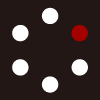
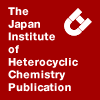
HETEROCYCLES
An International Journal for Reviews and Communications in Heterocyclic ChemistryWeb Edition ISSN: 1881-0942
Published online by The Japan Institute of Heterocyclic Chemistry
e-Journal
Full Text HTML
Received, 21st December, 2010, Accepted, 1st February, 2011, Published online, 9th February, 2011.
DOI: 10.3987/COM-10-12128
■ Synthesis of Novel Substituted 4-Hydroxy-3-oxo-3,4-dihydro-2H-1,4-benzoxazine-6,7-dicarbonitriles
Sergey I. Filimonov,* Zhanna V. Chirkova, Igor G. Abramov, Sergey I. Firgang, Galina A. Stashina, and Kyrill Yu. Suponitsky
Yaroslavl State Technical University, Moscowsky Prospekt 88, 150023 Yaroslavl, Russia
Abstract
New method has been elaborated for synthesis of novel substituted 4-hydroxy-3-oxo-3,4-dihydro-2H-1,4-benzoxazine-6,7-dicarbonitriles by means of activated nucleophilic substitution of the bromine atom in 4-bromo-5-nitrophthalonitrile by treatment of the latter with sodium salts of substituted 3-cyano-2-hydroxy-3-phenyl acrylates followed by reductive cyclisation. The structure of the resulting substituted 4-hydroxy-3-oxo-3,4-dihydro-2H-1,4-benzoxazine-6,7-dicarbonitriles has been determined by single crystal X-ray diffraction analysis.INTRODUCTION
4-Hydroxy-1,4-benzoxazin-3-ones are among the most interesting representatives of six-membered heterocyclic systems. This structural moiety is found in glycosides of key gramineous plants;1 furthermore, it determines the antibacterial, antifungal2 and herbicide3,4 properties of these compounds. Derivatives of 4-hydroxy-1,4-benzoxazinone are being intensely studied; over 500 studies concerning various aspects of chemistry and biology of this class of compounds have already been published over the past 50 years. One of the main methods for synthesizing the 1,4-benzoxazin-3-one ring involves alkylation of nitrophenols with α-haloacid derivatives followed by reductive cyclization.5,6 Other method involves acylation of corresponding chlorine anhydride with ortho-aminophenols followed by reductive alkylating cyclization.5,6 One more widely used method involves condensation of ortho-aminophenols with derivatives of α-ketoacid. Dimethyl acetylenedicarboxylate7 uses here as condensing reagent with formation of corresponding 1,4-benzoxazine-2-one.8-10 Nucleophylic substitution is described only getting of phenoxazines derivatives.11 There are some alternative ways for synthesis of heterocyclic phthalonitriles, but usually they based on high-temperature substitution of bromine (iodine) on cyanogroup (Rosenmund von Braun Synthesis) with cyanide copper (I)) with low yield,12-14 or more effective method with use of cyanide zinc and various palladium derivatives.15,16
However, the literature lacks information on the synthesis of substituted 4-hydroxy-3-oxo-3,4-dihydro-2H-1,4-benzoxazine-6,7-dicarbonitriles by activated aromatic nucleophilic substitution. It should also be noted that substituted ortho-dicarbonitriles are promising compounds for synthesising various macrocycles12,17,18 and a number of other compounds incorporating carbonyl, anhydride, imide, isoindoline and tetrazole moieties.19
RESULTS AND DISCUSSION
This study aims at development of a new two-stage synthesis of substituted 4-hydroxy-3-oxo-3,4-dihydro-2H-1,4-benzoxazine-6,7-dicarbonitriles based on reductive cyclisation of addition products of sodium salts of substituted 3-cyano-2-hydroxy-3-phenyl acrylates 2(a-d) to 4-bromo-5-nitrophthalonitrile 1 (BNPN). The characteristic features of BNPN that behaves as a highly activated substrate in SNAr reactions were considered in a number of papers.20-22 It is the most convenient substrate for synthesis of various heterocyclic systems fused with phthalonitrile. It is necessary to notice that there are almost no information about using of substituted 3-cyano-2-hydroxy-3-phenylacrylate as nucleophilic reagents,23 however it is known, that they are condensed with carbonylderivatives with formation of corresponding 4-Oxo-3,4-dihydro-2H-[1,3]oxazine-6-carboxylic acid ethyl ester.24,25
The first stage, i.e. reaction of BNPN with an excess of salts 2(a-d), was carried out for 24-48 h in a DMF solution at room temperature (Scheme 1). Increasing the reaction temperature favoured side reactions and thus decreased the yield of the target product.
