanate.45 The regioisomeric structures 80 were not considered in the paper.45
Barnikow and Bödeker 46 explored the reaction of 2-aminothiazole (49) with N-benzoyl isothiocyanate (79) (Scheme 36). They reported formation of 2-phenylthiazolo[3,2-a][1,3,5]triazin-4-thione (82b) as a main product (22%) together with N-benzoyl-N’-(2-thiazolyl)thiourea (19%) and 2-benzoylaminothiazole (0.5%). The report47 on the isolation of regioisomeric 4-phenylthiazolo[3,2-a][1,3,5]triazin-2-thione (83) seems to be erroneous as the X-ray crystallography data48 for the similarly obtained product supported the structure of 2-phenylthiazolo[3,2-a][1,3,5]triazin-4-thione (82b). Analogously, 2-propyl substituted compound 82a was prepared and its molecular and crystal structure were described.49 A series of 2-aryl substituted thiazolo[3,2-a][1,3,5]triazin-4-thiones (82) was synthesized by Saeed et al.50 with surprisingly high yields. The X-ray crystallography data for 82d were also reported.
N-Aryl benzimidoyl isothiocyanates 84 were reported to react with 2-aminothiazole (49) similarly to 79 providing 2-arylthiazolo[3,2-a][1,3,5]triazin-4-thiones (82) (Scheme 37).51 The addition of 84 to anilines, formed in the reaction after nucleophilic substitution of the imidoyl group, resulted in the formation of thioureas 85 as side products.
Analogously, 2-phenylthiazolo[3,2-a][1,3,5]triazin-4-thione (82b) was prepared by treatment of 2-aminothiazole (49) with bis-imidoyl isothiocyanate 86 (Scheme 38).52
2-Aminothiazoline (18) was quantitatively converted to 88 via the reaction with ethoxycarbonyl isothiocyanate (87) (Scheme 39). Initially, the regioisomeric structure 89 was assigned to the product,53 but the detail structure investigations by Klayman and Woods54 and crystallographic information55 proved that isothiocyanate group of 87 attacked endocyclic nitrogen atom of 2-aminothiazoline (18), affording 88 after the triazine ring closure.
The steric effect of the gem-dimethyl substitution at carbon atom adjacent to the endocyclic nitrogen of thiazoline ring was explored.54 The treatment of 2-amino-4,4-dimethylthiazoline (90) with 87 afforded the formation of 4-thioxothiazolo[3,2-a][1,3,5]triazin-2-one 91 as a sole regioisomer (Scheme 40). However, presence of the base was required for the reaction to proceed.
The reaction of 2-aminothiazoles (92) with ethoxycarbonyl isothiocyanate (87) was studied in details by Nagano et al.56-58 The effects of substitutions in the thiazole ring on the product yields and composition were explored.56 The formation of 4-thioxothiazolo[3,2-a][1,3,5]triazin-2-ones 93 was observed when 5-substituted 2-aminothiazoles (92) with pKa above 5 were treated with 87 (Scheme 41). However, the reaction lacked regioselectivity and was only fairly chemoselective. Together with 93, three other products, namely N-ethoxycarbonyl-N’-(2-thiazolyl)thioureas (94), N-(2-thiazolyl)urethanes (95) and N-(ethoxythiocarbonyl)urethane (96) were isolated from the reaction mixtures. In case of 2-aminothiazoles with the pKa below 5, no thiazolo[3,2-a][1,3,5]triazines were isolated from the reaction. Regardless the pKa value, 4-substituted 2-aminothiazoles in the reaction with 87 also did not afford thiazolo[3,2-a][1,3,5]triazines. Changing the ethoxy group in 87 to the methoxy one improved yield of 93a (R = H) from 29% to 41%.57 The higher alkoxy group (n-PrO, i-PrO, n-BuO and i-BuO) decreased yields of 93a to 19-26%. It was found that on heating, 94a (R = H) can undergo cyclization to 2-thioxo-thiazolo[3,2-a][1,3,5]triazin-4-one (98) (vide infra Scheme 83).57 Compounds 93 were claimed as potential bactericides and fungicides for agricultural use.58
Two regioisomeric thiazolo[3,2-a][1,3,5]triazines 93a and 98 were isolated from the reaction of 2-aminothiazole (49) with phenoxycarbonyl isothiocyanate (97) (Scheme 42).57 The formation of another side product, phenyl N-(2-thiazolyl)carbamate (99) was also mentioned.
2-Aminothiazoles with alkylated amino group (75) were shown to behave differently in the analogous reactions. The formation of regioisomeric mesoionic thiazolo[3,2-a][1,3,5]triazines 100 and 101 was observed when 75 reacted with ethoxycarbonyl isothiocyanate (87) and phenoxycarbonyl isothiocyanate (97), respectively (Scheme 43).42 The structures 100 and 101 were assigned on the basis of their further chemical transformations.
2-Arylaminothiazolo[3,2-a][1,3,5]triazin-4-thiones (103) were synthesized from 2-aminothiazole (49) and formamidinoyl isothiocyanates 102 (Scheme 44).59 The products of the addition of 102 to the amino group of 49, guanylthioureas 104 were also isolated from the reaction. Using analogues of 102 with piperidino or diethylamino substituents instead of morpholino moiety was reported to give the same products (103) of the triazine ring annelation.
It was reported,60 that N-(1-hydroxyimino-2-oxopropyl)pyridinium chloride (105) can be used as a stable and convenient substitute for acetyl isocyanate. The dehydrochlorination of salt 105 provided 106, which subsequently rearranged to the active complex of pyridine with acetyl isocyanate (107) (Scheme 45). The traces of 2-methylthiazolo[3,2-a][1,3,5]triazin-4-one (108) were detected in the reaction of 105 with 2-aminothiazole (49) in chloroform. Using 49 absorbed on SiO2 (1:2) allowed isolation of 108 with the 11% yield. Changing the 2-aminothiazole (49) – SiO2 ratio to 1:4 improved yield of 2-methylthiazolo[3,2-a][1,3,5]triazin-4-one (108) to 22%. Additionally, N-acetyl-N’-(2-thiazolyl)thiourea (109) was isolated from the reaction (Scheme 45).
