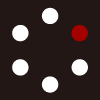
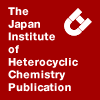
HETEROCYCLES
An International Journal for Reviews and Communications in Heterocyclic ChemistryWeb Edition ISSN: 1881-0942
Published online by The Japan Institute of Heterocyclic Chemistry
e-Journal
Full Text HTML
Received, 16th December, 2010, Accepted, 9th February, 2011, Published online, 18th February, 2011.
DOI: 10.3987/COM-10-12124
■ Synthesis and Reactivity of 6H-Dibenzo[c,e][1,2]oxaphosphinine 6-Sulfide, a Novel Thiophosphacyclic Molecule
Muriel Rakotomalala, Sebastian Wagner, Thomas Zevaco, Michael Ciesielski, Olaf Walter, and Manfred Döring*
Institute of Catalysis Research and Technology, Karlsruhe Institute of Technology, Hermann-von-Helmholtz Platz 1, 76344 Eggenstein-Leopoldshafen, Germany
Abstract
The new 6H-dibenzo[c,e][1,2]oxaphosphinine 6-sulfide (1) was synthesized and characterized by 1H-, 13C-, 31P-NMR, IR spectroscopy as well as X-ray diffraction and HR-MS. The reactivity of (1) was investigated with a series of test reactions, including Michael-type additions and the Atherton-Todd-reaction and finally compared to that of the parent 9,10-dihydro-9-oxa-10-phosphaphenanthrene-10-oxide.INTRODUCTION
Phosphorus-containing molecules have been the subject of both academic and industrial interest for several decades.1-3 Many applications for such molecules have been reported in different areas of chemistry such as coordination chemistry, material science, homogeneous catalysis, development of biologically active compounds or pesticides.4-9 Recently, there has been a growing interest from the flame retardant community regarding phosphorus-containing molecules as they are known to impart flame retardancy to a wide range of polymeric materials.10-12 Although a large number of organophosphorous compounds have been reported to be flame retardants, the influence of heteroatoms on the phosphorus environment was scarcely studied. Here, we present the synthesis and reactivity of a new phosphine sulfide molecule whose applications are not solely restricted to flame retardancy.
RESULTS AND DISCUSSION
To the best of our knowledge, 6H-dibenzo[c,e][1,2]oxaphosphinine 6-sulfide (1) had never been synthesized prior to our study. Krawiecki and Michalski showed that dialkyl phosphochloridates react readily with hydrogen sulphide in the presence of tertiary bases to yield tetra-alkyl thiopyrophosphates.13 This method was then successfully used by Zwierzak to obtain a series of cyclic hydrogen thiophosphites.14 Therefore, our first synthetic approach consisted of reacting DOP-Cl and hydrogen sulphide gas as a source of sulphur in the presence of triethylamine (Scheme 1). This successfully yielded the target molecule in quantitative yields. Similar to 9,10-dihydro-9-oxa-10-phosphaphenanthrene-10-oxide (2), the 1H-NMR spectrum of the obtained crystalline white powder showed a doublet in the aromatic region (δ= 8.4 ppm) with a large coupling (1JP-H = 536 Hz) that was associated with a P-H bond (Figure 1). The 31P-NMR spectrum showed a single peak (δ = 57.1 ppm) and the major peak in the mass spectra was at m/z = 232 strongly suggesting that the isolated product was (1). The 1H-NMR spectrum of (1) revealed well resolved signals which can be attributed via 2-D COSY methods involving both H-H (gCOSY, gDQFCOSY) and C-H correlations (gHSQC and gHMBC) (Figure 2).
Slow diffusion of a concentrated solution of (1) in dry dichloromethane into pentane yielded a single crystal that indisputably confirmed the isolation of the target molecule (Figure 3). The structure of (1) is similar to its parent oxide molecule (2), however, (1) has an increased solubility in most organic solvents.15
However, during this synthesis, the use of large amounts of H2S was not desirable, as its toxicity is similar to that of hydrogen cyanide.16 The two most commonly used sources of sulphur are phosphorus pentasulfide and Lawesson reagent.3 The use of such thionation agents solved the toxicity issue and also allowed the use of the commercially available (2) as a starting material rather than DOP-Cl which is highly air- and moisture-sensitive. Both thionation agents successfully yielded the desired molecule, though the yields were lower.
