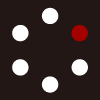
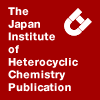
HETEROCYCLES
An International Journal for Reviews and Communications in Heterocyclic ChemistryWeb Edition ISSN: 1881-0942
Published online by The Japan Institute of Heterocyclic Chemistry
e-Journal
Full Text HTML
Received, 18th November, 2010, Accepted, 6th January, 2011, Published online, 14th January, 2011.
DOI: 10.3987/COM-10-12104
■ Synthesis and Antimicrobial Evaluation of Some New Tetrahydropyrimidine Derivatives
Yahia N. Mabkhot, Nabila A. Kheder,* and Ahmad M. Farag
Department of Chemistry, Faculty of Science, University of Cairo, Giza 12613, Egypt
Abstract
The utility of 1,2,3,4-tetrahydropyrimidine-5-carboxylate (1a,b), and 1,2-dihydropyrimidine-5-carboxylate (2) in the synthesis of some new functionalized pyrimidine derivatives such as 6-(2-(dimethylamino)vinyl)-1,2,3,4-tetrahydropyrimidine, 6-(1-cyano-2-(phenylamino)-2-thioxoethyl)-1,2,3,4-tetra-hydropyrimidine and 4,6-distyryl-1,2-dihydropyrimidine derivatives is reported. Antimicrobial evaluation of some selected examples from the synthesized products was carried out and showed promising results.Pyrimidine derivatives are reported to possess antibacterial,1-3 antifungal4,5 anticancer,6,7 anti- inflammatory,8,9 and cardioprotective effects.10 In addition, certain pyrimidine derivatives are known to display antimalarial, antifilarial and antileshmainal activities.11 Also, pyrimidine nucleus is considered as one of the most important classes of chemotherapeutic drugs. Many pyrimidine derivatives are used for thyroid drugs and leukemia.12 In view of the these results and as an extension of our recent work concerned with the synthesis of heterocycles of interested biological activity13-20 we decided to synthesize some new pyrimidine derivatives starting from the readily obtainable 1,2,3,4-tetrahydropyrimidine-5-carboxylate derivatives 1a,21 1b,22 and dihydropyrimidine 223 as highly versatile and useful building blocks for the synthesis of a wide variety of pyrimidine derivatives and to test their antimicrobial activity.
1,2,3,4-Tetrahydropyrimidine 1a was found to react readily with dimethylformamide dimethyl acetal (DMF-DMA) to produce ethyl 6-(2-(dimethylamino)vinyl)-2-oxo-4-phenyl-1,2,3,4-tetrahydropyrimidine-5-carboxylate (3) (Scheme 1). The IR spectrum of the product 3 showed absorption bands at, 1624, 1701, 3117, 3244 cm-1 due to two carbonyl groups, and two imino functions, respectively. Its 1H NMR spectrum showed a triplet signal at δ 1.11 (J = 6.9 Hz) due to CH3 protons, singlet signal at δ 2.85, characteristics for two methyl protons, a quartet signal at δ 3.96 (J = 6.9 Hz) due to CH2 protons, a doublet signal at δ 5.17 (J = 3.6 Hz) due to CH proton, two doublets at δ 6.18 and δ 7.48 (J = 14.1 Hz) due to two olefinic protons, and two D2O-exchangeable signals at δ 7.52 and 8.61 corresponding to two NH protons, in addition to an aromatic multiplet in the region δ 7.22-7.29. The value of the coupling constant for the ethylenic protons indicates that the pyrimidine 3 exists exclusively in the E-configuration.
The versatile synthons; ethyl 6-(bromomethyl)-2-oxo-4-phenyl-1,2,3,4-tetrahydropyrimidine-5-carboxylate (4a)24 and ethyl 6-(bromomethyl)-2-oxo-4-methyl-1,2,3,4-tetrahydropyrimidine-5-carboxylate (4b),24 were obtained from the bromination of ethyl 6-methyl-2-oxo-4-phenyl-1,2,3,4-tetrahydropyrimidine-5-carboxylate (1a) and ethyl 4,6-dimethyl-2-oxo-1,2,3,4-tetrahydropyrimidine-5-carboxylate (1b), in acetic acid. Attempted reaction of compound 4b with ethyl 3-oxo-3-phenylpropanoate (5), in order to obtain the expected structure 7 or 8, was unsuccessful. Instead, compound 4b underwent a thermal intramolecular cyclization reaction via elimination of bromoethane and afforded the corresponding 4-methyl-3,4-dihydrofuro[3,4-d]pyrimidine-2,5(1H,7H)-dione (6) (Scheme 2). Although ethyl 3-oxo-3-phenylpropanoate (5) is typically considered as a reagent, however, it behaves as an adequate high boiling solvent in this thermal cyclization reaction.