Vovk and Dorokhov61 found that reaction of 2-aminothiazole (49) with carbodiimide 110, structurally related to isocyanates 60, in mild conditions afforded thiazolo[3,2-a][1,3,5]triazine 111 with trifluoromethyl group at the quaternary C-2 atom (Scheme 46).
Thiazolo[3,2-a][1,3,5]triazin-4-ones 113 (analogues of 62), substituted with two geminal trifluoromethyl groups, were prepared by treatment of 2-aminothiazoles (92) with hexafluoroacetone ethoxycarbonylimine (112) in DMF under TsOH catalysis (Scheme 47).62 The reaction of 2-aminothiazoline (18) with imine 112 in the same conditions afforded 114 with the 71% yield.
Similarly, benzofused analogues 116 were prepared from 2-aminobenzothiazoles 115 and imine 112 (Scheme 48).62 Partially saturated 117 was also obtained with the 66% yield using this method.
Acylimines of hexafluoroacetone (118) were reported63 to react with 2-aminothiazoline (18) with formation of 4-substituted 2,2-bis(trifluoromethyl)-6,7-dihydrothiazolo[3,2-a][1,3,5]triazines (119)
(Scheme 49). The same reaction was used for the synthesis of 120.
Diphenyl iminodicarboxylate (121) was found to be a convenient alternative for relatively unstable phenoxycarbonyl isocyanate (72) (vide supra Scheme 32). The reaction of 2-aminothiazoline (18) with 121 in mild conditions resulted in the formation of 6,7-dihydrothiazolo[3,2-a][1,3,5]triazin-2,4-dione (122) with good yield (Scheme 50).64 The reaction of 2-aminothiazole (49) with 121 required heating in dioxane under reflux for 4.5 h to give thiazolo[3,2-a][1,3,5]triazin-2,4-dione (73) with the 75% yield.
Heating 2-aminothiazoline (18) with N-ethoxycarbonyl thiobenzamides (123) in ethanol was reported65 to afford 2-aryl-6,7-dihydrothiazolo[3,2-a][1,3,5]triazin-4-ones (124) (Scheme 51). The conversion of 124a to its thiocarbonyl analogue 80 was carried out to confirm regioselectivity of the reaction.
Using carbamoyl chloride 126 was claimed66 to be effective for the 1,3,5-triazine ring annelation on 2-amino-4-methylthiazole (125) (Scheme 52). However, the question on the structure of products (127 or 128) remained open. Similarly, when 2-aminobenzothiazole (36) was reacted with 126 (R = Ph) on heating in diethyl ether for 1 h, either 129 or 130 were proposed as products.
4-Dimethylamino-2-phenylthiazolo[3,2-a][1,3,5]triazinium perchlorate (132) was obtained by heating of 2-aminothiazole (49) with salt 131 in acetonitrile (Scheme 53).67
The reaction of 2-aminothiazole (49) with cyclic iminium salt 133 was reported68 to afford thiazolo[3,2-a][1,3,5]triazinium iodide 134 (Scheme 54). When 4-substituted 2-aminothiazoles were used in the reaction, the triazine ring was not formed and only nucleophilic addition or substitution at the methyl thioimidate group of 133 and analogues occurred due to attack of the amino group of 2-aminothiazoles.
2-Amino-4-phenylimino-1,3,5-triazino[2,1-b]benzothiazole (136a) was prepared via reaction of 2-aminobenzothiazole (36) with sodium salt of N-cyano-N’-phenyl thiourea (135) (Scheme 55).69 A number of substituted analogues of 136, claimed to possess immuno-regulant activity, were synthesized by this manner or using N-cyano-N’-phenyl-S-methyl isothiourea instead of 135. However, low yields of the product, long reaction time and using toxic mercuric chloride were apparent limitations of the method.
Heating 2-aminobenzothiazole (36) with N-(chloromethyl)benzimidoyl chloride (137) was reported70 to afford 2-phenyl-4H-1,3,5-triazino[2,1-b]benzothiazole (138) (Scheme 56). The assignment of structure 138 vs. its possible regioisomer was supported by the 13C NMR spectral data.
The reaction of 2-aminobenzothiazole (36) with N,N-bis(chloromethyl)acetamide (139) proceeded via double alkylation of endo- and exocyclic nitrogen atoms of 36, thus resulting in the formation of 3-acetyl-3,4-dihydro-2H-1,3,5-triazino[2,1-b]benzothiazole (140) (Scheme 57).71
2.1.5. Synthesis of thiazolo[3,2-a][1,3,5]triazines via formal [4+2] cycloaddition.
Commonly, this strategy was realized in the reactions of hetero-1,3-dienes - aminothiazole derivatives as 4π-component with isocyanates and their analogues (2π-dienophiles). Thus, formamidine 21 upon treatment with aryl isocyanates in the mild conditions underwent [4+2] cycloaddition with the formation of 141 (Scheme 58).16 The reaction was found to be exothermic and very sensitive to the conditions. Changing ratio of the reagents and temperature substantially affected the reaction pathway and composition of the products (cf. Scheme 9).
The cycloaddition of phenyl isothiocyanate to Schiff bases 58 was reported72,73 to afford thiazolo[3,2-a][1,3,5]triazin-4-thiones 142 (Scheme 59).