With several practical ways to synthesize molecule (1) in hand, we initiated the comparison of its reactivity with the parent phosphine oxide molecule (2) whose chemistry mainly involves the reactivity of the P-H bond. P-H bonds are known to undergo nucleophilic addition onto unsaturated functionalities such as carbonyl group and acrylates.17 The parent molecule (2) was reported to produce the hydroquinone derivative in very good yield when heated in presence of benzoquinone.18,19 Hence, benzoquinone was chosen as α,β-unsaturated carbonyl to assess the reactivity of (1). When a solution of (1) was heated in the presence of equimolar amounts of benzoquinone, a single product from a Pudovik reaction could be isolated in a high yield (Figure 4).20
Another organophosphorous reaction involving the reactive phosphorous-hydrogen bond in phosphites and phosphonites is the Atherton-Todd reaction.20-24 The Atherton-Todd reaction, usually involving dialkyl and diaryl phosphites in the presence of carbon tetrachloride and a base, is known as an effective way to access functionalised phosphine oxides, in particular phosphoramidates, which are an important and versatile class of chemicals.
However, this reaction remains relatively unexplored for phosphorous sulfides. In an effort to deepen our understanding of its reactivity, (1) was reacted with different nucleophiles using the Atherton-Todd approach as shown in Scheme 2.
With this intention, (1) was dissolved in chloroform and carbon tetrachloride then a solution of triethylamine and a nucleophile diluted in chloroform was added dropwise. The different nucleophiles (phenylethylamine, piperidine and 2-phenylethanol) were chosen as models for primary and secondary amines as well as primary alcohols, respectively. All three desired products were obtained in good yields with fast reaction times (below 60 minutes). Hence, (1) proved to be a very rewarding reagent for the Atherton-Todd reaction. The in situ formation of 10-chloro-9-oxa-10-phospha-phenanthrene 10-sulfide (4) can be observed using NMR spectroscopy (δ (31P, CDCl3) 74.5 ppm) and HR-MS analysis (calculated for [12C12H8OSPCl]+ 265.9722, found 265.9688). Even though primary alcohols are known to be problematic substrates for the Atherton-Todd reaction, the formation of compound (5) was observed in good yields.22 Crystal structures of molecules (5) , (6) and (7) were also obtained and indisputably verify the desired molecules. Figure 5 presents the crystal structures and selected bond lengths and torsion angles. The torsion angles are a sign of delocalisation. The P=S bond length in compound (5) was 191.1 pm (6(P=S): 193.6 pm, 7(P=S): 191.9 pm) which coincides with parent structures.25-27 The aliphatic phosphorus oxygen bond in (5) was 158.5 ppm which fits perfectly with literature.25,28,29 The phosphorus-nitrogen bonds in molecule (6) (162.0 pm) and (7) (163.7 pm) also correspond to those of similar structures.28,29 This set of experiments demonstrated that the Atherton-Todd reaction can be exploited to access different phosphine sulphide molecules.
To summarize our findings, we presented the synthesis of the new 6H-dibenzo[c,e][1,2]oxaphosphinine 6-sulfide (1) (DOPS). Compound (1) can be obtained using different thionation agents and undergoes nucleophilic additions such as the Pudovik reaction as well as the Atherton-Todd reaction. The ease of synthesis of molecule (1) combined with its broad range of reactivity makes it a promising compound for a wide spectrum of molecules and applications.