It was found that an increase in the amount of salt 2(a-d) in the reaction mixture increased the yield of ester 3(a-d), which reached a maximum with a two-fold excess of the reagent. Conversely, addition of potassium carbonate or sodium methoxide to the reaction mixture decreased the yield. Notwithstanding the ambident nature of nucleophile 2(a-d), O-nucleophilic substitution was the dominating process under the conditions used, to give esters 3(a-d) as the main reaction products (white crystals; yield 38-64%). Furthermore, esters with electron-donating substituents (Me, OMe) at the phenyl ring are formed more quickly. Salts containing substituents R = H or Cl react much more slowly, especially for R = Cl (complete conversion of BNPN took about 150 hours). Synthesized substituted enol ethers 3 are unstable in solutions, containing bases, because they can be exposed cleavage (similar mechanism is described for 1,3-diketones)26,27 with formation of 4-hydroxy-5-nitrophthalonitrile, therefore target product with low-activity substrate have received with a low yield, and nitrophenol is isolated as sodium salts with 15-20% yields.
At the second stage, esters 3(a-d) were reduced with tin(II) in hydrochloric acid at 40-50 °C to give bright yellow high-melting target products 4(a-d), poorly soluble in the majority of organic solvents, in 60-75% yields (Scheme 2). It is known that reduction of the nitro group in nitroesters with similar structures in the presence of tin (II),28 zinc,29 indium30 or palladium on carbon31,32 under mild conditions initially gave a hydroxylamine, which then underwent spontaneous cyclisation to give the corresponding N-hydroxy compounds. In some cases, formation of crystal hydrates was detected.31
It appeared rather difficult to determine unambiguously the structure of the resulting compounds 3(a-d) and 4(a-d) by NMR spectroscopy, but all compounds is represented by homogeneous products. Electron impact did not always allow the molecular ion to be detected for these compounds, but only low-molecular fragments could be observed. The assumption that compound 4с*1 belongs to derivatives of N-hydroxy substituted oxazines was made upon hydroxyl group methylation with methyl iodide. 1H NMR revealed an additional methoxy group signal at δ 4.06 in reaction product 5с at N1.
An information on the structure of the compounds synthesized in the present study 4(а-d) was obtained by the single crystal X-ray study of 4c.* The single crystals were obtained from THF solution in the form of yellow prisms. An independent unit cell consists of 4c and water molecule in the 1:1 ratio. General view of 4с•H2O is depicted in Figure 1. The 4c molecule adopts planar geometry and orientation of substituents at С1=С11 double bond corresponds to E-isomer. This probably is defined by an orientation of substitents in the initial ester 3c. The C14-H14A…O1 intramolecular H-bond is observed in the structure of 4c. However the planar molecular geometry is probably governed by π-conjugation between π-donor and π-acceptor substituents rather than weak C-H…O interaction which should be considered as a forced interaction.
The presence of π-conjugation is supported by elongation of С1=С11 and shortening of С11-С13 bonds (normally observed X-ray values are 1.339 и 1.488Å,33 respectively) as well as bond lengths distribution in С13...С18 and С3...С10 phenyl cycles which adopt to a some extent quinoid geometry. The С14-С15 and C7-С18 bonds in aryl substituent at methoxy-group (С13...С18) are somewhat shorter than others while simultaneous influence of two cyano-groups in phtalonitrile cycle (C3…C10) and π-donor properties of O1 and N1 atoms of oxazine cycle lead to shortening of С7-С9, С9-С10, С3-С4, С4-С5 bonds (Table 1).
Intensive fluorescent at λmax = 560 – 580 nm was observed for synthesized benzoxazines 4(a-d) upon UV irradiation (λ=265 nm) which makes them to be promising compounds for an application as elements of new fiber-optic, fluorescent and other modern optical materials.34
EXPERIMENTAL
IR spectra were measured on a Perkin-Elmer RX-1 spectrometer in the range of 700–4000 cm-1
NMR spectra were recorded on a Bruker DRX-500 instrument at 30 °C, residual solvent proton signals in 1H NMR spectra (δδH 2.50) or the signal of DMSO-d6 in 13C spectra (δδC 39.5) were used as references for chemical shift measurements.