Heating 2-(arylmethyleneamino)benzothiazoles (29) with phenyl isocyanate or phenyl isothiocyanate in toluene allowed the preparation of 143 (Scheme 60).74 The reaction pathway was found17 to strongly depend on the nature of the substituent R (cf. Scheme 12).
Similarly, when Schiff bases 144 reacted with aryl isothiocyanates, tricyclic structures 145 were formed (Scheme 61).75
The reaction of 2-(benzylidenamino)benzothiazoles (146) with ammonium thiocyanate resulted in the annulation of the triazine ring affording 147 (Scheme 62).76,77 Using microwave irradiation was reported77 to shorten the reaction time and improve yields. The antibacterial activity of 147 was evaluated.77
It was reported78 that 2-(4-methyl)thiazolyl isothiocyanate (148) reacted with Schiff bases and aryl isocyanates providing thiazolo[3,2-a][1,3,5]triazin-2-thiones 149 and 150, respectively (Scheme 63). Despite strong tendency to dimerization of 148, dimer 151 (a side product in the synthesis of 148) was not isolated from the reactions. Interestingly, no reaction was observed when 2-(4-phenyl)thiazolyl isothiocyanate was treated with aldimines or isocyanates even on heating.
The cyclodimerization of isocyanate 153, derived from hydrazide 152, resulted in the formation of thiazolo[3,2-a][1,3,5]triazin-2,4-dione 154 (Scheme 64).79
It was found that 3-phenylthiazolo[3,2-a][1,3,5]triazin-2,4-dione (26a) on heating lost phenyl isocyanate and form 51 as a product of the cyclodimerization of 2-thiazolyl isocyanate (50) (Scheme 65).23 Analogously, thermolysis of 28a resulted in the formation of 31a (cf. Schemes 12 and 21).
An example of the cyclodimerization of Schiff base 29b was reported by Abdel-Rahman.74 On prolonged heating in xylene, 29b afforded cycloadduct 155 (Scheme 66).
2.1.6. Synthesis of thiazolo[3,2-a][1,3,5]triazines via 1,3,5-triazine ring annelation on 2-substituted thiazoles using one-carbon inserting reagents.
George and Tahilramani80 reported synthesis of 2-phenylthiazolo[3,2-a][1,3,5]triazin-4-one (157) via inserting the carbonyl group by treatment of N-(thiazol-2-yl)benzamidine (156) with phosgene (Scheme 67). From the reaction of 156 with thiophosgene, 157 was isolated instead of the thiocarbonylation product (82b), which presumably underwent hydrolysis under the work-up conditions.
Similarly, benzofused analogue 130 was synthesized from N-(benzothiazol-2-yl)benzamidine (158) and phosgene (Scheme 68).80
3-Cyclohexylthiazolo[3,2-a][1,3,5]triazine-2,4-dione (160) was prepared from urea 159 by treatment with phosgene in the presence of base (Scheme 69).81 The product (160) was claimed to possess fungicidal properties.
Similarly, thiazolidine substituted ureas 161 were converted to 162 by the treatment with phosgene or diphosgene (Scheme 70).81-83 Compounds 162 were found to be useful as fungicides in agriculture.
Tetracyclic system 164 comprising thiazolo[3,2-a][1,3,5]triazin-4-one and benzimidazole was also prepared from 2-(thiazol-2-yl)benzimidazole (163) using phosgene as a one-carbon inserting reagent (Scheme 71).84
A variety of electrophilic reagents were adopted as one-carbon inserting reagents for the 1,3,5-triazine ring formation on the scaffold of thiazolyl and benzothiazolyl substituted guanidines. The reaction of 2-thiazolyl guanidines 165 with ethyl chloroacetate in the presence of sodium methylate was reported85 to afford thiazolo[3,2-a][1,3,5]triazine 166 (Scheme 72). In similar conditions, diethyl oxalate with 165 produced a mixture of methyl esters 167 and corresponding acids 168.
The analogous reaction of 2-benzothiazolylguanidine (169a) in with ethyl chloroacetate was applied for the synthesis of tricyclic 170 (Scheme 73).85 The triazine ring closure of 169a with diethyl oxalate resulted in the formation of mixture of 171 and 172.
Kreutzberger86 reported the synthesis of 1,3,5-triazino[2,1-b]benzothiazol-2-imine (173) from
2-benzothiazolyl guanidine (169a) and ethyl formate (Scheme 74). The yield of 173 was improved by the replacement of ethyl formate with 1,3,5-triazine as one-carbon inserting reagent.
The triazine ring closure with introduction of carbonyl group was achieved by treatment of 2-benzothiazolyl guanidines (169) with diethyl azodicarboxylate87 or phenyl and tosyl isocyanates88,89 (Scheme 75). The microwave irradiation was found to promote the reaction of 169 with phenyl isocyanate.89
Depending on the conditions applied, two types of the heterocyclization products were isolated from the reaction of 169 with phenyl isothiocyanate (Scheme 76). Heating 169 with phenyl isothiocyanate in pyridine afforded ring closure thiocarbonylation product 175.88 Under microwave irradiation, 2-amino-4-phenylimino-1,3,5-triazino[2,1-b]benzothiazoles (136) were formed in the similar reaction.89
The synthesis of 136a was also carried out via the cyclization of 2-benzothiazolyl guanidine (169) with phenyl isocyanide dichloride, but the yield was very low (Scheme 77).69
1,3,5-Triazino[2,1-b]benzothiazoles 177 were prepared form guanidines 176 by subsequent treatment with aryl isothiocyanate in the presence of sodium hydride followed by methyl iodide (Scheme 78).69
2.1.7. Synthesis of thiazolo[3,2-a][1,3,5]triazines via intramolecular cyclization of 2-substituted thiazoles with the formation of 1,3,5-triazine ring.