EXPERIMENTAL
General Experimental:
Unless stated otherwise, solvents and chemicals were obtained from commercial sources and used without further purification. NMR spectra were recorded with a Varian INOVA-400 (400 MHz). Chemical shifts are reported as δ values relative to the solvent peak. Tetramethylsilane was used as a standard. All 31P-NMR spectra are measured proton decoupled. All 13C-NMR spectra were measured proton decoupled and phosphorus coupled. 1H-NMR spectra were measured phosphorus coupled. Melting points are uncorrected and measured with a Büchi B-545. IR spectra were recorded with a Varian 660-IR (FT-IR). High resolution mass spectrometry (HR-MS) analyses were performed on a MicroMass GCT (time of flight (TOF); electron ionization (EI), 70 eV). Elemental analysis was performed using a Vario EL III from Elementar Analysensystem GmbH. Crystal structure analyses were conducted on a Siemens SMART-1000-CCD with Mo-Kα (λ=0.71073, graphite monochromator) at 200 K. A Lorentz and Polarisation correction was applied. Experimental absorption correction were done with SADABS, structure solving and refining was done with SHELX 97. XPMA and Mercury 2.2 were used for visualisation.
Synthesis:
6H-Dibenzo[c,e][1,2]oxaphosphinine 6-sulfide (1): In a flame-dried three-neck round-bottom flask fitted with a gas inlet, a thermometer and a gas outlet leading to a NaOH aqueous solution, H2S gas was bubbled through a solution of DOP-Cl (40.0 g, 171 mmol) in dry toluene (120 mL) at room temperature. The released acid was then trapped with triethylamine (19.0 g, 188 mmol). The suspension was filtered and concentrated in vacuo to yield a colorless powder (35.2 g, 152 mmol, 89%). mp 88 °C; 31P NMR (101 MHz, CDCl3) δ 57.1 ppm; 13C NMR (101 MHz, CDCl3) δ 149.7 (d, J = 11.2 Hz, 1C), 135.7 (d, J = 5.4 Hz, 1C), 134.9 (s, 1C), 131.7 (d, J = 14.0 Hz, 1C), 131.1 (s, 1C), 129.0 (d, J = 14.7 Hz, 1C), 125.6 (d, J = 5.2 Hz, 1C), 125.4 (s, 1C), 124.9 (d, J = 10.4 Hz, 1C), 124.2 (d, J = 9.5 Hz, 1C), 123.6 (d, J = 13.5 Hz, 1C), 120.8 ppm (d, J = 5.9 Hz, 1C); 1H NMR (400 MHz, CDCl3) δ 8.37 (d, J = 536.8 Hz, 1H), 7.95 (dd, J = 15.9 Hz, J = 7.6 Hz, 1H), 7.8 (d, J = 8.1 Hz, 1H), 7.83-7.80 (m, 2H), 7.66 (dt, J = 7.7 Hz, J = 1.1 Hz, 1H), 7.50 (dt, J = 7.5 Hz, J = 3.2 Hz, 1H), 7.35 (dt, J = 8.4 Hz, J = 1.1 Hz, 1H), 7.24 (dt, J = 8.0 Hz, J = 0.8 Hz, 1H), 7.20 ppm (dd, J = 8.1 Hz, J = 0.9 Hz, 1H); IR (KBr) ν 3061, 2364 (w, P-H), 1578 (m, P-Caryl), 1194 (vs, P-O), 941 (vs, P=S), 758 (s, C-H bend) cm-1; HR MS (EI) calcd for [C12H9OPS]+ 232.0112, found 232.0112. Structural details for 1: Reflections collected / unique /observed (I>2σ): 12328 / 2584 / 1607 [R(int) = 0.0728]; parameters refined: 141; formula C10H9OPS, MM 208.20 g/mol; T = 200(2) K; monoclinic, P2(1)/c (no. 14, Z = 4); a = 503.9(16) pm; b = 1391.5(5) pm; c = 1503.4(5) pm; α = 90°; β =94.504°; γ = 90°; V 1051.0(6)*106 pm3; ρ (calc.) = 1.316 g/cm3; Absorption coefficient = 0.417 mm-1; F(000) = 432; Goof (F2) = 1.018; crystal size 0.8 x 0.2 x 0.2 mm3; index ranges -6<=h<=6, -17<=k<=18, -19<=l<=19; completeness to θ = 28.35: 98.1%; R1 (I>2σ) = 0.0657, wR2 = 0.1536 (all data), Largest difference peak and hole: 0.555 and -0.524*10-6 e/pm-3.