The first step of this reaction is connected with a nucleophilic attack of the lone pair of electrons of carbonyl oxygen in the ester group on the 6-bromomethyl group in 4b followed by thermal elimination of bromoethane molecule under the reaction conditions to afford the final product 6. The latter product was found to be identical in all respects [mp, mixed mp and IR spectrum] with that obtained from literature.24
Pyridinium N-ylides are highly interesting compounds due to their reactivity as organic dipoles as well as their biological properties and applications. Usually, simple electron attracting groups, such as carbonyl, cyano etc., have been used to delocalize the negative charge. Less attention has been paid to the possibility of using heteroaryl groups as stabilizers except in the case of some tetrazole25 and triazole stabilized ylides26 which already described. Our work in this field has been concerned with the synthesis of pyrimid-6-yl stabilized pyridine ylide27 as a convenient root for producing highly stable dipole. Thus, pyridinium bromides 9a and 9b were prepared from 4a or 4b with an equivalent amount of pyridine in dry THF (Scheme 3).
Reaction of the ethyl 6-(bromomethyl)-2-oxo-4-phenyl-1,2,3,4-tetrahydropyrimidine-5-carboxylate (4a) with potassium cyanide afforded the corresponding pyrimidine derivative 10.28
Treatment of the latter compound with phenyl isothiocyanate, in N,N-dimethylformamide, and in the presence of potassium hydroxide, at room temperature, followed by treatment with dilute hydrochloric acid, afforded the corresponding ethyl 6-(1-cyano-2-(phenylamino)-2-thioxoethyl)-2-oxo-4-phenyl-1,2,3,4-tetrahydropyrimidine-5-carboxylate (12) (Scheme 4).
Treatment of ethyl 4,6-dimethyl-2-oxo-1,2-dihydropyrimidine-5-carboxylate (2) with the appropriate aromatic aldehydes in the presence of a catalytic amount of ZnCl2, afforded the corresponding bis-arylidene derivatives 13a-c (Scheme 5). The above reaction is assumed to proceed via loss of two water molecules.
The Structure of the isolated products was assigned as ethyl 4,6-bis(substituted)-2-oxo-1,2-dihydropyrimidine-5-carboxylate 13a-c based on their elemental analyses and their spectroscopic data. The 1H NMR spectrum spectra of the isolated products showed in each case the absence of methyl signal and the value of the coupling constant for the ethylenic protons indicates that the pyrimidine 13 exists exclusively in the E-configuration.
In conclusion, the utility 1,2,3,4-tetrahydropyrimidine-5-carboxylate (1a,b), and 1,2-dihydropyrimidine-5-carboxylate (2) in the synthesis of some new functionalized pyrimidine derivatives of biological and pharmaceutical importance is reported.
EXPERIMENTAL
All melting points were measured on a Gallenkamp melting point apparatus. The infrared spectra were recorded in potassium bromide disks on a pye Unicam SP 3300 and Shimadzu FT IR 8101 PC infrared spectrophotometers. The NMR spectra were recorded on a Varian Mercury VX-300 NMR spectrometer. 1H spectra were run at 300 MHz and 13C spectra were run at 75.46 MHz in dimethyl sulphoxide (DMSO-d6). Chemical shifts were related to that of the solvent. Mass spectra were recorded on a Shimadzu GCMS-QP 1000 EX mass spectrometer at 70 e.V. Elemental analyses (C, H, N, S) were carried out at the Microanalytical Center of Cairo University, Giza, Egypt. The biological evaluation of the products 9b, 12, 13a and 13c were carried out in the Medical Mycology Laboratory of the Regional Center for Mycology and Biotechnology of Al-Azhar University, Cairo, Egypt. Ethyl 6-methyl-2-oxo-4-phenyl-1,2,3,4-tetrahydropyrimidine-5-carboxylate (1a),21 ethyl 4,6-dimethyl-2-oxo- 1,2,3,4-tetrahydropyrimidine-5-carboxylate (1b),22 ethyl 4,6-dimethyl-2-oxo-1,2-dihydropyrimidine-5-carboxylate (2),23 ethyl 6-(bromomethyl)-2-oxo-4-phenyl-1,2,3,4-tetrahydropyrimidine-5-carboxylate (4a),24 ethyl 6-(bromometh-yl)-2-oxo-4-methyl-1,2,3,4-tetrahydropyrimidine-5-carboxylate (4b)24 and ethyl 6-(cyanomethyl)-2-oxo-4-phenyl-1,2,3,4-tetrahydropyrimidine-5-carboxylate (10)28 were prepared following the literature procedure.