Etienne and Bonte90 found that in aqueous acetic acid, 2-thiazolyl substituted biuret 178 underwent intramolecular cyclization with methylamine elimination affording 3-methylthiazolo[3,2-a][1,3,5]triazin-2,4-dione (179) (Scheme 79).
N-Acyl-N’-(2-thiazolyl)thioureas, prepared from 2-aminothiazoles and N-acyl isothiocyanates were used for the synthesis of a variety of substituted thiazolo[3,2-a][1,3,5]triazin-2-thiones. Thus, treatment N-cinnamoyl-N’-(2-thiazolyl)thiourea 180 with sodium ethoxide resulted in the 1,3,5-triazine ring annelation with the formation of 181 (Scheme 80).91
Interestingly, structurally related thiourea 182 (R = Ph-CH=CH) with 4-(3-coumaryl) substituent on the thiazole ring reacted differently in the similar conditions affording cycloaddition product 183 (Scheme 81).92 However, heating N-benzoyl analogue 182 (R = Ph) in n-butanol was reported92 to produce thiazolo[3,2-a][1,3,5]triazin-2-thione 184.
A number of thiazolo[3,2-a][1,3,5]triazin-2-thiones (185) were prepared via this type of cyclocondensation by heating of corresponding N-acyl-N’-(2-thiazolyl)thioureas with alkali,93 phosphoryl chloride94 or phosphoryl chloride/phosphorus pentachloride mixture.95,96 The biological activity (antiviral,95 antibacterial94 and antifungal96) of 185 was also evaluated. However, lack of an appropriate structural characterization and controversy of the reported experimental data significantly decrease importance of the works.
Heating N-aroyl-N’-(2-benzothiazolyl)thioureas 186 in butanol resulted in the 1,3,5-triazine ring closure with the formation of 4-aryl-1,3,5-triazino[2,1-b]benzothiazol-2-thiones 187 (Scheme 82).97 The biological evaluation of 187 revealed anticonvulsant properties of the compounds in the maximum electroshock test in mice without signs of neurotoxicity or hepatotoxicity.
N-Ethoxycarbonyl-N’-(2-thiazolyl)thioureas (94), which were among products of the reaction of 2-aminothiazoles (92) with ethoxycarbonyl isothiocyanate (84) (vide supra Scheme 41), underwent thermal cyclocondensation affording 2-thioxothiazolo[3,2-a][1,3,5]triazin-4-ones 98 (Scheme 83).57,58 The cyclization of N-ethoxycarbonyl-N’-(2-thiazolyl)thioureas (94) was also successfully induced by alkali.98-100
From the reaction of N-ethoxycarbonyl-N’-(2-thiazolyl)thioureas (94) with alkyl halides in basic conditions, small quantities of thiazolo[3,2-a][1,3,5]triazin-4-ones 188 were isolated along with other alkylated products 189 and 190 (Scheme 84).101
In cases of alkylation of the thioureas substituted in position 4 of the thiazole ring as well as N-ethoxycarbonyl-N’-(5-bromothiazol-2-yl)thiourea, no formation of thiazolo[3,2-a][1,3,5]triazines was observed. In the presence of base or on heating, the major products of the reactions, isothioureas 190, were able to undergo cyclocondensation affording 188 with good yields (Scheme 85).101 Other alkoxycarbonyl analogues of 94 and corresponding 190 reacted in the same way.
The reaction of N-ethoxycarbonyl-N’-(2-benzothiazolyl)thiourea with methyl iodide in basic conditions did not result in the 1,3,5-triazine ring closure. However, the major product (79%) of the reaction, compound 191, can be easily cyclized to 4-methylthio-1,3,5-triazino[2,1-b]benzothiazol-4-one (192) upon heating (Scheme 86).101
The heterocyclization of 193, which was isolated (46% yield) from the reaction of 2-benzothiazolyl guanidines (169) with diethyl azodicarboxylate in diethyl ether at room temperature, afforded 174a (Scheme 87).87 The synthesis of 174a without isolation of intermediate 193 was also reported (vide supra Scheme 75).
The methylation of 194 was used to facilitate the 1,3,5-triazine ring closure providing 195 (Scheme 88).69
The synthesis of 2,2-bis(trifluoromethyl)-4-(4-chlorophenyl)-6,7-dihydrothiazolo[3,2-a][1,3,5]triazines (119c) was performed in the stepwise manner (cf. Scheme 49).63 Adduct 196, obtained with the 94% yield from the exothermic reaction between 2-aminothiazoline (18) and 4-chlorobenzoylimine of hexafluoroacetone (118c) in benzene, underwent further cyclization to give 119c (Scheme 89).
The thermal cyclocondensation of 197, prepared with the 91% yield by treatment of 2-aminobenzothiazole (36) with hexafluoroacetone ethoxycarbonylimine (112) in diethyl ether, afforded 116e (Scheme 90).102 The structure of the product (116e) was confirmed by X-ray crystallography. Sokolov and Aksinenko62 used these data to support the regiochemistry of the direct synthesis of 116 from 36 and 112 without isolation of intermediates (vide supra Scheme 48).