Alternative experimental procedure for the synthesis of 1:
Using Phosphorus pentasulfide:
DOPO (54 g, 0.25 mol) and dry toluene (150 mL) were fed into a three-neck round-bottom flask equipped with a mechanical stirrer, a gas inlet and a thermometer. This mixture was heated up to 55 °C under an Ar atmosphere. P4S10 (13.9 g, 0.03125 mol) was then added and the reaction mixture was stirred at 55 °C. After 2 h at 55 °C, a second portion (13.9 g) of P4S10 was added and the mixture was stirred at 40 °C over a period of 15 h under an Ar atmosphere. The cloudy solution was decanted up from the phosphorus sulfides/oxides and was filtered through a thin layer of silica gel. Afterwards the toluene was removed in vacuo. The resulting oil was dissolved in chloroform (100 mL). A small amount of a precipitate was removed by filtration. The filtrate was then stirred with an aqueous solution of sodium hydrogen carbonate (100 mL) for 20 min under argon (the chloroformic phase became milk-like; evolution of CO2). The cloudy chloroformic phase was collected, dried over sodium sulphate and filtered over silica gel. The resulting clear solution was concentrated in vacuo to yield an oily product that gradually solidified (43.5g, 0.18 mol, 70 %). mp 88 °C; 31P NMR (101 MHz, CDCl3) δ 57.1 ppm.
Using Lawesson reagent:
DOPO (0.32 g, 1.50 mmol), Lawesson reagent (1.22 g, 3.0 mmol) and dry toluene (100 mL) were charged into a three-neck round-bottom flask equipped with a mechanical stirrer, a gas inlet and a thermometer. While under Ar atmosphere, this mixture was heated up to 140 °C using an oil bath. After 1 h at 140 °C, the oil bath was removed and the reaction mixture was allowed to cool to room temperature. Once cooled the solvent was removed in vacuo to yield a brown solid. The impure product was then redissolved in chloroform and filtered over silica gel. The resulting liquid was then concentrated in vacuo to yield a pale yellow syrup. The syrup was then recrystallised from acetonitrile to yield a crystalline white solid (0.118g, 0.51 mmol, 34%). mp 88 °C; 31P NMR (101 MHz, CDCl3) δ 57.1 ppm.
2-(10-Thioxo-10H-9-oxa-10λ5-phosphaphenanthren-10-yl) benzene-1,4-diol (3): A thoroughly dried two-neck round-bottom flask fitted with a consender and an Ar gas inlet was charged with DOPS (12.3 g, 53.0 mmol) an dry toluene (20 mL). This solution was heated under positive argon pressure using an oil bath at 110 °C. While under an Ar atmosphere, benzoquinone (6.30 g, 58.0 mmol) was added in portions to the stirring solution over a period of 30 min. After 3.5 h under reflux, 1 was fully consumed and the heat source was removed to allow the reaction mixture to cool down to room temperature. The crude black reaction mixture was concentrated in vacuo to yield a black solid. The black solid was triturated with cold dichloromethane and the resulting suspension was filtered to yield a spectroscopically pure off-white solid (13.3 g, 39.2 mmol, 74%). mp 127 °C; 31P NMR(101 MHz, DMSO-d6) δ 73.6 ppm; 13C NMR (101 MHz, DMSO-d6) δ 152.5 (s, 1C), 150.0 (d, J = 18.2 Hz, 1C), 149.7 (d, J = 9.8 Hz, 1C), 134.0 (d, J = 4.6 Hz, 1C), 132.8 (s, 1C), 131.1 (s, 1C) 130.1 (d, J = 14.2 Hz, 1C), 129.2 (d, J = 106.4 Hz, 1C), 129.2 (d, J = 14.6 Hz, 1C), 126.1 (s, 1C), 125.1 (s, 1C), 124.2 (d, J = 8.8 Hz, 1C), 123.4 (s, 1C), 122.5 (d, J = 12.4 Hz, 1C), 121.0 (d, J = 5.8 Hz, 1C), 120.4 (d, J = 14.2 Hz, 1C), 118.1 (d, J = 9.2 Hz, 1C), 116.8 (d, J = 111.8 Hz, 1C); 1H NMR (400 MHz, DMSO-d6) δ 9.53 (s, 1H), 9.21 (s, 1H), 8.11 (d, J = 7.8 Hz, 2H), 7.63 (dt, J = 6.6 Hz, J = 1.3 Hz, 1H), 7.47-7.36 (m, 4H), 7.24 (dt, J = 7.2 Hz, J = 0.8 Hz, 1H), 7.18 (dd, J = 8.1 Hz, J = 0.6 Hz, 1H), 6.85 (dd, J = 8.7 Hz, J = 2.8 Hz, 1H), 6.58 ppm (t, J = 5.6 Hz, 1H); IR (KBr) ν 3267 (br, -OH), 3190, 1591 (s, P-Caryl), 1580, 1471, 1221 (vs, P-O), 1182 (vs, P-O), 901 (vs, P=S), 752 cm-1; HRMS (EI) calcd for [12C18H13PO3S]+ 340.0326, found 340.0323; Anal. Calcd for C18H13PO3S: C, 63.52; H, 3.85. Found: C, 63.22; H, 4.15.