Ethyl 6-(2-(dimethylamino)vinyl)-2-oxo-4-phenyl-1,2,3,4-tetrahydropyrimidine-5-carboxylate (3).
A mixture of compound 1 (2.60 g, 10 mmol) and dimethylformamide dimethyl acetal (DMF-DMA) (1.33 mL, 10 mmol) in dry xylene (20 mL) was refluxed for 3 h, then left to cool. The precipitated product was filtered off, washed with EtOH and dried. Recrystallization from dioxane/ EtOH gave yellow crystals of compound 3 in 85% yield, mp 244-245 oC; IR (KBr) v 3244 (NH), 3117 (NH), 1701 (C=O), 1624 (C=O) cm-1; 1H NMR (DMSO-d6) δ 1.11 (t, 3H, CH3, J = 6.9 Hz), 2.85 (s, 6H, 2NCH3), 3.96 (q, 2H, CH2, J = 6.9 Hz), 5.17 (d, 1H, J = 3.6 Hz), 6.18 (d, 1H, CH, J= 14.1 Hz), 7.22-7.29 (m, 5H), 7.48 (d,1H, CH, J= 14.1 Hz), 7.52 (s, 1H, D2O-exchangeable NH), 8.61 (s, 1H, D2O-exchangeable NH); MS m/z (%) 315 (M+, 20.0), 238 (100), 77 (36.0). Anal. Calcd for C17H21N3O3: C, 64.74; H, 6.71; N, 13.32. Found: C, 64.80; H, 6.67; N, 13.26%.
Attempted reaction of 6-bromomethylpyrimidine 4b with ethyl 3-oxo-3-phenylpropanoate (5).
To an ethanolic solution of 6-bromomethylpyrimidine 4b (0.28 g, 1 mmol) and ethyl 3-oxo-3-phenylpropanoate (5) (0.19 mL, 1 mmol) was added few drops of piperidine and the reaction mixture was refluxed for 4 h. The formed solid product was collected by filtration, washed with EtOH and purified by crystallisation from the DMF to afford a product identified as 4-methyl-3,4-dihydrofuro[3,4-d]pyrimidine- 2,5(1H,7H)-dione (6): Yield (70%), mp 268 °C. The latter product is assumed to be formed through an intramolecular cyclization of compound 4b via thermal elimination of bromoethane and it was found to be identical in all respects (mp, mixed mp and IR spectrum) with an authentic sample prepared according to a literature procedure.24 It is noteworthy that ethyl 3-oxo-3-phenylpropanoate (5) was not involved in above reaction but it acted as a suitable high boiling solvent for the above reaction. IR (KBr) v 3310 (NH), 3228 (NH), 1726 (C=O), 1662 (C=O) cm-1; 1H NMR (DMSO-d6) δ 1.24 (s, 3H, CH3), 4.27 (d, 1H, CH, J = 5.1 Hz), 4.74 (s, 2H, CH2), 7.3 (s, 1H, D2O-exchangeable NH), 9.68 (s, 1H, D2O-exchangeable NH). Anal. Calcd for C7H8N2O3: C, 50.00; H, 4.80; N, 16.66. Found: C, 50.12; H, 4.88; N, 16.64%.
Reaction of 6-bromomethylpyrimidine 4a or 4b with pyridine.
To a solution of 6-bromomethylpyrimidine 4a or 4b (10 mmol) in dry THF (20 mL), pyridine (0.8 mL, 10 mmol) was added. The reaction mixture was refluxed for 20 min, then left to cool to rt. The solid product was filtered off, washed with Et2O and dried to afford the corresponding pyridinium salts 9a and 9b, respectively.