The pyrimidine and 1,3,5-triazine ring closure with formation of cyclazine 199 occurred when 198 was heated in diphenyl ether (Scheme 91).103
2.2. Synthesis of thiazolo[3,2-a][1,3,5]triazines by annelation of the thiazole ring onto a 1,3,5-triazine scaffold.
Even though the annelation of the thiazole ring onto a 1,3,5-triazine scaffold was the first method for the synthesis of thiazolo[3,2-a][1,3,5]triazines (vide supra Scheme 1),2,3 this approach has not been thoroughly explored. The main contributions to this field were done by Dovlatyan et al.,104-108 who studied the reactions of 1,3,5-triazines with 1,2-dichloroethane and cyclizations of the 2-chloroethyl substituted 1,3,5-triazines. The reaction of triazine 200 with 1,2-dichloroethane in the presence of alkali was reported to result in the formation of salt 201, which was converted to the corresponding free base (88% yield) by treatment with aqueous sodium hydroxide (Scheme 92).104
The similar reaction of the methylated analogue of 200 provided triazine 202, which underwent further cyclization to 203 upon heating in xylene (Scheme 93).104
The cyclocondensation of 2-(2-chloroethylthio)-4-dialkylamino-6-methoxy-1,3,5-triazines (204) with elimination of methyl chloride was found to afford 205 (Scheme 94).105,106 The detail mass-spectrometry investigation of the products 205 was also reported.107
Compounds 205 were also prepared from triazines 206 via the translocative rearrangement to 207 and subsequent intramolecular cyclization with the methyl chloride elimination (Scheme 95).108 The conversion of 206 into 2-dialkylamino-6,7-dihydrothiazolo[3,2-a][1,3,5]triazin-4-ones (205) was also performed by heating at 180-190 °C without isolation of 207.
The similar synthesis of 2-dialkylamino-6,7-dihydrothiazolo[3,2-a][1,3,5]triazin-4-thiones (210) from corresponding 208 was proposed105 to involve transfer of the chloroethyl group to the endocyclic nitrogen (Scheme 96). This pathway was suggested by intermediates 209, which were isolated when the reaction was stopped after 15 min of heating 208 in toluene.
The treatment of triazines 211 with bromoacetophenones (212, R1 = H) in acetone resulted in the formation of salts 213 (Scheme 97), which were claimed109 as potential antiulcer agents. The similar reaction of 211 with 3-aroyl-3-bromopropionic acids (212, R1 = CH2CO2H) in methanol at ambient temperature afforded corresponding adducts 213, which were able to increase survival time of the mice with P338 leukemia.110 The dehydration products 214 were isolated when the reaction was carried out on heating.
The cyclocondensation of 215 in polyphosphoric acid was reported111 to produce 216 (Scheme 98). 2,4,6-Triphenyl-3,4-dihydro-2H-thiazolo[3,2-a][1,3,5]triazine (216a, R1 = H, R2 = R3 = Ph) was explored as a stabilizer for the single base propellant.112
Acids 217 were found to undergo intramolecular cyclization affording 218 (Scheme 99).113,114 2,4-Diphenyl-2,3,4,7-tetrahydrothiazolo[3,2-a][1,3,5]triazin-6-one (218a, R1 = H, R2 = Ph) was explored as a stabilizer for the double base propellant.115
2.3. Synthesis of thiazolo[3,2-a][1,3,5]triazines via formation of the thiazole and 1,3,5-triazine ring.
2-Amino-4-imino-1,3,5-triazino[2,1-b]benzothiazole (221) was prepared from o-aminothiophenol (219) and substituted cyanoguanidine 220 via consecutive formation of the thiazole and triazine rings (Scheme 100).69
3. HETEROCYCLIC SYSTEMS COMPRISING THIAZOLO[3,4-a][1,3,5]TRIAZINE CORE
The 1,3,5-triazine ring fused with 3,4-side of thiazole has been reported only as a part of more complex heterocyclic systems. For example, tetracyclic compound 223 was formed by the condensation of 222 with benzaldelyde (Scheme 101).116
The cyclazines comprising the thiazolo[3,4-a][1,3,5]triazine and pyrimidine rings were synthesized by Ceder and Beijer.103,117 The reaction of 2,4-diamino-5-methylthiazole (224) with ethyl 2-cyano-3-ethoxyacrylate followed by heating with acetic-formic anhydride resulted in the formation of 8-ethoxycarbonyl-4-methyl-5-thia-1,3,6-triazacycl[3.2.3]azine (225) with low yield (Scheme 102).117
For the preparation of analogous phenyl substituted cyclazine 227, intermediate 226 was isolated and converted to 227 by the treatment with acetic-formic anhydride at ambient temperature (Scheme 103).103
The thermal cyclocondensation of 228, a product of the acetylation of amine 226, allowed the synthesis of 229 (Scheme 104).103
4. CONCLUSION
A variety of effective methods for the preparation of thiazolo[3,2-a][1,3,5]triazines has been developed, mainly by the annelation of the 1,3,5-triazine ring onto a 1,2,4-triazole scaffold. The spectrum of known biological activities of compounds bearing thiazolo[3,2-a][1,3,5]triazine skeleton includes antitumor, antiviral, antibacterial, antifungal, anthelmintic, and anticonvulsant activities. The examples of the phospholipase C-γ inhibitors, 5-HT2-receptor antagonists, and selective DNA binding agents have been reported. However, these data are sporadic and more systematic medicinal chemistry investigations of thiazolo[3,2-a][1,3,5]triazines are required. The present review aims to serve as a background for the research in this area.
The chemistry of thiazolo[3,4-a][1,3,5]triazines remains in the initial development stages and its potential is to be discovered in future.
References
1. Part 3 in the series of reviews “Fused 1,3,5-triazines.” For Parts 1 and 2 see: A. V. Dolzhenko, A. V. Dolzhenko, and W. K. Chui, Heterocycles, 2006, 68, 1723; CrossRef A. V. Dolzhenko, A. V. Dolzhenko, and W. K. Chui, Heterocycles, 2008, 75, 1575. CrossRef
2. B. Rathke, Ber., 1887, 20, 1059. CrossRef
3. B. Rathke, Ber., 1888, 21, 874. CrossRef
4. S. Y. Solov'eva-Yavits, S. M. Ramsh, and A. I. Ginak, Khim. Geterotsikl. Soedin., 1981, 477 (Chem. Heterocycl. Compd., 1981, 17, 340).
