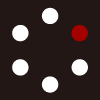
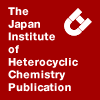
HETEROCYCLES
An International Journal for Reviews and Communications in Heterocyclic ChemistryWeb Edition ISSN: 1881-0942
Published online by The Japan Institute of Heterocyclic Chemistry
e-Journal
Full Text HTML
Received, 24th November, 2010, Accepted, 6th January, 2011, Published online, 18th January, 2011.
DOI: 10.3987/COM-10-12109
■ Palladium-Catalyzed Amination of 2-Chloro-1-azaazulene with 2-Aminopyridine
Mitsunori Oda,* Daisuke Miyawaki, Naoko Matsumoto, and Shigeyasu Kuroda
Department of Chemistry, Faculty of Science, Shinshu University, Asahi, Matsumoto 390-8621, Japan
Abstract
Palladium-catalyzed amination of 2-chloro-1-azaazulene with 2-aminopyridine was studied under various conditions in order to obtain N-(1-azaazulen-2-yl)-N-(2-pyridyl)amine (4) and N,N-bis(1-azaazulen-2-yl)-N-(2-pyridyl)amine (5) as a multidentate ligand. Results of the reaction and physical and chemical properties of 4 and 5 are described.INTRODUCTION
Recently Abe et al. reported palladium-catalyzed amination1 of ethyl 2-chloro-1-azaazulne-3-carboxylate (1) with heteroarylamines leading to various 2-heteroarylamino-1-azaazulenes (Scheme 1), some of which showed anticancer activity.2 Since oxidative insertion of palladium metal to aryl-halogen bonds is activated by an electron-withdrawing group on the aryl ring,3 the ethoxycarbonyl group of 1 may play an import role for its effective amination. Indeed, we reported that 2-chloro-1-azaazulene (2), in which there is no electron-withdrawing substituent, indicated declined reactivity compared with 2-bromo- and 2-iodo-1-azaazulenes in palladium-catalyzed cross couplings, particularly no reactivity in the Suzuki–Miyaura coupling.4 Meanwhile, 1-azaazulene derivatives directly connected with a 2-pyridyl group have been attracted as a ligand in complexation with proton and metal cations.4–7 We reported that 2-(2-pyridyl)-1-azaazulene (3) showed relatively stronger basicity compared with 2,2’-bipyridyl and exhibited the pH- and cationic metal-dependent emission.4 In order to investigate further ability of 1-azaazulenes as a ligand in complexation with proton and metal cations and also to characterize their structural and spectroscopic features in the complexation, we were interested in N-(1-azaazulen-2-yl)-N-(2-pyridyl)amine (4)8 and N,N-bis(1-azaazulen-2-yl)-N-(2-pyridyl)amine (5), whose amino groups connecting with 1-azaazulene and pyridine rings may donate their electrons to contribute to tight complexation. In this paper, we describe details of their synthesis directly from 2 and physical and chemical properties of 4 and 5.
RESULTS AND DISCUSSION
The palladium-catalyzed amination of 2 with ca. one or a half equivalent of 2-aminopyridine was examined under various conditions. Results are shown in Table 1 and Scheme 2 and 3. Compound 4 was obtained in moderate yields by using Pd(OAc)2/XPhos/NaOBu-t and Pd(dba)2/QPhos/NaOBu-t (entry 1 and 5) and in poor yields by using PdCl2(dppf) /NaOBu-t and Pd(dba)2/(t-Bu)3PHBF4/NaOBu-t (entry 2 and 3).9 Under the conditions with Pd(dba)2/TrippyPhos/NaOBu-t (entry 4), 4 was obtained in 23% yield, accompanied with 5 and 1-(1-azaazulen-2-yl)-1-azaazulan- 2-one (6). Since t-BuOH/water was used as solvent under the conditions of entry 4, formation of 6 may arise from 1-azaazulan-2-one, which can be formed by hydrolysis of 2. The best yield of 4 was found under the conditions with Pd(dba)2/XantPhos/Cs2CO3 (entry 6), accompanied with 5 in 7% yield, similarly as reported by Abe et al.2 Although 5 was found in low yields under some of the conditions (entry 4 and 6) in Table 1, however, decreasing the amount of 2-aminopyridine to a half equivalent to 2 resulted in only slight increase of 5 under the similar conditions even in prolonged reaction time. In order to synthesize 5 efficiently, further reaction conditions with various Pd/ligand/solvent systems were surveyed. It was found that 5 was obtained in 65% yield by using Pd(OAc)2/BINAP/t-BuONa/toluene, accompanied with N,N-{1,2’-bi(1-azaazulen)-2-ylidene}-N-2-pyridylamine (7) in 10% yield (entry 9).
