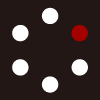
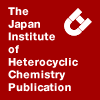
HETEROCYCLES
An International Journal for Reviews and Communications in Heterocyclic ChemistryWeb Edition ISSN: 1881-0942
Published online by The Japan Institute of Heterocyclic Chemistry
e-Journal
Full Text HTML
Received, 16th December, 2010, Accepted, 18th January, 2011, Published online, 24th January, 2011.
DOI: 10.3987/COM-10-12125
■ Silyl Nitronate 1,3-Dipolar Cycloaddition Reactions with meso-Tetraarylporphyrins
Mothi M. Ebrahim, Maxime Moreau, and Mathias O. Senge*
School of Chemistry, SFI Tetrapyrrole Laboratory, Trinity College Dublin, Dublin 2, Ireland
Abstract
Isoxazoline-fused chlorins were obtained in moderate yields from the reaction of silyl nitronates and 5,10,15,20-tetra(pentafluorophenyl)porphyrin in toluene at 85 °C.Chlorins are a class of reduced porphyrins characterized by one saturated pyrrolic Cβ–Cβ bond. Besides being at the core of natural photosynthetic system such as chlorophylls, chlorins have attracted a great deal of attention due to their distinctive optical and photochemical properties. Most notably, their strong absorption near the red end of the visible spectrum has made them one of the highly desired tetrapyrrolic macrocycles for their potential utility as second generation photosensitizers in photodynamic therapy of cancer.1 Such and other newly emerging applications have made these molecules attractive targets for chemical synthesis. Apart from total synthesis and modification of naturally occurring chlorins, a highly practical strategy has been the derivatization of simple and easily available porphyrins. In this context, amongst β-β addition reactions, 1,3-dipolar cycloaddition reactions feature prominently as an one step strategy to obtain five-membered heterocycle-fused chlorins.2 Not surprisingly, a host of dipolar molecules including azomethine ylides,3 diazoalkanes,4 nitrones,5 carbonyl ylides,6 nitrile oxides7 and nitrile imines8 have been examined for their 1,3-dipolar cycloaddition reaction with porphyrins.9
Isoxazolines are noteworthy for their biological applications and have proven particularly useful as precursors for several multi-functional intermediates such as β-hydroxyketones, α,β-unsaturated ketones, γ- amino alcohols, etc., to name a few.10 Although nitrile oxide 1,3-dipolar cycloaddition provides access to isoxazoline-fused chlorins, the method suffers from poor selectivity (both chlorins and bacteriochlorins are formed) and instability of the reagent.7 Noting the difference in reactivity we envisaged that an alternate strategy could be the use of silyl nitronates as 1,3-dipoles to make isoxazoline-fused chlorins.11 Here, we report the results of our initial investigation of the reactivity of silyl nitronates towards meso-tetraarylporphyrins.
Silyl nitronates (2) were easily obtained from primary aliphatic nitro compounds and triisopropylsilyl chloride.12 Considering the fact that silyl nitronates exhibit superior reactivity with electron-deficient olefins, we chose 5,10,15,20-tetra(pentafluoro)porphyrin as an electron-deficient porphyrin substrate for 1,3-dipolar cycloaddition reaction. Our preliminary attempts involving reactions with silyl nitronates 2d and 2e in toluene at 85 °C provided very a small amount of product (Table 1).
Different reaction conditions were tried, such as changes in solvent (toluene, PhCl, 1,2-Cl2Ph), temperature (80-110 °C) and silyl nitronate reagents. With toluene as solvent and 85 °C as optimum temperature, we found that silyl nitronates containing longer alkyl chains such as n-propyl (entry 1) and n-pentyl (entry 2) provided an appreciable increase in yield (Table 1). Best results were obtained when silyl nitronate containing an ester functionality was used (entry 3). Interestingly, in all the cases only isoxazolines and not the expected N-silyloxyisoxazolidines were obtained as the final product due to the spontaneous elimination of silanol. In addition, no bisaddition was observed. Having found compound 2c as the best dipole we then tried to use the less electron-deficient, 5,10,15,20-tetrakis(3,5-difluorophenyl)porphyrin. Performing the reaction under the identical conditions, however, yielded only traces of the desired product. In contrast, no product was detected when 5,10,15,20-tetraphenylporphyrin was employed as dipolarophile. The observed low conversion in all the reactions can be traced to the high protolytic lability and low thermal stability of the silyl nitronate reagents. It is worth noting that the TMS group, which is the typical silyl group used in this type of 1,3-dipolar cycloadditions, was found to be unsuitable in the present case due to above mentioned reasons.