The experimental data for compound 7 is given as a typical representative of an Atherton-Todd reaction involving DOPS (1) as a substrate.
1-(10-Thioxo-10H-9-oxa-10λ5-phosphaphenanthren-10-yl)piperidine (7): A flame dried three neck flask with a condenser, thermometer and an addition funnel was charged with 1 (3.80 g, 16.4 mmol), carbon tetrachloride (2.77 g, 18.0 mmol) and 20 mL of dry chloroform. The reaction mixture was cooled with an ice bath to 5 °C. The addition funnel was charged with triethylamine (1.80 g, 18.0 mmol) and piperidine (1.39 g, 16.4 mmol) dissolved in 20 mL of dry chloroform. The triethylamine mixture was added dropwise under vigorous stirring. The reaction temperature was not allowed to exceed 10 °C. After 1 h the addition was completed and NMR analysis indicated the complete conversion of the starting material. The reaction mixture was washed three times with 50 mL water to remove the triethylamine hydrochloride. The organic phase was isolated, dried over MgSO4 and the solvent was removed in vacuo. The spectroscopically pure product was obtained as a brown solid (4.51 g, 14.3 mmol, 87%) mp 72-74 °C; 31P NMR (101 MHz, CDCl3) δ 72.3 ppm; 13C NMR (101 MHz, CDCl3) δ 149.2 (s, 1C), 136.0 (s, 1C), 132.1 (s, 1C), 130.2 (s, 1C), 129.1 (d, J = 11.0 Hz, 1C), 128.6 (d, J = 14.7 Hz, 1C), 128.2 (d, J = 137.0 Hz, 1C), 124.9 (s, 1C), 124.2 (s, 1C), 123.7 (d, J = 10.7 Hz, 1C), 122.5 (d, J = 11.8 Hz, 1C), 120.6 (d, J = 5.7 Hz, 1C), 46.0 (2C), 26.2 (s, 1C), 26.1 (s, 1C), 24.5 ppm (s, 1C); 1H NMR (400 MHz, CDCl3) δ 7.92–7.87 (m, 2H,), 7.77 (ddd, J = 15.1 Hz, J = 7.6 Hz, J = 1.2 Hz, 1H), 7.60 (tt, J = 7.5 Hz, J = 1.3 Hz, 1H), 7.46 (ddt, J = 7.5 Hz, J = 3.1 Hz, J = 0.9 Hz, 1H), 7.35 (tt, J = 7.6 Hz, J = 1.2 Hz, 1H), 7.26–7.18 (m, 2H), 3.34–3.28 (m, 4H, N-CH2), 1.63–1.60 (m, 2H, CH2), 1.54–1.49 ppm (m, 4H, CH2); IR (KBr) ν 3052, 2955, 2934, 2918, 2848, 1604 (w, P-Caryl), 1580, 1474, 1443, 1425, 1206 (vs, P-O), 1164, 1113, 1059 (vs, P-N), 962 (vs, P=S), 783, 746, 725 cm-1; HRMS (EI) calcd for [12C17H18NPOS]+ 315.0847, found 315.0852. Anal. Calcd for C17H18NPOS. ¼ H2O: C, 63.83; H, 5.83; N, 4.38. Found: C, 64.03; H, 5.67; N, 3.96; Structural details for 7: Reflections collected / unique /observed (I>2σ): 19987 / 3768 / 2523 [R(int) = 0.0891]; parameters refined: 192; formula C17H18NOPS, MM 315.35 g/mol; T = 200(2) K; monoclinic, P2(1)/c (no. 14, Z = 4); a = 1124.0(5) pm; b = 1302.0(6) pm; c = 1174.8(5) pm; α = 90°; β =116.068(5)°; γ = 90°; V 1544.3(11)*106 pm3; ρ (calc.) = 1.356 g/cm3; Absorption coefficient = 0.311 mm-1; F(000) = 664; Goof (F2) = 1.109; crystal size 0.4 x 0.4 x 0.06 mm3; index ranges -14<=h<=14, -17<=k<=17, -15<=l<=15; completeness to θ = 28.44: 92.8%; R1 (I>2σ) = 0.1135, wR2 = 0.2931 (all data), Largest difference peak and hole: 1.561 and -0.506*10-6 e/pm-3.