5. S. M. Ramsh, S. Y. Solov'eva-Yavits, A. I. Ginak, and E. G. Sochilin, Pat. SU 802283, 1981, (Chem. Abstr., 95, 25151).
6. S. Y. Solov'eva, S. M. Ramsh, and A. I. Ginak, Khim. Geterotsikl. Soedin., 1983, 1204 (Chem. Heterocycl. Compd., 1983, 19, 961).
7. S. M. Ramsh, A. G. Ivanenko, V. A. Shpilevyi, N. L. Medvedskiy, and P. M. Kushakova, Khim. Geterotsikl. Soedin., 2005, 1089 (Chem. Heterocycl. Compd., 2005, 41, 921). CrossRef
8. S. M. Ramsh, A. G. Ivanenko, N. L. Medvedskiy, D. G. Lagerev, D. B. Lazarev, and L. N. B. Costa, Khim. Geterotsikl. Soedin., 2006, 1252 (Chem. Heterocycl. Compd., 2006, 42, 1086). CrossRef
9. E. Gallmeier, T. Hucl, J. R. Brody, D. A. Dezentje, K. Tahir, J. Kasparkova, V. Brabec, K. E. Bachman, and S. E. Kern, Cancer Res., 2007, 67, 2169. CrossRef
10. S. M. Ramsh, N. L. Medvedskiy, and S. O. Uryupov, Khim. Geterotsikl. Soedin., 2006, 1095 (Chem. Heterocycl. Compd., 2006, 42, 948). CrossRef
11. J. Reynisson, W. Court, C. O'Neill, J. Day, L. Patterson, E. McDonald, P. Workman, M. Katan, and S. A. Eccles, Bioorg. Med. Chem., 2009, 17, 3169. CrossRef
12. H. P. Penner and A. R. Conklin, Jr., J. Heterocycl. Chem., 1967, 4, 93. CrossRef
13. D. C. H. Bigg and S. R. Purvis, J. Heterocycl. Chem., 1976, 13, 977. CrossRef
14. D. L. Klayman and G. W. Milne, Tetrahedron, 1969, 25, 191. CrossRef
15. J. Karle, J. L. Flippen, and I. L. Karle, Z. Kristallogr., 1967, 125, 201. CrossRef
16. R. Richter and H. Ulrich, Chem. Ber., 1970, 103, 3525. CrossRef
17. M. A. Abdel-Rahman, Bull. Chem. Soc. Jpn., 1993, 66, 510. CrossRef
18. U. von Gizycki and G. Oertel, Angew. Chem., Int. Ed. Engl., 1968, 7, 380. CrossRef
19. B. G. Khadse, M. H. Shah, C. V. Deliwala, M. B. Bhide, S. S. Mahajani, and V. M. Bhat, Bull. Haffkine Inst., 1977, 5, 9.
20. A. S. Bobade, C. N. Desai, and B. G. Khadse, Indian J. Pharm. Sci., 1985, 47, 132.
21. J. Bödeker, K. Courault, A. Köckritz, and P. Köckritz, J. Prakt. Chem., 1983, 325, 463. CrossRef
22. J. Bödeker, P. Köckritz, and K. Courault, Z. Chem. 1979, 19, 59.
23. U. von Gizycki and G. Oertel, Angew. Chem., Int. Ed. Engl., 1968, 7, 381. CrossRef
24. V. A. Dorokhov, A. V. Asilyev, S. V. Baranin, and V. S. Bogdanov, Russ. Chem. Bull., 1998, 47, 1626. CrossRef
25. M. J. Haddadin, M. J. Kurth, and M. M. Olmstead, Tetrahedron Lett., 2000, 41, 5613. CrossRef
26. K. S. Huang, M. J. Haddadin, M. M. Olmstead, and M. J. Kurth, J. Org. Chem., 2001, 66, 1310. CrossRef
27. O. P. Cetinkol, A. E. Engelhart, R. K. Nanjunda, W. D. Wilson, and N. V. Hud, ChemBioChem, 2008, 9, 1889.
28. O. P. Cetinkol and N. V. Hud, Nucleic Acids Res., 2009, 37, 611. CrossRef
29. K. Wermann, M. Walther, W. Guenther, H. Goerls, and E. Anders, J. Org. Chem., 2001, 66, 720. CrossRef
30. L. D. S. Yadav, V. K. Rai, and S. Yadav, Lett. Org. Chem., 2007, 4, 47. CrossRef
31. L. D. S. Yadav, S. Yadav, and V. K. Rai, Green Chem., 2006, 8, 455. CrossRef
32. L. D. S. Yadav and R. Kapoor, Tetrahedron Lett., 2003, 44, 8951. CrossRef
33. Y. G. Bal'on and V. A. Smirnov, Zh. Org. Khim., 1980, 16, 738.
34. Y. G. Bal'on and V. A. Smirnov, Zh. Org. Khim., 1981, 17, 391.
35. M. V. Vovk, Y. G. Bal'on, I. G. Krainikova, and L. I. Samarai, Ukr. Khim. Zh., 1995, 61, 63.
36. F. W. Hoover, H. B. Stevenson, and H. S. Rothrock, J. Org. Chem., 1963, 28, 1825. CrossRef
37. H. Zinner, U. Rosenthal, H. P. Kruse, S. Rosenthal, and M. Schnell, J. Prakt. Chem., 1978, 320, 625. CrossRef
38. J. C. Kauer and A. K. Schneider, J. Am. Chem. Soc., 1960, 82, 852. CrossRef
39. Y. Watanabe, H. Usui, S. Kobayashi, H. Yoshiwara, T. Shibano, T. Tanaka, Y. Morishima, M. Yasuoka, and M. Kanao, J. Med. Chem., 1992, 35, 189. CrossRef
40. Y. Watanabe and H. Usui, Pat. JP 04054184, 1992 (Chem. Abstr., 117, 26593).
41. Y. Watanabe, H. Usui, T. Shibano, T. Tanaka, Y. Morishima, and M. Yasuoka, Pat. EP 401707, 1990 (Chem. Abstr., 114, 185570).