Structures of 4, 5, 6, and 7 were characterized by spectroscopic and elemental analyses. UV-Vis spectra of 3, 4, and 5 in ethanol are shown in Figure 1. The longest-wavelength absorption maxima of 4 and 5 were observed at 454 and 488 nm, respectively, indicating a hypsochromic shift and a large hyperchromic effect compared with that of 3 at 512 nm. Both 4 and 5 showed pH-dependent absorption changes, from which pKa values in the first and second dissociation from the diprotonated amines were determined (Table 2). As expected, both pKa1 and pKa2 values of 4 are greater than those of 3.4 The pKa2 value of 5 is the same as that of 3 but the pKa1 value is greater than that of 3. The absorption maxima of 4 and 5 in 50% H2SO4, where both compounds appear to be fully protonated, were observed at 427 and 440 nm, respectively. It is worthy to note that 4 and 5 in 50% H2SO4 exhibited emissions of 491 and 485 nm upon excitation at the absorption maxima with quantum yields of 5.1 and 8.5%, respectively (Figure 2), which are greater than that of 3 (0.41%).4 Both absorptions of 4 and 5
changed depending on metal cations present in the solutions. While the absorption of 4 in the presence of a large excess of monovalant metal cations in acetonitrile showed a very slight change, the absorption in the presence of divalant metal cations displayed a clear hyperchromic effect (Figure 3, left). Among the divalant metal cations examined, Ca2+ ion granted the greatest hyperchromic effect to 4, suggesting that 4 could be applied for detecting Ca2+ ion. On the other hand, the absorption of 5 in the presence of a large excess of divalent metal cations in acetonitrile showed a large hyperchromic effect with all metal ions examined except Hg2+ ion (Figure 3, right). The absorption of 5 in the presence of Hg2+ ion was conferred with the greatest hypsochromic shift by 39 nm and rather a slight hyperchromic effect. The solutions of 5 in the presence of divalant metal cations exhibited emission upon excitation at the longest-wavelength absorption maxima. Among them, the emission spectrum of 5 in the presence of Zn2+ ion was endowed with the greatest emission quantum yield (3.0%, Figure 4), which is greater than that of 5 without metal ion by 230 times.
CONCLUSION
N-azaazulenyl-N-pyridylamines, 4 and 5, were synthesized by palladium-catalyzed amination directly from 2-chloro-1-azaazulene (2). Compound 4 shows stronger basicity than that of 3. In strong acidic media, 4 and 5 exhibited relatively strong emission. The absorption and emission spectra of 4 and 5 clearly changed in the presence of certain metal cations, suggesting that these compounds could be applied somehow or other for sensing metal cations.
EXPERIMENTAL
Melting points were measured on a Yanaco MP-3. IR spectra were recorded on a JEOL Diamond-20 spectrometer. UV spectra were measured on a Shimadzu UV-2550 spectrometer. Emission spectra were recorded on a Shimadzu RF-5300PC spectrometer. 1H and 13C-NMR spectra were recorded with tetramethylsilane as internal standard on a JEOL λ400 NMR instrument. Mass spectra were measured on a JMS-700 mass spectrometer. pH was measured with a TPX-90Si pH meter of TOKO Chem. Lab. Co. Column chromatography was done with Silica gel 60N from Kanto Chem., Inc. XPhos, QPhos, Pd(dba)2, Pd2(dba)3, TrippyPhos, PdCl2(dppf), and XantPhos were purchased from Sigma-Aldrich Japan, Inc. Pd(OAc)2 and 2-aminopyridine were purchased from Wako Chem. Co. (t-Bu)3PHBF4 was prepared according to a literature procedure.10 Compound 2 was prepared from 2-aminotropone by the Nozoe’s method.11 Emission quantum yields were determined by comparison of a total emission area with that of anthracene (Φ = 0.27, upon excitation at 356 nm in ethanol). The refractive indices, n = 1.37 (ethanol), n = 1.39 (50%H2SO4), and n = 1.35 (acetonitrile), were used in correction based on the refractive index.
