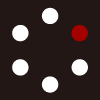
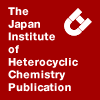
HETEROCYCLES
An International Journal for Reviews and Communications in Heterocyclic ChemistryWeb Edition ISSN: 1881-0942
Published online by The Japan Institute of Heterocyclic Chemistry
e-Journal
Full Text HTML
Received, 6th December, 2010, Accepted, 12th January, 2011, Published online, 12th January, 2011.
DOI: 10.3987/COM-10-12116
■ Resveratrol Dimers with an Oxabicyclo Ring in Vatica albiramis
Naohito Abe, Tetsuro Ito, Masayoshi Oyama, Ryuichi Sawa, Yoshikazu Takahashi, and Munekazu Iinuma*
Laboratory of Pharmacognosy, Gifu Pharmaceutical University, 1-25-4 Daigaku-nishi, Gifu 501-1196, Japan
Abstract
Investigation of the chemical constituents in the stem of Vatica albiramis (Dipterocarpaceae) resulted in the isolation of six stilbenoid derivatives, albiraminols C (1) and D (5), vatalbinosides G (2)–I (4), and J (6). We determined their structures by spectroscopic analysis including two- dimensional NMR, comparison of the NMR data based on isomerism, and computer-aided molecular modeling. Compounds 1–5 had two resveratrol units and are the first instance of resveratrol derivatives bearing an oxabicyclo[3.2.2]nonadiene ring system. Compound 6 is the oxidative product of 2 bearing a carbonyl group.INTRODUCTION
Our previous papers have demonstrated the structural diversity of resveratrol oligomers found in dipterocarpaceous plants and some of their biological properties.1-7 The different condensation degrees of resveratrol by biosynthesis produce the scaffold of resveratrol oligomers with a variety of skeletons and stereoisomerisms in the family.1 Two blocking units of resveratrol produce a variety of skeletons represented by the dihydrobenzofuran, dibenzobicyclo[3.2.1]octadiene, and dibenzobicyclo[3.3.0]octadiene systems. In the course of structural elucidation of some resveratrol oligomers, we established the spectroscopic properties of each skeleton and its stereoisomers due to the accumulation of comparative analysis based on the NMR results. The genus Vatica is rich in resveratrol dimers and tetramers and was our focus for phytochemical study for the expansion of chemical library of resveratrol oligomers.2,8-10 In our previous papers, we discussed the phytochemical study of Vatica albiramis and the structure of its
resveratrol derivatives. We also identified the strong inhibitory effect of MMP-1 production by some cognates.2 Further examination of the extract resulted in isolation of six new resveratrol derivatives, called albiraminols C (1) and D (5), vatalbinosides G (2)–I (4), and J (6), bearing an oxabicyclo[3.2.2]nonadiene system.
RESULTS AND DISCUSSION
Albiraminols C (1) and D (5) and vatalbinosides G (2)–I (4), obtained as pale yellow solids, showed positive reactions with the Gibbs reaction and optical activities. Compounds 1–5 had the same core planar structure of the 10,11-dihydro-5H-10,5-(epoxymethano)dibenzo[a,d][7]annulene skeleton, among which 2–4 are mono-glucosides of 1 or 5. The core skeletons bore three different relative configurations due to two asymmetric carbons (C-7a and C-7b). The planar structural analysis for each was similar; hence, the protocol followed for 1 is described in detail below and comparative results due to stereoisomerism and/or glucosylation are discussed for 1–5.