The identities of compounds 3a-3c were fully established by their MS, 1H NMR and UV-vis spectra.13 The most straightforward evidence for the formation of chlorins came from the UV-vis spectra with the characteristic absorption at 650 nm. The molecular dissymmetry is clearly visible from the 1H NMR spectra. While the NH protons appear as two separate singlets, the eight β-pyrrolic protons show multiple signals in the range 8.45–8.80 ppm. The fused isoxazoline ring protons show considerable deshielding (6.03-6.63 and 7.55-7.76 ppm) due to the proximity of the porphyrin ring current as well as the two heteroatoms. The ‘cis’ configuration of these bridgehead protons are evident from their vicinal coupling constant value (~9 Hz).14
In summary, we have shown that isoxazoline fused chlorins can be obtained conveniently via silyl nitronate 1,3-dipolar cycloaddition reactions. Compared to previous studies this method allows the selective synthesis of chlorins and no bisaddition leading to bacteriochlorins was observed.
ACKNOWLEDGEMENTS
This work was supported by a grant from Science Foundation of Ireland (SFI 09/IN.1/B2650). MME thanks IRCSET for a postdoctoral fellowship.
References
1. H. Scheer, 'Chlorophylls,' CRC Press, Boca Raton, 1991; M. O. Senge, A. Wiehe, and C. Ryppa, in: Chlorophylls and Bacteriochlorophylls: Biochemistry, Biophysics, Functions and Applications, Vol. 25, ed. by B. Grimm, R. Porra, W. Rüdiger, and H. Scheer, ‘Advances in Photosynthesis and Respiration,’ Springer, Dordrecht, 2006, pp. 27-37; T. Fukuda and N. Kobayashi, Dalton Trans., 2008, 4685. CrossRef
2. A. C. Tomé, M. G. P. M. S. Neves, and J. A. S. Cavaleiro, J. Porphyrins Phthalocyanines, 2009, 13, 408. CrossRef
3. A. M. G. Silva, A. C. Tomé, M. G. P. M. S. Neves, A. M. S. Silva, and J. A. S. Cavaleiro, Chem. Commun., 1999, 1767. CrossRef
4. A. M. G. Silva, A. C. Tomé, M. G. P. M. S. Neves, and J. A. S. Cavaleiro, Synlett, 2002, 1155. CrossRef
5. A. M. G. Silva, A. C. Tomé, M. G. P. M. S. Neves, A. M. S. Silva, J. A. S. Cavaleiro, D. Perrone, and A. Dondoni, Tetrahedron Lett., 2002, 43, 603. CrossRef
6. J. Fleming and D. Dolphin, Tetrahedron Lett., 2002, 43, 7281. CrossRef
7. X. Li, J. Zhuang, Y. Li, H. Liu, S. Wang, and D. Zhu, Tetrahedron Lett., 2005, 46, 1555; CrossRef X. Liu, Y. Feng, X. Chen, F. Li, and X. Li, Synlett, 2005, 1030; CrossRef L. Xingang, F. Yaqing, H. Xiaofen, and L. Xianggao, Synthesis, 2005, 3632; CrossRef S. Ostrowski, P. Wyrębek, and A. Mikus, Heterocycles, 2006, 68, 885. CrossRef
8. N. M. M. Moura, F. Giuntini, M. A. F. Faustino, M. G. P. M. S. Neves, A. C. Tomé, A. M. S. Silva, E. M. Rakib, A. Hannioui, S. Abouricha, B. Röder, and J. A. S. Cavaleiro, ARKIVOC, 2010, (v), 24.