1-[(5-(Ethoxycarbonyl)-2-oxo-6-phenyl-1,2,3,6-tetrahydropyrimidin-4-yl)methyl]pyridiniumbromide (9a): Yield (88%), mp 197 ºC; IR (KBr) v 3230 (NH), 3093 (NH), 1700 (C=O), 1647 (C=O) cm-1; 1H NMR (DMSO-d6) δ 1.09 (t, 3H, CH3, J = 6.9 Hz), 3.97 (q, 2H, CH2, J = 6.9 Hz), 4.82 (s, 2H, CH2), 5.14 (s, 1H), 7.22-7.35 (m, 10H, Ar-H), 7.70 (s, br., 1H, D2O-exchangeable NH), 9.15 (s, br., 1H, D2O-exchangeable NH). Anal. Calcd for C19H20BrO3N3: C, 54.56; H, 4.82; N, 10.05. Found: C, 54.62; H, 4.89; N, 10.12%.
1-[(5-(Ethoxycarbonyl)-6-methyl-2-oxo-1,2,3,6-tetrahydropyrimidin-4-yl)methyl]pyridiniumbromide (9b): Yield (83%), mp 220 ºC; IR (KBr) v 3283 (NH), 3202 (NH), 1701 (C=O), 1659 (C=O) cm-1; 1H NMR (DMSO-d6) δ 1.18 (t, 3H, CH3, J = 6.9 Hz), 1.22 (s, 3H, CH3), 4.12 (q, 2H, CH2, J = 6.9 Hz), 4.24 (s, 2H, CH2), 5.58 (s, 1H) , 7.48 (s, br., 1H, D2O-exchangeable NH), 8.17 (m, 2H), 8.64 (m, 1 H), 9.0 (d, 2H), 9.52 (s, br., 1H, D2O-exchangeable NH). Anal. Calcd for C14H18BrO3N3: C, 47.20; H, 5.09; N, 11.80. Found: C, 47.29; H, 5.02; N, 11.88%.
Ethyl 6-(1-cyano-2-(phenylamino)-2-thioxoethyl)-2-oxo-4-phenyl-1,2,3,4-tetrahydropyrimidine-5-carboxylate (12).
To a stirred solution of potassium hydroxide (0.11 g, 2 mmol) in DMF (20 mL) was added the pyrimidine derivative 1028 (0. 57 g, 2 mmol). After stirring for 30 min, phenyl isothiocyanate (0.27 g, 2 mmol) was added to the resulting mixture. Stirring was continued for 6 h, and then the reaction mixture was poured over a cold solution of 0.5 N hydrochloric acid. The formed solid product was filtered off, washed with water, dried, and finally recrystallized from the EtOH to afford compound 12 in 62% yield, mp 175 ºC; IR (KBr) v 3209 (NH), 3101 (NH), 3051 (NH), 1697 (C=O), 1632 (C=O) cm-1; 1H NMR (DMSO-d6) δ 1.1 (t, 3H, CH3, J = 6.9 Hz), 4.06 (q, 2H, CH2, J = 6.9 Hz), 4.13 (s, 1H), 5.18 (d, 1H, CH, J = 2.4 Hz), 7.22-7.37 (m, 10H, Ar-H), 7.91 (s, br., 1H, D2O-exchangeable NH), 8.0 (s, br., 1H, D2O-exchangeable NH), 9.71 (s, 1H, D2O-exchangeable, NH); MS m/z (%) 420 (M+, 5.2), 419 (7.9), 374 (2.5), 373 (6.4), 339 (100.0), 291(13.7), 257 (60.7), 185 (52.8), 77 (85.2). Anal. Calcd for C22H20N4O3S: C, 62.84; H, 4.79; N, 13.32%. Found: C, 62.80; H, 4.84; N, 13.28%.
Reaction of ethyl 4,6-dimethyl-2-oxo-1,2-dihydropyrimidine-5-carboxylate (2) with aromatic aldehydes
General procedure:
A mixture of the appropriate aromatic aldehyde (20 mmol), the pyrimidine derivative 2 (1.96 g, 10 mmol) and zinc chloride (1 mmol) was heated at 100 º C with constant stirring for 1 h. After all starting material was consumed (TLC), the solution was cooled to ambient temperature. The precipitated crude product was purified by recrystallization from EtOH / DMF to afford the corresponding bis-arylidene derivative 13a-c.