10-Phenethyloxy-9-oxa-10-phosphaphenanthrene 10-sulfide (5). The product was isolated as a white solid (4.31 g, 12.2 mmol, 75%). mp 102-104 °C; 31P NMR (101 MHz, CDCl3) δ 76.9 ppm; 13C NMR (101 MHz, CDCl3) δ 149.7 (d, J = 11.3 Hz, 1C), 136.7 (s, 1C), 134.4 (d, J = 4.9 Hz, 1C), 133.1 (s, 1C), 130.6 (J = 14.7 Hz, 1C), 130.2 (s, 1C), 128.6 (s, 2C), 128.4 (s, 2C), 128.0 (s, 1C), 126.8 (d, J = 145.7 Hz, 1C), 126.4 (s, 1C), 125.1 (s, 1C), 124.6 (s, 1C), 123.6 (d, J = 10.6 Hz, 1C), 122.6 (d, J = 12,2 Hz, 1C), 119.9 (d, J = 5.8 Hz, 1C), 66.9 (d, J = 6.7 Hz, 1C, CH2), 36.3 ppm (d, J = 6.2 Hz, 1C, CH2); 1H NMR (400 MHz, CDCl3) δ 7.91 (dd, J = 16.9 Hz, J = 7.6 Hz, 1H), 7.78–7.74 (m, 2H), 7.54 (dt, J = 7.5 Hz, J = 1.2 Hz, 1H), 7.35 (dt, J = 7.5 Hz, J = 3.7 Hz, 1H), 7.25-721 (m, 1H), 7.13 (t, J = 7.8 Hz, 1H), 7.08-702 (m, 4H), 6.88-6.86 (m, 2H) 4.24–4.18 (m, 2H, CH2), 2.77-2.66 ppm (m, 2H, CH2); IR (KBr) ν 3062, 3023, 2977, 2947, 2889, 1606 (w, P-Caryl), 1580, 1559, 1496, 1474, 1447, 1429, 1194 (vs, P-O), 1115 (vs, P-O), 1045, 902 (vs, P=S), 754, 733, 716, 696 cm-1; HRMS (EI) calcd for [12C20H15PO2S]+ 352.0687, found 352.0721. Elemental analysis for C, H, N, was not within limits indicating the thermal instability of 5. Structural details for 5: Reflections collected / unique /observed (I>2σ): 21958 / 4083 / 3336 [R(int) = 0.0343]; parameters refined: 220; formula C20H17O2PS , MM per molecule 352.37 g/mol; T = 200(2) K; monoclinic, P2 (1)/n (No. 14, Z = 4); a = 969.9(3) pm; b = 1349.1(4) pm; c = 1307.3(4) pm; α = 90 °; β = 100.997(4)°; γ = 90°; V = 1679.3(8)*106 pm3; ρ (calc.) = 1.394 g/cm3; Absorption coefficient = 0.297 mm-1; F(000) = 736; Goof (F2) = 1.037; crystal size = 1.8 x 0.6 x 0.4 mm3; index ranges -12<h<12, -17<k<17, -17<l<17; completeness to θ = 28.28: 94.1%; R1 (I>2σ) = 0.0468, wR2 = 0.1099 (all data), Largest difference peak and hole: 0.379 and -0.263*10-6 e/pm-3.