Albiraminol C (1) showed a [M+Na]+ ion at m/z 493.1258 in the positive ion ESI-MS, attributable to the molecular formula C28H22O7. The 1H-NMR signals analyzed from the DQF-COSY spectrum indicated the presence of two sets of 4-oxygenatedphenyl groups (δH 6.92, 6.63 (2H each, d, J = 8.8 Hz, H-2a(6a), H-3a(5a); ring A1), 7.16, and 6.74 (2H each, d, J = 8.8 Hz, H-2b(6b), H-3b(5b); ring B1)), two sets of 3,5-dioxygenated-1,2-disubstituted benzene rings (δH 6.18, 6.42 (1H each, d, J = 2.4 Hz, H-12a, H-14a; ring A2), 6.24, and 6.50 (1H each, d, J = 2.4 Hz, H-12b, H-14b; ring B2)), and two sets of mutually coupled aliphatic methine protons (δH 5.60, 4.25 (1H each, s, H-7a, H-8a), 4.34, and 5.00 (1H each, d, J =
3.6 Hz, H-7b, H-8b)). The 13C-NMR spectrum displayed 28 carbon signals including 24 aromatics (sp2) and four aliphatics (sp3). The HMQC and HMBC spectra enabled the complete assignment of all protonated and quaternary carbons as shown in Table 1. In the HMBC spectrum (see Figure 1 and Table 2), we observed 3J correlations between H-7a/C-2a(6a), H-8a/C-14a, H-7b/C-2b(6b), and H-8b/C-14b, which confirmed the C–C bonds for C-1a–C-7a, C-8a–C-9a, C-1b–C-7b, and C-8b–C-9b, respectively. These results suggested that 1 was a stilbene dimer composed of two resveratrols A and B (resveratrol A: ring A1–C-7a–C-8a–ring A2). The HMBC correlations between H-8a/C-11b and H-7b/C-11a indicated the connections for C-8a/C-10b and C-7b/C-10a, substantiating the formation of a 10,11-dihydro-5H-dibenzo [a,d][7]annulene skeleton. The unsaturation for 1 (18) and the molecular formula suggested the formation of one ether bond. We unambiguously deduced the 10,11-dihydro-5H-10,5-(epoxymethano)dibenzo-[a,d][7]annulene skeleton formed by the ether (C-7a–O–C-8b) from the HMBC correlation (H-8b/C-7a). The 13C chemical shifts of the oxymethines (δC 81.4 (C-7a), 81.3 (C-8b)) also supported the ring formation. The other six oxygen atoms form hydroxyl groups on the aromatic rings. We then elucidated the planar structure of 1.
The relative stereostructure of 1 was elucidated as follows. The consequent configurations for C-8a and C-8b in the framework of the oxabicyclo ring system were S and R, respectively, and four configurations due to the other two asymmetric centers (C-7a and C-7b) are proposed for 1. Figure 2 represents each configuration after energy minimization using the PCMODEL11 with the Merck molecular force field (MMFF94) (A: (C-7a(S), C-7b(R), B: (C-7a(R), C-7b(R), C: (C-7a(R), C-7b(S), and D: (C-7a(S), C-7b(S)). In the NOESY experiment of 1, we observed NOEs between H-7a/H-2b(6b) and H-7b/H-14b, which can only be explained by A bearing a cofacial orientation of H-7a and a ring B1. Therefore, we elucidated albiraminol C (1) as (5S,10R,11R,13S)-11,13-bis(4-hydroxyphenyl)-10,11-dihydro-5H-10,5-(epoxymethano)dibenzo[a,d][7]annulene-1,3,6,8-tetraol.
We identified the compositions of the vatalbinosides G (2)–I (4) and albiraminol D (5) as C34H32O12 (2–4) and C28H22O7 (5), respectively, from the ESI-MS spectra ((2: [M+Na]+, m/z 655.1783); 3: ([M−H]−, m/z 631.1814); 4: ([M−H]−, m/z 631.1816); and 5: ([M−H]−, m/z 469.1302). As described above, 1–5 have the same planar structure except for a glucopyranosyl group. The presence of one O-β-glucopyranosyl moiety in 2–4 was supported by the NMR spectral data (see Tables 1 and 3). For example, 2 showed typical NMR signals due to a β-glucopyranosyloxy group given by the six carbon signals (δC 102.3, 74.9, 78.1, 71.4, 78.0, and 62.6), an anomeric proton (δH 4.89 (d, J = 7.2 Hz)), and a sequence of methine protons (δH 3.45–3.91). The 1H- and 13C-NMR data of 2 and 3 (see Table 1), except for the β-glucopyranosyloxy group, showed similarity to that of 1. The HMBC and NOESY spectra confirmed that the aglycone of 2
and 3 was 1. The positions of the O-β-glucopyranosyloxy group were fixed by NOEs (C-13b for 2; C-4a for 3). The NMR results confirmed the position of the O-β-glucopyranosyloxy group of 4 as C-13b.