The starting BNPN 1 was synthesised using the procedure reported elsewhere.35
The starting sodium salts 2(a-d) were synthesised using the procedure reported elsewhere.36
General procedure for the synthesis of compounds 2(a-d) – Etyl (2E)-3-cyano-2-hydroxy-3-(aryl)acrylates sodium salts.
Hexane (300 mL) and sodium hydride (0.11 mol) are added to a solution of benzyl cyanide (0.1 mol) and diethyl oxalate (0.11 mol) in 50 mL of anhydrous dioxane and the suspension is stirred without heating until hydrogen evolution ceases completely and a yellow precipitate is formed (2-4 h); the latter is filtered off, washed with dry hexane and used in reactions without further purification.
General procedure for the synthesis of compounds 3(a-d)
Compound 2(3) (0.0044 mol) is added to a solution of BNPN (0.002 mol) in 3 mL of DMF; the mixture is stirred for 24-48 h at room temperature and poured into 10 mL of cold 1% hydrochloric acid solution. The resulting resinous precipitate is extracted with CH2Cl2, thoroughly washed with water, and chromatographed on silica gel using hexane:CH2Cl2 (1:2) as the eluent. The eluent is evaporated, and the resulting precipitate is filtered off and recrystallised from EtOH.
General procedure for the synthesis of compounds 4(a-d)
A solution of compound 2 in EtOH (3 mL) is added to a solution of tin dichloride (0.007 mol) in concentrated hydrochloric acid (2 mL). The reaction mixture is stirred for 1 h at 50 °С; the resulting yellow precipitate is filtered off and recrystallized from a DMF: EtOH (1:2) mixture.
General procedure for the synthesis of compounds 5
Compound 4 (0.001 mol) is dissolved in DMF (3 mL); potassium carbonate (0.0012 mol) and methyl iodide (0.0012 mol) are added in succession. The reaction mixture is stirred at room temperature for 24 h and then diluted with water (3 mL); the resulting precipitate is filtered off and recrystallised from a DMF: EtOH mixture (1:2).
Ethyl (2E)-3-cyano-2-(4,5-dicyano-2-nitrophenoxy)-3-phenylacrylate (3a)
yield 44%, mp 143-145 °C (EtOH); IR (KBr) v 2240 (C≡N), 1742 (C=O), 1539 (NO2), 1261 (C-O-C), 1141(C-O-C) cm-1; 1H NMR (DMSO-d6): δ 1.15 (t, J=7.1 Hz, 3H, CH3), 4.25 (q, J=7.1 Hz, 2H, CH2), 7.51 (m, 3H, aryl), 7.64 (m, 2H, aryl), 8.60 (s, 1H, 6-H), 8.93 (s, 1H, 3-H). 13C NMR (DMSO-d6): δ 13.50, 63.37, 111.07, 114.18, 114.29, 114.87, 115.61, 120.60, 124.11, 128.87, 129.17, 129.24, 131.18, 131.37, 141.12, 147.32, 150.81, 158.41; Anal. Calcd for C19H18N8O5: C, 61.86; H, 3.11; N, 14.43. Found: C, 61.95; H, 2.94; N, 14.47.
Ethyl (2E)-3-cyano-2-(4,5-dicyano-2-nitrophenoxy)-3-(4-methylphenyl)acrylate (3b)
yield 64%, mp 154-156 °C (EtOH); IR (KBr) v 2240 (C≡N), 1714 (C=O), 1538 (NO2), 1297 (C-O-C), 1157 (C-O-C) cm-1; 1H NMR (DMSO-d6): δ 1.22 (t, J=7.0 Hz, 3H, CH3), 2.36 (s, 3H, CH3’), 4.28 (q, J=7.0 Hz, 2H, CH2), 7.28 (d, J=8.1 Hz, 2H, aryl), 7.55 (d, J=8.1 Hz, 2H, aryl), 8.55 (s, 1H, 6-H), 8.87 (s, 1H, 3-H); Anal. Calcd for C21H14N4O5: C, 62.69; H, 3.51; N, 13.92. Found: C, 62.82; H, 3.32; N, 13.98.