9. M. O. Senge and J. Richter, J. Porphyrins Phthalocyanines, 2004, 8, 934; CrossRef S. Horn, K. Dahms, and M. O. Senge, J. Porphyrins Phthalocyanines, 2008, 12, 1053. CrossRef
10. I. N. N. Namboothiri and N. Rastogi, Top. Heterocycl. Chem., 2008, 12, 1. CrossRef
11. W. Dehaen and A. Hassner, Tetrahedron Lett., 1990, 31, 743. CrossRef
12. J. E. Wilson, A. D. Casarez, and D. W. C. MacMillan, J. Am. Chem. Soc., 2009, 131, 11332; CrossRef E. W. Colvin, A. K. Beck, B. Bastani, D. Seebach, Y. Kai, and J. D. Dunitz, Helv. Chim. Acta, 1980, 63, 697. CrossRef
13. Selected analytical data. 3a – Purple solid, yield: 9.0 mg (0.008 mmol, 16%), mp >250 °C; Rf (CH2Cl2/n-hexane, 1:1 v/v, silica) 0.41; 1H NMR (400 MHz, CDCl3): δ = –1.87 (s, 1H, NH), –1.83 (s, 1H, NH), 0.71 (t, 3H, J=7.6 Hz, (CH2)2CH3), 1.12–1.15 (m, 4H, (CH2)2CH3), 6.03 (d, 1H, J=9.3 Hz, CH), 7.55 (d, 1H, J=9.3 Hz, CH), 8.45 (d, 1H, J=4.7 Hz, βH), 8.50–8.53 (m, 2H, βH), 8.56 (d, 1H, J=4.7 Hz, βH), 8.74–8.77 (m 2H, βH); UV/vis (CH2Cl2): λmax (log ε) = 404 (5.10), 505 (4.03), 532 (3.63) 598 (3.52), 650 (4.46); HRMS (MALDI-TOF+) [C48H17N5OF20]: calcd [M+] 1059.1114, found 1059.1083. 3b – Purple solid, yield: 12.4 mg (0.011 mmol, 18%), mp >250 °C; Rf (CH2Cl2/n-hexane, 1:1 v/v, silica) 0.52; 1H NMR (400 MHz, CDCl3): δ = –1.87 (s, 1H, NH), –1.83 (s, 1H, NH), 0.72 (t, 3H, J=6.4 Hz, (CH2)4CH3) 1.25–1.31 (m, 6H, CH2(CH2)3CH3), 1.60–1.62 (m, 2H, CH2(CH2)3CH3), 6.04 (d, 1H, J=8.8 Hz, CH), 7.55 (d, 1H, J=8.8 Hz, CH), 8.46 (d, 1H, J=4.1 Hz, βH), 8.50–8.54 (m, 2H, βH), 8.56 (d, 1H, J=4.7 Hz, βH), 8.75–8.78 (m 2H, βH); UV/vis (CH2Cl2): λmax (log ε) = 404 (5.10), 505 (4.04), 532 (3.64) 598 (3.53), 650 (4.45); HRMS (MALDI-TOF+) [C50H21N5OF20]: calcd [M+] 1087.1427, found 1087.1400. 3c – Purple solid, yield: 20.5 mg (0.019 mmol, 34%), mp >250 °C; Rf (CH2Cl2/n-hexane, 1:1 v/v, silica) 0.38; 1H NMR (400 MHz, CDCl3): δ = –1.99 (s, 1H, NH), –1.97 (s, 1H, NH), 3.61 (s, 3H, CH3), 6.63 (d, 1H, J=9.3 Hz, CH), 7.76 (d, 1H, J=9.3 Hz, CH), 8.48 (d, 1H, J=4.7 Hz, βH), 8.54 (s, 2H, βH), 8.57 (d, 1H, J=4.7 Hz, βH), 8.75 (d, 1H, J=4.7 Hz, βH), 8.80 (d, 1H, J=4.7 Hz, βH); UV/vis (CH2Cl2): λmax (log ε) = 405 (5.12), 504 (4.04), 531 (3.66) 595 (3.56), 648 (4.41); HRMS (ESI-) [C47H12N5O3F20]: calcd for [M-H-] 1074.0621, found 1074.0588.
14. R. M. Silverstein and F. X. Webster, ‘Spectrometric Identification of Organic Compounds,’ John Wiley and Sons, Inc., New York, 1997.