We determined the relative stereostructure of 4 from the key NOEs (H-2a(6a)/H-2b(6b) and H-7b/H-14b), confirming the aglycone as B that had cofacial orientation of the rings A1 and B1. Compound 4 was an epimer of 2 due to C-7a, and the isomerism contributed to the differences in the 1H-NMR data for ring A1, H-7a, and H-8a.
Compound 5 was a diastereomer of 3 and was elucidated as C ((5S,10R,11S,13R)-11,13-bis(4-hydroxyphenyl)-10,11-dihydro-5H-10,5-(epoxymethano)dibenzo[a,d][7]annulene-1,3,6,8-tetraol). In the 1H-NMR spectrum of 5, ring B1 displayed broad singlets due to four aromatic protons (δH 5.74 (1H, H-2b), 8.30 (2H, H-3b and H-5b), and 7.76 (1H, H-6b)), indicating that the ring could not rotate freely. The 13C-NMR spectrum also showed broad signals for C-2b, C-3b, C-5b, and C-6b. We discussed the hindered rotation of the 4-hydroxyphenyl groups in resveratrol oligomers and the NMR properties of
vaticanol G,8 isovaticanol C,9 vateriaphenol A,12 cotylelophenol A,13 and grandiphenol C,14 and concluded that steric hindrance and CH/π interactions contributed to the phenomenon. The ring B1 of 5 was the same case, and the two pairs of sp2-CH groups (CH-2b and CH-6b; CH-3b and CH-5b) were located in a different environment.
The energy minimized model could reasonably explain the anisotropic effect of rings B1 and B2, which caused an upper field shift of the aromatic protons, H-2b (δH 5.74) and H-14b (δH 5.42). The other energy-minimized structures (A, B, and D) could never be suitable for anisotropy based on the configuration and fixation of ring B1.
We isolated vatalbinoside J (6) as a brown solid that exhibited a molecular ion peak [M−H]− at m/z 553.1344 in the negative ion ESI-MS corresponding to the molecular formula of C28H26O12. Analysis of 1H− and 13C-NMR data (see Table 3) revealed the presence of a 4-oxygenatedphenyl group (B1), two sets of 3,5-dioxygenated-1,2-disubstituted benzene rings (rings A and B2), a mutually coupled aliphatic methine proton (H-7b/H-8b), an O-β-glucopyranosyl unit, and a carbonyl group (δC 175.7 (C-1a)). The connection of partial structures was established as follows (see Figure 1). The significant HMBC
correlations observed between H-2a/C-8a, H-2a/C-1a, H-2a/C-11b, H-7b/C-5a, H-7b/C-2b(6b), and H-8b/C-14b indicated C–C bonds between C-2a–C-3a, C-2a–C-1a, C-2a–C-10b, C-7b–C-4a, C-7b–C-1b, and C-8b–C-9b, respectively. The ether linkage C-1a–O–C-8b was supported by the cross peak H-8b/C-1a. Long range correlations between the anomeric proton (δH 4.89) and the carbon (δC 159.4) in the HMBC spectrum confirmed that the glucosyloxy group was at C-13b. Therefore, we elucidated the planar structure of 6, which would be produced by an oxidative elimination of ring A1 from 2 or 4. The lack of rings caused difficulty in determination of the stereostructure of 6, because the configurational elucidation of C-7b of 1–5 were elucidated by NOEs from aromatic protons on ring B1. In the case for 6, the accumulated NMR data for 1–5 solved the problem. In particular, the S configuration of C-7b resulted in anisotropy of the rings B1 and B2 as described for 5 and possessed a larger coupling constant (5: J = 6.0 Hz) than those of opposite configurations (1–4: J ≤ 3.6 Hz; see Tables 1 and 2). The NMR data of 6 were suitable for those of the S configuration of C-7b. Therefore, vatalbinoside J (6) was elucidated as (5S,10R,11R)-8-glucopyranosyloxy-1,3,6-trihydroxy-11-(4-hydroxyphenyl)-10,11-dihydro-5H-10,5-(epoxy-methano)dibenzo[a,d][7]annulen-13-one.