Phenethyl-(10-thioxo-10H-9-oxa-10λ5-phosphaphenanthren-10-yl)amine (6). The product was isolated as a white solid (5.76g, 16.4 mmol, 82%). mp 106-109 °C; 31P NMR (101 MHz, CDCl3) δ 69.3 ppm; 13C NMR (101 MHz, CDCl3) δ 137.2 (s, 1C), 133.7 (d, J = 6.6 Hz, 1C), 131.5 (s, 1C), 129.4 (s, 1C), 128.6 (d, J = 11.6 Hz, 1C), 127.8 (s, 2C), 127.6 (s, 1C), 127.5 (s, 1C), 126.5 (d, J = 133 Hz, 1C), 125.6 (s, 1C), 124.0 (s, 1C), 123.5 (s, 1C), 122.7 (d, J = 10.6 Hz, 1C), 121.6 (d, J = 12.1 Hz, 1C), 119.8 (d, J = 5.9 Hz, 1C), 42.5 (s, 1C), 36.8 ppm (d, J = 5.3 Hz, 1C); 1H NMR (400 MHz, CDCl3) δ 7.83 (d, J = 8.1 Hz, 1H), 7.82 (d, J = 6.5 Hz, 1H), 7.73 (dd, J = 14.9, J = 7.1 Hz, 1H), 7.55 (dt, J = 8.5 Hz, J = 1.3 Hz, 1H), 7.38 (dt, J = 7.5 Hz, J = 3.3 Hz, 1H), 7.31 (dt, J = 8.2 Hz, J = 1.1 Hz, 1H), 7.20-7.11 (m, 6H), 7.00 (dd, J = 8.0 Hz, J = 0.9 Hz, 2H), 3.30 (q, J = 6.7 Hz, 1H), 3.19-3.09 (m, 2H), 2.74-2.62 ppm (m, 2H); IR (KBr) ν 3301 (br, -NH), 3062, 3028, 2932, 1582 (m, P-Caryl), 1477, 1211 (vs, P-O), 1092 (vs, P-N), 745 cm-1; HRMS (EI) calcd for [12C20H18NPOS]+ 351.0847, found 351.0891. Anal. Calcd for C20H18NPOS. ½ H2O: C, 66.65; H, 5.31; N, 3.89. Found: C, 66.98; H, 5.35; N, 3.64. Structural details for 6: Reflections collected / unique / observed (I>2σ): 42678 / 4249 / 2789 [R(int) = 0.0711]; parameters refined: 224; formula C20H18NOPS, MM 351.35 g/mol; T = 200(2) K; orthorhombic, Pbca (no. 61, Z = 8); a = 729.4(3) pm; b = 1989.0(8) pm; c = 2369.8(9) pm; α = 90°; β =90°; γ = 90°; V 3438(2)*106 pm3; ρ (calc.) = 1.358 g/cm3; Absorption coefficient = 0.287 mm-1; F(000) = 1472; Goof (F2) = 1.059; crystal size 0.4 x 0.4 x 0.2 mm3; index ranges -9<=h<=9, -26<=k<=25, -31<=l<=31; completeness to θ = 28.32: 88.1%; R1 (I>2σ) = 0.0937, wR2 = 0.1453 (all data), Largest difference peak and hole: 0.551 and -0.325*10-6 e/pm-3.
X Ray structure:
The crystal structures of molecule 1, 5, 6 and 7 were deposited at the Cambridge Crystallographic Data Centre and the corresponding data can be found at the deposition numbers: CCDC 802202 – 802205.
ACKNOWLEDGEMENTS
The authors would like to thank Mr. Zwick for measuring the HR-MS, Ms Schmelcher and Ms Kölmel for elemental analysis and Mrs. Lenzner for carrying out the differential scanning calorimetry experiments.