A typical procedure of amination reactions
A mixture of 2, 2-aminopyridine, palladium/ligand, and base in solvent (2.5 mL to 1 mmol of 2) was evacuated and replaced with argon gas (five times). This mixture was heated in an oil bath under argon atmosphere using a balloon. The reaction was monitored by TLC analysis and the resulted reaction mixture was poured into 20 mL of 10% EDTA-NaHCO3 solution and extracted three times with 20 mL of chloroform. The combined organic layer was washed with brine and was dried with MgSO4. The solvent was removed and the residue was purified by silica gel chromatography to give following products.
N-(1-azaazulen-2-yl)-N-(2-pyridyl)amine (4): Red prisms, mp 190-191 °C; 1H NMR (CDCl3) δ = 6.94 (ddd, J = 7.4, 5.2, 0.8 Hz, 1H), 7.44-7.51 (m, 2H), 7.54 (d, J = 8.4 Hz, 1H), 7.55 (m, 2H), 7.66 (ddd, J = 8.4, 7.4, 2.0 Hz, 1H), 8.16 (m, 1H), 8.19 (d, J = 9.6 Hz, 1H), 8.40 (ddd, J = 5.2, 2.0, 0.8 Hz, 1H), 10.71 (brs, NH) ppm; 13C NMR (CDCl3) δ = 102.1, 112.1, 117.0, 128.4, 129.3, 129.9, 130.1, 131.8, 137.9, 148.0, 148.1, 153.6, 157.2, 163.2 ppm; IR (KBr) νmax = 3431w, 1610s, 1593m, 1537m, 1512s, 1477s, 1450s, 1439s, 1417s, 1309w, 1238w, 877w, 771w, 737w cm–1; UV-Vis (EtOH) λmax = 217 (logε = 4.16), 266 (4.42), 280 (4.44), 334 (4.35), 387 (4.10), 395 (4.09), 454 (3.76), 475sh (3.68), 507sh (3.22) nm; UV-Vis (MeCN) λmax = 217 (logε = 4.20), 265sh (4.43), 281 (4.48), 332 (4.38), 380 (4.17), 393 (4.16), 455 (3.68), 479sh (3.60), 511sh (3.16) nm; MS (70 eV) m/z (rel int) 221 (M+, 11), 220 (9), 168 (16), 167 (100), 166 (28), 165 (60), 152 (25), 146 (10), 139 (8), 119 (8), 115 (8), 105 (8), 92 (10), 91 (6), 90 (12), 89 (6), 77 (15), 65 (13), 63 (11), 51 (10). Anal. Calcd for C14H11N3·0.25H2O: C, 74.48; H, 5.13; N, 18.61%. Found: C, 74.34; H, 5.06; N, 18.45%.
N,N-bis(1-azaazulen-2-yl)-N-(2-pyridyl)amine (5): Red microcrystals, mp 198-199 °C; 1H NMR (CDCl3) δ = 7.37 (s, 2H), 7.39 (ddd, J = 7.6, 4.8, 2.0 Hz, 1H), 7.48 (m, 2H), 7.56 (m, 2H), 7.57 (d, J = 8.0 Hz, 1H), 7.60 (td, J = 10.0, 1.6 Hz, 2H), 7.92 (tdd, J = 7.6, 2.0, 0.8 Hz, 1H), 8.22 (d, J = 10.0 Hz, 2H), 8.32 (ddd, J = 8.8, 1.6, 0.8 Hz, 2H), 8.70 (ddd, J = 4.8, 2.0, 0.8 Hz, 1H) ppm; 13C NMR (CDCl3) δ = 105.1, 122.8, 123.0, 129.1, 129.9, 131.6, 131.7, 133.5, 138.8, 147.2, 149.8, 155.9, 156.9, 164.9 ppm; IR (KBr) νmax = 3074w, 3031w, 1587m, 1516m, 1465s, 1439s, 1433s, 1410s, 1367m, 1334m, 1288w, 1217w, 1039w, 894w, 848w, 796m, 773m, 738m cm–1; UV-Vis (EtOH) λmax = 215 (logε = 4.43), 260 (4.63), 280 (4.59), 299sh (4.47), 338 (4.41), 390sh (4.22), 408 (4.42), 470sh (4.09), 488 (4.13), 517sh (3.93) nm; UV-Vis (MeCN) λmax = 215 (logε = 4.43), 260 (4.63), 282 (4.60), 299sh (4.47), 339 (4.41), 390sh (4.22), 411 (4.43), 468sh (4.09), 489 (4.13), 519sh (3.92) nm; MS (70 eV) m/z (rel int) 349 (M++1, 28), 348 (M+, 100), 347 (86), 271 (12), 270 (37), 269 (20), 268 (10), 244 (19), 243 (13), 242 (10), 230 (21), 220 (23), 219 (15), 218 (12), 128 (10), 127 (10), 102 (30), 101 (16), 78 (53), 77 (23), 51 (20). Anal. Calcd for C23H16N4: C, 79.29; H, 4.63; N, 16.08%. Found: C, 79.04; H, 4.72; N, 15.85%.