Oligostilbenoids are rich in structural isomers and stereoisomers that are composed of a simple blocking unit, resveratrol. In recent years, we reported that the smallest oligomerization degree groups of resveratrol dimers in dipterocarpaceaeous plants comprise various heterocyclic ring systems represented by dihydrobenzofuran, bicyclo[3.2.1]octadiene, bicyclo[5.3.0]decadiene, and bicyclo[3.3.0]octadiene, and bear stereoisomerisms of diastereomers, epimers, and enantiomers.1 In this study, we discovered an oxabicyclo[3.2.2]nonadiene system (dihydro-5H-10,5-(epoxymethano)dibenzo[a,d][7]annulen) as resveratrol dimers for the first time. Although an isolation of D was not achieved, three isomerisms (A (1–3), B (4), and C (5)) of the core skeleton yielded a variation of stereoisomers and their comparative NMR database.
EXPERIMENTAL PROCEDURES
General method
The following instruments were used: optical rotations, JASCO P-1020 polarimeter; UV spectra, Shimadzu UV-3100 spectrophotometer (in methanol solution); CD spectra, JASCO J-820 spectrometer (in MeOH solution); 1H- and 13C- NMR spectra, JEOL JNM AL-400 (chemical shift values in 1H-NMR spectra are presented as δ values with TMS as an internal standard); and ESI-MS, Thermo Fisher Scientific LTQ Orbitrap instrument and Shimadzu LCMS-IT-TOF.
The following adsorbents were used for purification: analytical TLC, Merck Kieselgel 60 F254 (0.25 mm); preparative TLC, Merck Kieselgel 60 F254 (0.5 mm); CC, Merck Kieselgel 60, Pharmacia Fine Chemicals AB Sephadex LH-20, and Fuji Silysia Chemical, Waters Sep-Pak C18 cartridges; VLC, Merck Kieselgel 60; medium pressure column chromatography (MPLC), Fuji Silysia Chemical Chromatorex ODS (100-200 mesh); preparative HPLC; Capcell Pak C18 column (UG120, 5 μm, 10 mm i.d. × 250 mm, SHISEIDO, Japan), Shimadzu 6A pump, Shimadzu SPD-10A UV-VIS detector, SPD-M10Avp UV-VIS diode array detector.
Plant material
Vatica albiramis was collected in Borneo, Malaysia, in April 2002 with an identification by J. Josue, head of the forest product branch at the Forest Research Center, Sandakan Sabah, Malaysia. A voucher specimen number DP-026 was deposited at the herbarium of Gifu Pharmaceutical University.
Extraction and isolation
The extraction and isolation procedures were the same as those discussed in our previous report.2 Compounds 1 (16.7 mg) and 5 (15.7 mg) were obtained from Fraction 4 after purification by CC with Sephadex LH-20 (MeOH), Sep-Pak C18 (MeOH/H2O system). Fraction 9 was further purified with silica gel CC (CHCl3/MeOH gradient system), Sephadex LH-20 CC (MeOH), Sep-Pak C18 CC (MeOH/H2O system), VLC (EtOAc/CHCl3/MeOH/H2O system), reversed phase MPLC (MeOH/H2O system), and preparative HPLC (MeCN/H2O system) to give 2 (18.0 mg), 3 (11.9 mg), 4 (8.9 mg), and 6 (3.9 mg).