Ethyl 2-oxo-4,6-distyryl-1,2-dihydropyrimidine-5-carboxylate (13a): Yield (70%), mp 213 ºC; IR (KBr): 1623 (C=O), 1711 (C=O), 3024 (NH) cm-1; 1H NMR (DMSO-d6) δ 1.28 (t, 3H, CH3, J = 7.2 Hz), 4.57 (q, 2H, CH2, J = 7.2 Hz), 6.95 (d, 2H, J = 15.85 Hz), 7.45-7.61 (m, 10H), 7.83 (d, 2H, J = 15.85 Hz), 12.10 (s, br., 1H, D2O-exchangeable NH); MS m/z (%) 373 (16.7), 372 (M+, 44.2), 371 (54.3), 343 (100.0), 299 (73.2), 254 (12.3), 130 (16.0), 77 (24.2). Anal. Calcd for C23H20N2O3: C, 74.18; H, 5.41; N, 7.52%. Found: C, 74.10; H, 5.35; N, 7.56%.
Ethyl 4,6-bis(4-methoxystyryl)-2-oxo-1,2-dihydropyrimidine-5-carboxylate (13b): Yield (74%), mp 220 ºC; IR (KBr): 1628 (C=O), 1712 (C=O), 3202 (NH) cm-1; 1H NMR (DMSO-d6) δ 1.33 (t, 3H, CH3, J = 7.2 Hz), 3.81 (s, 6H, OCH3), 4.45 (q, 2H, CH2, J = 7.2 Hz), 7.0-7.05 (m, 6H), 7.60 (d, 4H, J = 8.7 Hz), 7.85 (d, 2H, J = 15.9 Hz), 11.9 (s, br., 1H, D2O-exchangeable NH); MS m/z (%) 432 (M+, 30.4), 403 (100.0), 359 (26.8), 315 (14.3), 285 (39.3), 121 (51.8), 77 (57.1). Anal. Calcd for C25H24N2O5: C, 69.43; H, 5.59; N, 6.48%. Found: C, 69.49; H, 5.50; N, 6.56%.
Ethyl 4,6-bis(4-chlorostyryl)-2-oxo-1,2-dihydropyrimidine-5-carboxylate (13c): Yield (76%), mp > 300 ºC; IR (KBr): 1628 (C=O), 1709 (C=O), 3098 (NH) cm-1; 1H NMR (DMSO-d6) δ 1.29 (t, 3H, CH3, J = 7.2 Hz), 4.41 (q, 2H, CH2, J = 7.2 Hz), 7.27 (d, 2H, J = 15.9 Hz), 7.43-7.6 (m, 11H, Ar-H+NH); MS m/z (%) 368 (10.0), 367 (M+ -EtOCO, 60.0), 174 (30.0), 127 (45.0), 86 (35.0). Anal. Calcd for C23H18Cl2N2O3: C, 62.60; H, 4.11; N, 6.35 %. Found: C, 62.67; H, 4.04; N, 6.30%.
ANTIMICROBIAL EVALUATION
Four of the newly synthesized target compounds were evaluated for their in vitro antibacterial activity against Staphylococcus aureus (RCMB 000106) and Bacillis subtilis (RCMB 000107) as examples of Gram-positive bacteria and Pseudomonas aeruginosa (RCMB 000102) and Escherichia coli (RCMB
000103) as examples of Gram-negative bacteria. They were also evaluated for their in vitro antifungal potential against Aspergillus fumigatus (RCMB 002003), Geotrichum candidum (RCMB 052006), Candida albicans (RCMB 005002) and Syncephalastrum racemosum (RCMB 005003) fungal strains.
Agar-diffusion method was used for the determination of the antibacterial and antifungal activity.
Mean zone of inhibition in mm ± Standard deviation beyond well diameter (6 mm) produced on a range of environmental and clinically pathogenic microorganisms using (10 mg/mL) concentration of tested samples.
The results depicted in Table 1 revealed that most of the tested compounds displayed variable inhibitory effects on the growth of the tested Gram-positive bacteria and Gram-negative bacteria strains and also against fungal strains. In general, most of the tested compounds revealed better activity against the Gram- positive bacteria rather than the Gram-negative bacteria and all compounds exhibited almost and no activity against Syncephalastrum racemosum and Pseudomonas aeruginosa.
Compounds 12 and 13c showed promising results. Substitution at C-4 with phenyl or styryl substituent with electron withdrawing group in para position increased the activity. Also, substitution at C-6 with 1-cyano-2-(phenylamino)-2-thioxoethyl or styryl group increased the activity.