References
1. L. D. Quin, A Guide to Organophosphorus Chemistry; Wiley-Interscience: New-York, USA, 2000.
2. F. H. Westheimer, Science, 1987, 235, 1173. CrossRef
3. R. A. Cherkasov, G. A. Kutyrev, and A. N. Pudovik, Tetrahedron, 1985, 41, 2567. CrossRef
4. G. Keglevich, A. Kerenyi, B. Mayer, T. Kortvelyesi, and K. Ludanyi, Transit. Metal. Chem., 2008, 33, 505.
5. K. J. M. Andrews, F. R. Atherton, F. Bergel, and A. L. Morrison, J. Chem. Soc., 1954, 1638. CrossRef
6. Z. R. Li, J. Y. Han, Y. Y. Jiang, P. Browne, R. J. Knox, and L. Q. Hu, Bioorgan. Med. Chem., 2003, 11, 4171. CrossRef
7. R. F. Borch and G. W. Canute, J. Med. Chem., 1991, 34, 3044. CrossRef
8. M. Mevel, T. Montier, F. Lamarche, P. Delepine, T. Le Gall, J. J. Yaouanc, P. A. Jaffres, D. Cartier, P. Lehn, and J. C. Clement, Bioconjugate Chem., 2007, 18, 1604. CrossRef
9. L. Kollar and G. Keglevich, Chem. Rev., 2010, 110, 4257. CrossRef
10. S. V. Levchik and E. D. J. Weil, Fire Sci., 2006, 24, 345. CrossRef
11. S. V. Levchik and E. D. J. Weil, In ‘Advances in Fire Retardant Materials’, 2008, p. 41. CrossRef
12. M. Rakotomalala, S. Wagner, and M. Döring, Materials, 2010, 3, 4300. CrossRef
13. C. Krawiecki and J. Michalski, J. Chem. Soc., 1960, 881. CrossRef
14. A. Zwierzak, Can. J. Chem., 1967, 45, 2501. CrossRef
15. A. Schafer, S. Seibold, W. Lohstroh, O. Walter, and M. Doring, J. Appl. Polym. Sci., 2007, 105, 685. CrossRef
16. R. J. Reiffenstein, W. C. Hulbert, and S. H. Roth, Ann. Rev. Pharmacol., 1992, 32, 109. CrossRef
17. A. A. Abramov, Dokl. Akad. Nauk. Sssr., 1950, 74, 1051.
18. C. S. Wang, C. H. Lin, and C. Y. Chen, J. Polym. Sci. Pol. Chem., 1998, 36, 3051. CrossRef
19. C. S. Wang and J. Y. Shieh, Polymer, 1998, 39, 5819. CrossRef
20. F. R. Q. Atherton, Rev. Chem. Soc., 1949, 3, 146. CrossRef
21. F. R. Atherton, H. T. Openshaw, and A. R. Todd, J. Chem. Soc., 1945, 382. CrossRef
22. F. R. Atherton, H. T. Openshaw, and A. R. Todd, J. Chem. Soc., 1945, 660. CrossRef
23. F. R. Atherton and A. R. Todd, J. Chem. Soc., 1947, 674. CrossRef
24. F. R. Atherton, H. T. Howard, and A. R. Todd, J. Chem. Soc., 1948, 1106. CrossRef
25. R. L. Lapp and R. A. Jacobson, Cryst. Struct. Commun., 1980, 9, 65.
26. J. R. Krishna, M. Krishnaiah, G. S. Prasad, C. S. Reddy, and V. G. Puranik, Acta. Crystallogr. E, 2007, 63, O2407. CrossRef
27. R. A. Moss, P. K. Gong, and H. Morales-Rojas, Org. Lett., 2002, 4, 1835. CrossRef
28. A. E. Koziol and K. Suwinska, Acta Crystallogr. C, 1992, 48, 398. CrossRef
29. M. D. Fernandez, C. P. Vlaar, H. Fan, Y. H. Liu, F. R. Fronczek, and R. P. Hammer, J. Org. Chem., 1995, 60, 7390. CrossRef