Albiraminol C ((5S,10R,11R,13S)-11,13-bis(4-hydroxyphenyl)-10,11-dihydro-5H-10,5-(epoxymethano)-dibenzo[a,d][7]annulene-1,3,6,8-tetraol) (1): Pale yellow solid. [α]D –29.0° (c 0.1, MeOH). UV (log ε, MeOH): 281 (4.22). CD (c 53.2 µM, MeOH) nm (Δε): 217 (−28.7), 247 (+12.9). 1H-NMR (acetone-d6, 400 MHz) and 13C-NMR (acetone-d6, 100 MHz): see Table 1. ESI-MS (pos.): m/z 493 ([M+Na]+). HR-ESI-MS (pos.): m/z 493.1258 ([M+Na]+, C28H22O7Na+; calc. 493.1258).
Vatalbinoside G ((5S,10R,11R,13S)-8-(4-(β-glucopyranosyloxy)phenyl)-11,13-bis(4-hydroxyphenyl)-10,11-dihydro-5H-10,5-(epoxymethano)dibenzo[a,d][7]annulene-1,3,6-triol) (2): Pale yellow solid. [α]D −15.1° (c 0.1, MeOH). UV (log ε, MeOH): 281 (4.30). CD (c 39.0 µM, MeOH) nm (Δε): 217 (−53.4), 244 (+20.9). 1H-NMR (methanol-d4, 400 MHz) and 13C-NMR (methanol-d4, 100 MHz): see Table 1. ESI-MS (pos.): m/z 655 ([M+Na]+). HR-ESI-MS (pos.): m/z 655.1783 ([M+Na]+, C34H32O12Na+; calc. 655.1786).
Vatalbinoside H ((5S,10R,11R,13S)-13-(4-(β-glucopyranosyloxy)phenyl)-11-(4-hydroxyphenyl)-10,11-dihydro-5H-10,5-(epoxymethano)dibenzo[a,d][7]annulene-1,3,6,8-tetraol) (3): Pale yellow solid. [α]D –6.1˚ (c 0.1, MeOH). UV (log ε, MeOH): 281 (4.01). CD (c 39.0 µM, MeOH) nm (Δε): 218 (−32.6), 246 (+14.9). 1H-NMR (methanol-d4, 400 MHz) and 13C-NMR (methanol-d4, 100 MHz): see Table 1. ESI-MS (neg.): m/z 631 ([M−H]−). HR-ESI-MS (neg.): m/z 631.1814 ([M−H]−, C34H31O12−; calc. 631.1821).
Vatalbinoside I ((5S,10R,11R,13R)-8-(4-(β-glucopyranosyloxy)phenyl)-11,13-bis(4-hydroxyphenyl)-10,11-dihydro-5H-10,5-(epoxymethano)dibenzo[a,d][7]annulene-1,3,6-triol) (4): Pale yellow solid. [α]D –95.9˚ (c 0.1, MeOH). UV (log ε, MeOH): 279 (4.06). CD (c 39.0 µM, MeOH) nm (Δε): 214 (−42.2). 1H-NMR (methanol-d4, 400 MHz) and 13C-NMR (methanol-d4, 100 MHz): see Table 3. ESI-MS (neg.): m/z 631 ([M−H]−). HR-ESI-MS (neg.): m/z 631.1816 ([M−H]−, C34H31O12−; calc. 631.1821).
Albiraminol D ((5S,10R,11S,13R)-11,13-bis(4-hydroxyphenyl)-10,11-dihydro-5H-10,5-(epoxymethano)-dibenzo[a,d][7]annulene-1,3,6,8-tetraol) (5): Pale yellow solid. [α]D +4.4˚ (c 0.1, MeOH). UV (log ε, MeOH): 279.5 (4.01). CD (c 53.2 µM, MeOH) nm (Δε): 216 (−39.6), 246 (+10.4). 1H-NMR (acetone-d6, 400 MHz) and 13C-NMR (acetone-d6, 100 MHz): see Table 3. ESI-MS (neg.): m/z 469 ([M−H]−). HR-ESI-MS (neg.): m/z 469.1302 ([M−H]−, C28H21O7−; calc. 469.1293).