42. R. A. Coburn and B. Bhooshan, J. Org. Chem., 1973, 38, 3868. CrossRef
43. H. Gotthardt and J. Blum, Chem. Ber., 1988, 121, 1579. CrossRef
44. D. L. Klayman and T. S. Woods, J. Org. Chem., 1975, 40, 2000. CrossRef
45. B. Radha Rani, M. F. Rahman, and U. T. Bhalerao, Synth. Commun., 1991, 21, 319. CrossRef
46. G. Barnikow and J. Bödeker, J. Prakt. Chem., 1971, 313, 1148. CrossRef
47. G. M. Kaplan, A. N. Frolov, and A. V. El'tsov, Zh. Org. Khim., 1991, 27, 201.
48. U. Yunus, M. K. Tahir, M. H. Bhatti, S. Ali, and M. Helliwell, Acta Crystallogr., 2007, E63, o3690.
49. U. Yunus, M. K. Tahir, M. H. Bhatti, and W. Y. Wong, Acta Crystallogr., 2008, E64, o722.
50. S. Saeed, N. Rashid, P. G. Jones, and U. Yunas, J. Heterocycl. Chem., 2010, 47, 908. CrossRef
51. G. Barnikow and H. Ebeling, Z. Chem., 1973, 13, 468.
52. G. Barnikow and H. Ebeling, Z. Chem., 1974, 14, 356.
53. L. Capuano and H. J. Schrepfer, Chem. Ber., 1971, 104, 3039. CrossRef
54. D. L. Klayman and T. S. Woods, J. Org. Chem., 1974, 39, 1819. CrossRef
55. J. L. Flippen, Acta Crystallogr., 1974, B30, 1123.
56. M. Nagano, T. Matsui, J. Tobitsuka, and K. Oyamada, Chem. Pharm. Bull., 1972, 20, 2626.
57. M. Nagano, J. Tobitsuka, T. Matsui, and K. Oyamada, Chem. Pharm. Bull., 1972, 20, 2618.
58. K. Oyamada, M. Nagano, T. Matsui, and J. Tobitsuka, Pat. JP 47012350, 1972 (Chem. Abstr., 77, 34595).
59. W. Abraham and G. Barnikow, Tetrahedron, 1973, 29, 699. CrossRef
60. I. A. Poplavskaya, G. B. Aubakirova, and R. G. Kurmangalieva, Zh. Obshch. Khim., 1995, 65, 480.
61. M. V. Vovk and V. I. Dorokhov, Russ. J. Org. Chem., 1997, 33, 96.
62. V. B. Sokolov and A. Y. Aksinenko, Russ. Chem. Bull., 2003, 52, 2167. CrossRef
63. V. B. Sokolov, A. Y. Aksinenko, T. A. Epishina, T. V. Goreva, A. N. Pushin, and I. V. Martynov, Russ. Chem. Bull., 2005, 54, 1667. CrossRef
64. H. Usui, Y. Watanabe, and M. Kanao, J. Heterocycl. Chem., 1993, 30, 551. CrossRef
65. W. D. Dean and E. P. Papadopoulos, J. Heterocycl. Chem., 1982, 19, 171. CrossRef
66. E. Degener, H. Holtschmidt, and K. Swincicki, Pat. BE 633232, 1963 (Chem. Abstr., 60, 75426).
67. G. B. Okide, J. Heterocycl. Chem., 1994, 31, 535. CrossRef
68. M. Karanik, M. Paetzel, and J. Liebscher, Synthesis, 2003, 1201. CrossRef
69. C. Bennion and D. Robinson, Pat. EP 93515, 1983 (Chem. Abstr., 100, 85727).
70. H. Böhme and H. J. Drechsler, Arch. Pharm. (Weinheim), 1979, 312, 1011. CrossRef
71. H. Böhme and J. P. Denis, Arch. Pharm. (Weinheim), 1982, 315, 227. CrossRef
72. S. H. Abdel-Hafez, Phosphorus, Sulfur Silicon Relat. Elem., 2003, 178, 2563. CrossRef
73. S. A. Abdel-Mohsen, J. Chin. Chem. Soc. (Taipei), 2003, 50, 1085.
74. M. A. Abdel-Rahman, Synth. Commun., 1993, 23, 1427. CrossRef
75. R. M. Mishra, D. V. Singh, and A. R. Mishra, Indian J. Heterocycl. Chem., 2004, 14, 85.
76. P. Kriplani, P. Swarnkar, and K. G. Ojha, Heterocycl. Commun., 2005, 11, 527.
77. P. Kriplani, P. Swarnkar, R. Maheshwari, and K. G. Ojha, E-J. Chem., 2006, 3, 307.
78. M. Marchalin, J. Lesko, and A. Martvon, Collect. Czech. Chem. Commun., 1982, 47, 1229.
79. C. Tanaka, K. Nasu, N. Yamamoto, and M. Shibata, Chem. Pharm. Bull., 1982, 30, 4195.
80. T. George and R. Tahilramani, Synthesis, 1974, 346. CrossRef
81. K. Ura, I. Sakata, K. Makino, Y. Kawamura, Y. Kawamura, T. Igai, and T. Oguchi, Pat. JP 56051489, 1981 (Chem. Abstr., 95, 115617).
82. K. Maeda, M. Kaeriyama, N. Matsui, A. Mizuno, Y. Yasuda, and A. Nakada, Pat. JP 56079694, 1981 (Chem. Abstr., 95, 204005).