Ethyl (2E)-3-cyano-2-(4,5-dicyano-2-nitrophenoxy)-3-(4-methoxyphenyl)acrylate (3c)
yield 58%, mp 152-154 °C (EtOH); IR (KBr) v 2238 (C≡N), 1717 (C=O), 1542 (NO2), 1295 (C-O-C), 1159 (C-O-C) cm-1; 1H NMR (DMSO-d6): δ 1.19 (t, J=7.3 Hz, 3H, CH3), 3.80 (s, 3H, OCH3) 4.25 (q, J=7.3 Hz, 2H, CH2), 7.02 (d, J=8.8 Hz, 2H, aryl), 7.65 (d, J=8.8 Hz, 2H, aryl), 8.53 (s, 1H, 6-H), 8.90 (s, 1H, 3-H); 13C NMR (DMSO-d6): δ 13.51, 55.57, 63.15, 110.93, 114.18, 114.32, 114.70, 114.88, 115.64, 120.60, 121.23, 123.95, 130.92, 131.39, 141.11, 145.52, 150.92, 158.65, 161.44; Anal. Calcd for C21H14N4O6: C, 60.29; H, 3.37; N, 13.39. Found: C, 60.44; H, 3.24; N, 13.42.
Ethyl (2E)-3-(4-chlorophenyl)-3-cyano-2-(4,5-dicyano-2-nitrophenoxy)acrylate (3d)
yield 38%, mp 161-162 °C (EtOH); IR (KBr) v 2239 (C≡N), 1737 (C=O), 1540 (NO2), 1292 (C-O-C), 1152 (C-O-C) cm-1; 1H NMR (DMSO-d6): δ 1.22 (t, J=7.0 Hz, 3H, CH3), 4.28 (q, J=7.0 Hz, 2H, CH2), 7.51 (d, J=8.5 Hz, 2H, aryl), 7.67 (d, J=8.5 Hz, 2H, aryl), 8.56 (s, 1H, 6-H), 8.88 (s, 1H, 3-H); Anal. Calcd for C20H11ClN4O5: C, 56.82; H, 2.62; N, 13.25. Found: C, 56.42; H, 2.32; N, 13.08.
(2E)-2-[Cyano(phenyl)methylene]-4-hydroxy-3-oxo-3,4-dihydro-2H-1,4-benzoxazine-6,7-dicarbonitrile (4a)
yield 64%, mp 228-229 °C; IR (KBr) v 3350 (OH), 2235 (C≡N), 2213 (C≡N), 1686 (C=O), 1610 (Ar), 1260 (C-O-C) cm-1; 1H NMR (DMSO-d6): δ 7.50(t, J= 7.2 Hz,1H, aryl), 7.55(t, J= 7.2 Hz, 2H, aryl), 7.76 (d, J= 7.2 Hz, 2H, aryl), 8.01 (s, 1H, 5-H), 8.11 (s, 1H, 8-H), 12.28 (br.s, 1H, OH); 13C NMR (DMSO-d6): δ 97.39, 109.49, 110.65, 115.18, 115.29, 116.98, 117.37, 120.89, 128.81, 129.53, 130.44, 132.35, 142.17, 150.47, 150.58; Anal. Calcd for C18H8N4O3*H2O: C, 62.43; H, 2.91; N, 16.18. Found: C, 62.58; H, 2.98; N, 16.10.
(2E)-2-[Cyano(4-methylphenyl)methylene]-4-hydroxy-3-oxo-3,4-dihydro-2H-1,4-benzoxazine-6,7-dicarbonitrile (4b)
yield 75%, mp 279-280 °C; IR (KBr) v 3462 (OH), 2242 (C≡N), 2220 (C≡N), 1680 (C=O), 1613 (Ar), 1260 (C-O-C) cm-1; 1H NMR (DMSO-d6): δ 2.42 (s, 3H, CH3), 7.32 (d, J=8.5 Hz, 2H, aryl), 7.67 (d, J=8.5 Hz, 2H, aryl), 7.88 (s, 1H, 5-H), 7.95 (s, 1H, 8-H), 12.18 (br.s, 1H, O-H); 13C NMR (DMSO-d6): δ 20.98, 97.82, 109.62, 110.76, 115.32, 115.43, 117.06, 117.46, 121.01, 127.71, 128.87, 129.50, 132.51, 139.55, 142.35, 150.13, 150.65; Anal. Calcd for C19H10N4O3*H2O: C, 63.33; H, 3.36; N, 15.55. Found: C, 63.08; H, 3.46; N, 15.32.