References
1. E. Wyrzykiewicz, G. Bartkowiak, Z. Nowakowska, and B. Kedzia, Farmaco., 1993, 48, 979.
2. P. Sharma, N. Rane, and V. K. Gurram, Bioorg. Med. Chem. Lett., 2004, 14, 4185. CrossRef
3. Y. M. Elkholy and M. A. Morsy, Molecules, 2006, 11, 89. CrossRef
4. B. S. Holla, M. Mahalinga, M. S. Karthikeyan, P. M. Akberali, and N. S. Shetty, Bioorg. Med. Chem., 2006, 14, 2040. CrossRef
5. N. Ingarsal, G. Saravanan, P. Amutha, and S. Nagarajan, Eur. J. Med. Chem., 2007, 42, 517. CrossRef
6. X.-L. Zhao, Y.-F. Zhao, S.-C. Guo, H.-S. Song, D. Wang, and P. Gong, Molecules, 2007, 12, 1136. CrossRef
7. L. Cordeu, E. Cubedo, E. Bandrés, A. Rebollo, X. Sáenz, H. Chozas, M. V. Domínguez, M. Echeverría, B. Mendivil, C. Sanmartin, J. A. Palop, M. Font, and J. García-Foncillas, Bioorg. Med. Chem., 2007, 15, 1659. CrossRef
8. S. M. Sondhi, N. Singh, M. Johar, and A. Kumar, Bioorg. Med. Chem., 2005, 13, 6158. CrossRef
9. K. M. Amin, M. M. Hanna, H. E. Abo-Youssef, and R. F. George, Eur. J. Med. Chem., 2009, 44, 4572. CrossRef
10. N. M. Khalifa, N. S. Ismail, and M. M. Abdulla, Egypt. Pharm. J., 2005, 4, 277.
11. A. Agarwal, Ramesh, Ashutosh, N. Goyal, P. M. S. Chauhan, and S. Gupta, Bioorg. Med. Chem., 2005, 13, 6678. CrossRef
12. G. Martinelli, C. Papayannidis, I. Iacobucci, S. Soverini, D. Cilloni, and M. Baccarani, Hematology Reviews, 2009, 1, 1. CrossRef
13. E. S. Darwish, N. A. Kheder, and A. M. Farag, Heterocycles, 2010, 81, 2247. CrossRef
14. Y. N. Mabkhot, N. A. Kheder, and A. M. Farag, Heterocycles, 2010, 81, 2369. CrossRef
15. A. M. Farag, K. A. K. Ali, T. M. A. Eldebss, A. S. Mayhoub, A. E. Amr, N. A. Abdel-Hafez, and M. M. Abdulla, Eur. J. Med. Chem., 2010, 45, 5887. CrossRef
16. A. M. Farag, A. S. Mayhoub, T. M. A. Eldebss, A. E. Amr, K. A. K. Ali, N. A. Abdel-Hafez, and M. M. Abdulla, Arch. Pharm. Chem. Life Sci., 2010, 343, 384. CrossRef
17. N. A. Kheder, E. S. Darwish, and K. M. Dawood, Heterocycles, 2009, 78, 177. CrossRef
18. N. A. Kheder, Y. N. Mabkhot, and A. M. Farag, Heterocycles, 2009, 78, 937. CrossRef
19. N. A. Kheder, Heterocycles, 2009, 78, 1281. CrossRef
20. A. M. Farag, N. A. Kheder, and Y. N. Mabkhot, Heterocycles, 2009, 78, 1787. CrossRef
21. P. Biginelli, Gazz. Chim. Ital., 1893, 23, 36.
22. K. Folkers, H. J. Harwood, and T. B. Johnson, J. Am. Chem. Soc., 1932, 54, 3751. CrossRef
23. A. Puchala, F. Belaj, J. Bergman, and C. O. Kappe, J. Heterocycl. Chem., 2001, 38, 1345. CrossRef
24. G. Zigeuner, H. Hamberger, H. Blaschke, and H. Sterk, Monatsh. Chem., 1966, 97, 140.
25. A. R. Katritzky and D. Motherhack, J. Chem. Soc., Perkin Trans. 1., 1976, 909. CrossRef
26. E. Alcalde, L. Perez Garcia, G. Miravitlles, J. Rius, and E. Valenti, J. Org, Chem., 1992, 57, 4829.
27. N. A. Kheder, Y. N. Mabkhot, and A. M. Farag, Heterocycles, 2008, 75, 887. CrossRef
28. N. A. Kheder, Y. N. Mabkhot, and A. M. Farag, ARKIVOC, 2008, xvii, 107.