83. M. Kaeriyama, S. Suga, A. Hashimoto, and S. Sakai, Pat. JP 58157794, 1983 (Chem. Abstr., 100, 103397).
84. R. Bossio, S. Marcaccini, V. Parrini, and R. Pepino, J. Heterocycl. Chem., 1985, 22, 1147. CrossRef
85. S. M. Bayomi, A. Tantawy, and M. M. El-Kerdawy, J. Chem. Soc. Pak., 1987, 9, 487.
86. A. Kreutzberger, Arch. Pharm. (Weinheim), 1976, 309, 794. CrossRef
87. Y. Kihara, S. Kabashima, T. Yamasaki, T. Ohkawara, and M. Furukawa, J. Heterocycl. Chem., 1990, 27, 1213. CrossRef
88. D. V. Krylsky, K. S. Shikhaliev, and A. S. Solovyev, Khim. Geterotsikl. Soedin., 2001, 567 (Chem. Heterocycl. Compd., 2001, 37, 524). CrossRef
89. A. V. Dolzhenko, W. K. Chui, and A. V. Dolzhenko, Synthesis, 2006, 597. CrossRef
90. A. Etienne and B. Bonte, Bull. Soc. Chim. Fr., 1975, 1419.
91. E. A. A. Hafez, M. R. H. Elmoghayar, and M. M. M. Ramiz, Liebigs Ann. Chem., 1987, 65. CrossRef
92. R. M. Fikry, N. A. Ismael, A. A. El-Bahnasawy, and A. A. S. El-Ahl, Phosphorus, Sulfur Silicon Relat. Elem., 2004, 179, 1227. CrossRef
93. M. R. H. Elmoghayar, M. K. A. Ibrahim, and F. M. Darwish, Org. Prep. Proced. Int., 1984, 16, 1. CrossRef
94. K. P. Channabasavaraj, M. Ahmed, A. C. Bajji, Y. N. Manohara, and I. J. Kuppast, Indian J. Heterocycl. Chem., 2005, 14, 351.
95. A. Pande and V. K. Saxena, Indian J. Pharm. Sci., 1985, 47, 227.
96. S. Giri, A. Singh, and J. P. N. Giri, Bokin Bobai, 1983, 11, 575.
97. N. Siddiqui, A. Rana, S. A. Khan, S. E. Haque, M. S. Alam, W. Ahsan, and S. Ahmed, J. Enzyme Inhib. Med. Chem., 2009, 24, 1344. CrossRef
98. M. H. Elnagdi, S. M. Fahmy, M. R. H. Elmoghayar, and E. M. Kandeel, J. Heterocycl. Chem., 1979, 16, 61. CrossRef
99. S. M. Fahmy, M. K. A. Ibraheim, K. Abouhadid, M. H. Elnagdi, and H. H. S. Alnima, Arch. Pharm. (Weinheim), 1982, 315, 791. CrossRef
100. S. M. Fahmy, M. K. A. Ibrahim, K. Abouhadid, M. H. Elnagdi, and H. H. S. Alnima, Iraqi J. Sci., 1982, 23, 28.
101. M. Nagano, T. Matsui, J. Tobitsuka, and K. Oyamada, Chem. Pharm. Bull., 1973, 21, 74.
102. A. N. Chekhlov, O. V. Korenchenko, A. Y. Aksinenko, V. B. Sokolov, and I. V. Martynov, Dokl. Akad. Nauk, 1994, 339, 503.
103. O. Ceder and B. Beijer, Tetrahedron, 1974, 30, 3657. CrossRef
104. V. V. Dovlatyan, N. K. Khachatryan, and T. A. Gomktsyan, Arm. Khim. Zh., 1982, 35, 534.
105. V. V. Dovlatyan, K. A. Eliazyan, S. M. Saakyan, and R. G. Mirzoyan, Khim. Geterotsikl. Soedin., 1981, 1275 (Chem. Heterocycl. Compd., 1981, 17, 957). CrossRef
106. V. V. Dovlatyan, K. A. Eliazyan, and A. V. Azatyan, Khim. Geterotsikl. Soedin., 1987, 978 (Chem. Heterocycl. Compd., 1982, 23, 803). CrossRef
107. R. G. Mirzoyan, S. M. Saakyan, M. P. Demirchyan, P. B. Terent'ev, V. V. Dovlatyan, and A. V. Dovlatyan, Khim. Geterotsikl. Soedin., 1982, 124 (Chem. Heterocycl. Compd., 1982, 18, 106). CrossRef
108. V. V. Dovlatyan, A. V. Dovlatyan, K. A. Eliazyan, and R. G. Mirzoyan, Khim. Geterotsikl. Soedin., 1977, 1420 (Chem. Heterocycl. Compd., 1977, 13, 1140). CrossRef
109. J. T. A. Boyle, Pat. US 4127660, 1978 (Chem. Abstr., 90, 104023).
110. J. L. Archibald and J. T. A. Boyle, Pat. GB 2096609, 1982 (Chem. Abstr., 98, 179424).
111. A. A. W. Soliman, J. Chem. Eng. Data, 1984, 29, 99. CrossRef
112. A. A. W. Soliman, Propellants, Explos., Pyrotech., 1985, 10, 82. CrossRef
113. A. A. W. Soliman, Z. Naturforsch., 1976, 31B, 1397.
114. A. A. W. Soliman, Egypt. J. Chem., 1985, 27, 471.
115. A. A. W. Soliman, Propellants Explos., 1977, 2, 100. CrossRef
116. R. M. Mohareb, H. F. Zohdi, and W. W. Wardakhan, Monatsh. Chem., 1995, 126, 1391. CrossRef
117. O. Ceder and B. Beijer, Acta Chem. Scand., 1976, B30, 468. CrossRef