1-(1-Azaazulen-2-yl)-1-azaazulan-2-one (6): Light red microcrystals, mp 138-139 °C; 1H NMR (CDCl3) δ = 6.15 (s, 1H), 6.90 (dd, J = 11.0, 8.6 Hz, 1H), 7.04 (dd, J = 11.0, 8.6 Hz, 1H), 7.14 (t, J = 10.0 Hz, 1H), 7.48 (d, J = 11.2 Hz, 1H), 7.68 (d, J = 9.6 Hz, 1H), 7.78 (t, J = 9.4 Hz, 1H), 7.85 (t, J = 9.8 Hz, 1H), 8.08 (s, 1H), 8.59 (d, J = 9.6 Hz, 1H), 8.66 (d, J = 9.6 Hz, 1H), 8.85 (d, J = 9.6 Hz, 1H) ppm; 13C NMR (CDCl3) δ = 104.2, 108.7, 117.5, 129.2, 129.3, 129.7, 130.3, 132.5, 132.7, 135.0, 135.3, 136.6, 143.6, 146.8, 148.5, 155.2, 158.2, 168.4 ppm; IR (KBr) νmax = 3074w, 3031w, 1687s, 1664s, 1662m, 1589s, 1539m, 1495s, 1464s, 1441s, 1410s, 1334m, 1310m, 1290m, 1252m, 1165w, 1064w, 883w, 825w, 791m, 741m, 706m cm-1; UV-Vis (MeCN) λmax = 212 (logε = 4.41), 237 (4.39), 253 (4.50), 284 (4.59), 311 (4.52), 348 (4.29), 396 (3.90), 418 (3.93), 470 (3.68) nm; MS (70 eV) m/z (rel int) 272 (M+, 19), 244 (18), 242 (18), 207 (25), 149 (35), 116 (16), 97 (24), 69 (81), 57 (100). Anal. Calcd for C18H12N2O: C, 79.39; H, 4.44; N, 10.29%. Found: C, 79.19; H, 4.70; N, 10.17%.
N,N-{1,2’-bi(1-azaazulen)-2-ylidene}-N-2-pyridylamine (7): Dark red microcrystals, mp 163-165 °C; 1H NMR (CDCl3) δ = 6.56 (ddd, J = 11.0, 8.4, 0.6 Hz, 1H), 6.72 (s, 1H), 6.75 (dd, J = 11.2, 8.4 Hz, 1H), 6.84 (ddd, J = 11.0, 9.4, 0.8 Hz, 1H), 6.92 (ddt, J = 7.2, 5.2, 0.8 Hz, 1H), 7.02 (dd, J = 8.0, 0.6 Hz, 1H), 7.13 (d, J = 11.2 Hz, 1H), 7.60 (dddd, J = 8.0, 7.2, 2.0, 0.8 Hz, 1H), 7.65 (t, J = 9.8 Hz, 1H), 7.77 (t, J = 9.8 Hz, 1H), 7.83 (t, J = 9.8 Hz, 1H), 8.09 (dd, J = 9.4, 0.6 Hz, 1H), 8.14 (s, 1H), 8.42 (ddt, J = 5.2, 2.0, 0.8 Hz, 1H), 8.57 (d, J = 9.8 Hz, 1H), 8.66 (d, J = 9.8 Hz, 1H) ppm; 13C NMR (CDCl3) δ = 103.8, 110.8, 114.3, 117.7, 118.7, 128.52, 128.54, 128.9, 129.6, 132.3, 132.8, 135.2, 135.4, 136.7, 137.4, 146.47, 146.53, 146.7, 148.4, 155.2, 158.5, 159.9, 162.6 ppm; IR (KBr) νmax = 2924w, 2852w, 1620m, 1579s, 1550m, 1499m, 1468m, 1458m, 1442s, 1423s, 1408m, 1338w, 1315w, 1265m, 1172w, 1144w, 1034w, 885w, 868w, 800w, 739w, 704w cm–1; UV-Vis (MeCN) λmax = 214 (logε = 4.40), 280 (4.61), 310sh (4.42), 354sh (4.19), 442 (4.23) nm; MS (70 eV) m/z (rel int) 349 (M++1, 25), 348 (M+, 100), 347 (61), 271 (10), 270 (38), 269 (28), 268 (13), 244 (26), 243 (23), 242 (16), 231 (13), 230 (25), 218 (17), 102 (17), 78 (55), 77 (13), 51 (18). HRMS m/z Calcd for C23H16N4(M+) 348.1375, found : 348.1343.