Vatalbinoside J ((5S,10R,11R)-8-(4-(β-glucopyranosyloxy)phenyl)-1,3,6-trihydroxy-11-(4-hydroxy-phenyl)-10,11-dihydro-5H-10,5-(epoxymethano)dibenzo[a,d][7]annulen-13-one) (6): Pale yellow solid. [α]D +16.1˚ (c 0.1, MeOH). UV (log ε, MeOH): 284.5 (4.06). CD (c 45.0 µM, MeOH) nm (Δε): 213 (−19.0) , 229 (+6.3), 246.3 (+9.5). 1H-NMR (methanol-d4, 400 MHz) and 13C-NMR (methanol-d4, 100 MHz): see Table 3. ESI-MS (neg.): m/z 553 ([M−H]−). HR-ESI-MS (neg.): m/z 553.1344 ([M−H]−, C28H25O12−; calc. 553.1352).
References
1. T. Ito, Yakugaku Zasshi, 2011, 131, 93. CrossRef
2. N. Abe, T. Ito, K. Ohguchi, M. Nasu, Y. Masuda, M. Oyama, Y. Nozawa, M. Ito, and M. Iinuma, J. Nat. Prod., 2010, 73, 1499. CrossRef
3. T. Ito, Y. Akao, H. Yi, K. Ohguchi, K. Matsumoto, T. Tanaka, M. Iinuma, and Y. Nozawa, Carcinogenesis, 2003, 24, 1489. CrossRef
4. T. Ito, T. Tanaka, Z. Ali, Y. Akao, Y. Nozawa, Y. Takahashi, R. Sawa, K. Nakaya, J. Murata, D. Darnaedi, and M. Iinuma, Heterocycles, 2004, 63, 129. CrossRef
5. M. A. Shibata, Y. Akao, E. Shibata, Y. Nozawa, T. Ito, S. Mishima, J. Morimoto, and Y. Otsuki, Cancer Chemother. Pharmacol., 2007, 60, 681. CrossRef
6. Y. Tabata, K. Takano, T. Ito, M. Iinuma, T. Yoshimoto, H. Miura, Y. Kitao, S. Ogawa, and O. Hori, Am. J. Physiol., 2007, 293, C411. CrossRef
7. T. Tsukamoto, R. Nakata, E. Tamura, Y. Kosuge, A. Kariya, M. Katsukawa, S. Mishima, T. Ito, M. Iinuma, Y. Akao, Y. Nozawa, Y. Arai, S. Namura, and H. Inoue, Nutr. Metab., 2010, 7, 46. CrossRef
8. T. Ito, T. Tanaka, K. i. Nakaya, M. Iinuma, Y. Takahashi, H. Naganawa, M. Ohyama, Y. Nakanishi, K. F. Bastow, and K. H. Lee, Tetrahedron, 2001, 57, 7309. CrossRef
9. T. Ito, T. Tanaka, M. Iinuma, I. Iliya, K. i. Nakaya, Z. Ali, Y. Takahashi, R. Sawa, Y. Shirataki, and J. Murata, Tetrahedron, 2003, 59, 5347. CrossRef
10. T. Ito, T. Tanaka, M. Iinuma, K. Nakaya, Y. Takahashi, R. Sawa, J. Murata, and D. Darnaedi, J. Nat. Prod., 2004, 67, 932. CrossRef
11. PCMODEL v 9.0, Serena Software, Box 3076, Bloomington, IN 47402-3076.
12. T. Ito, T. Tanaka, K. i. Nakaya, M. Iinuma, Y. Takahashi, H. Naganawa, M. Ohyama, Y. Nakanishi, K. F. Bastow, and K. H. Lee, Tetrahedron Lett., 2001, 42, 5909. CrossRef
13. T. Ito, Z. Ali, M. Furusawa, I. Iliya, T. Tanaka, K. Nakaya, J. Murata, D. Darnaedi, M. Oyama, and M. Iinuma, Chem. Biodivers., 2005, 2, 1200. CrossRef
14. T. Ito, N. Abe, M. Oyama, T. Tanaka, J. Murata, D. Darnaedi, and M. Iinuma, Helv. Chim. Acta, 2009, 92, 1203. CrossRef