Determination of pKa values
The pKa values of 4 and 5 were determined from titration curves based on pH-dependent absorption spectra in 50% aqueous ethanol solutions by a curve fitting method using KaleidaGraph program. For 4, the absorption peak at 435 nm was used, and for 5 at 479 nm. Buffer solutions used are as follows; AcOH/AcONa at a range of pH 4.35–7.40 and KH2PO4/Na2HPO4 at a range of pH 6.97–8.92.
References
1. For palladium-catalyzed aminations, see following reviews; J. P. Wolfe, S. Wagaw, J.-F. Marcoux, and S. L. Buchwald, Acc. Chem. Res., 1998, 31, 805; CrossRef J. F. Hartwig, Acc. Chem. Res., 1998, 31, 852; CrossRef J. F. Hartwig, Angew. Chem. Int. Ed., 1998, 37, 2046. CrossRef
2. K. Koizumi, K. Shimabara, A. Takemoto, S. Yamazaki, N. Yamauchi, H. Fujii, M. Kurosawa, T. Konakahara, and N. Abe, Heterocycles, 2009, 79, 319. CrossRef
3. I. B. Campbell, “Organocopper Reagents”, ed. by R. J. K. Taylor, Oxford University Press, Oxford, 1994, ch. 10, pp. 217-235.
4. M. Oda, K. Ogura, G. Chung Thanh, S. Kishi, S. Kuroda, K. Fujimori, T. Noda, and N. Abe, Tetrahedron Lett., 2007, 48, 4471. CrossRef
5. N. Abe, E. Hashimoto, H. Fujii, Y. Murakami, S. Tagashira, and A. Kakehi, Heterocycles, 2004, 63, 2341. CrossRef
6. N. Abe, M. Tanaka, T. Maeda, H. Fujii, and A. Kakehi, Heterocycles, 2005, 66, 229. CrossRef
7. T. Ariyoshi, T. Noda, S. Watarai, S. Tagashira, Y. Murakami, H. Fujii, and N. Abe, Heterocycles, 2009, 77, 565. CrossRef
8. Compound 4 has been synthesized as a deesterified by-product in the reaction of Scheme 1 with 2-aminopyridine. However, its detail of spectroscopic properties is not reported yet. See ref. 2.
9. For trivial names and references of reagents, see followings. XPhos is a trivial name of 2-dicyclohexylphosphino- 2’,4’,6’-triisopropylbiphenyl; X. Huang, K. W. Anderson, D. Zim, L. Jiang, A. Klapars, and S. L. Buchwald, J. Am. Chem. Soc., 2003, 125, 6653; CrossRef QPhos is a trivial name of 1, 2, 3, 4, 5-pentaphenyl-1′-(di-tert-butylphosphino)ferrocene; Q. Shelby, N. Kataoka, G. Mann, and J. F. Hartwig, J. Am. Chem. Soc., 2000, 122, 10718; CrossRef Dppf is 1,1′-bis(diphenylphosphino)- ferrocene; G. Marr and T. Hunt, J. Chem. Soc., C, 1969, 7, 1070; CrossRef Dba is dibenzalacetone. TrippyPhos is a trivial name of 1-{2-(di-tert-butylphosphino)phenyl}-3,5-diphenyl-1H-pyrazole; R. A. Singer, S. Caron, R. E. McDermott, P. Arpin, and M. D. Nga, Synthesis, 2003, 1727; CrossRef XantPhos is a trivial name of 4,5-bis(diphenylphosphino)-9,9′-dimethylxanthene; S. Hillebrand, J. Bruckmann, C. Kriiger, and M. W. Haenel, Tetrahedron Lett., 1995, 36, 75. BINAP is 1,1’-bi{(2-diphenyl- phosphino)naphthyl}. CrossRef
10. M. R. Netherton and G. C. Fu, Org. Lett., 2001, 3, 4295. CrossRef
11. T. Nozoe, S. Seto, S. Matsuura, and T. Terasawa, Chem. Ind., 1954, 1357.