(2E)-2-[Cyano(4-methoxyphenyl)methylene]-4-hydroxy-3-oxo-3,4-dihydro-2H-1,4-benzoxazine-6,7-dicarbonitrile (4c)
yield 68%, mp 290-291 °C; IR (KBr) v 3424 (OH), 2235 (C≡N), 2217 (C≡N), 1687 (C=O), 1611 (Ar), 1259 (C-O-C) cm-1; 1H NMR (DMSO-d6): δ 3.84 (s, 3H, O CH3), 7.09 (d, J=8.5 Hz, 2H, aryl), 7.75 (d, J=8.5 Hz, 2H, aryl), 7.97 (s, 1H, 5-H), 8.11 (s, 1H, 8-H), 12.17 (br.s, 1H, OH); 13C NMR (DMSO-d6): δ 55.29, 92.26, 107.23, 110.48, 114.04, 114.14, 115.89, 118.27, 119.24, 119.71, 123.83, 130.18, 135.15, 143.13, 150.22, 151.19, 159.29; Anal. Calcd for C19H10N4O4*H2O: C, 60.64; H, 3.21; N, 14.89. Found: C, 60.32; H, 3.32; N, 14.78.
(2E)-2-[Cyano(4-chlorophenyl)methylene]-4-hydroxy-3-oxo-3,4-dihydro-2H-1,4-benzoxazine-6,7-dicarbonitrile (4d)
yield 61%, mp 288-289 °C; IR (KBr) v 3456 (OH), 2236 (C≡N), 2217 (C≡N), 1692 (C=O), 1612 (Ar), 1256 (C-O-C) cm-1; 1H NMR (DMSO-d6): δ 7.54 (d, J=8.5 Hz, 2H, aryl), 7.78 (d, J=8.5 Hz, 2H, aryl), 8.03 (s, 1H, 5-H), 8.14 (s, 1H, 8-H), 12.27 (br.s, 1H, O-H); Anal. Calcd for C18H7ClN4O3*H2O: C, 56.78; H, 2.38; N, 14.71. Found: C, 56.32; H, 2.12; N, 14.46.
(2E)-2-[Cyano(4-methoxyphenyl)methylene]-4-methoxy-3-oxo-3,4-dihydro-2H-1,4-benzo-xazine-6,7-dicarbonitrile (5с)
yield 78%, mp 269-270 °C; IR (KBr) v 2235 (C≡N), 2217 (C≡N), 1699 (C=O), 1611 (Ar), 1259 (C-O-C), 1190 (C-O-C) cm-1; 1H NMR (DMSO-d6): δ 3.87 (s, 3H, OCH3), 4.06 (s, 3H, OCH3), 7.07 (d, J=8.8 Hz, 2H, aryl), 7.76 (d, J=8.8 Hz, 2H, aryl), 8.09 (s, 1H, 5-H), 8.14 (s, 1H, 8-H); Anal. Calcd for C20H12N4O4: C, 64.52; H, 3.25; N, 15.05. Found: C, 64.32; H, 3.15; N, 14.96.
1 *1 Reflection for compound 4c were collected at SMART 1000 difractometer (λ(Mo-Kα)=0.71073 Å, graphite monochromator, ω-scans) at 120K. The structure solved by the direct methods and refined by the full-matrix least-squares procedure against F2 in anisotropic approximation. Hydrogen atoms of OH group and water molecule were found in the difference Fourier synthesis and refined in isotropic approximation. All the other hydrogen atoms were placed in geometrically calculated positions and refined within riding model.
4c: C19H12N4O5: monoclinic, space group P21/c: a = 11.786(3)Å, b = 17.643(4)Å, c = 8.329(2)Å, β = 105.348(5), V = 1670.2(6)Å3, Z=4, M=376.33, dcalc= 1.497 g⋅cm-3, µ=0.112 mm-1, F(000)=776, R2=0.1403, GOF=1.017 for 4430 independent reflections with 2θ<58°, R1= 0.0570 for 2964 reflections with I>2σ(I)
References
1. T. Ishizaki, Y. Hashimoto, K. Shudo, and T. Okamoto, Tetrahedron Lett., 1982, 23, 4055. CrossRef
2. S. Oeztuerk, A. M. Ozturk, G. Hakan, and A. Nurten, Farmaco, 2000, 55, 715. CrossRef
3. H. M. Niemeryer, J. Agric. Food Chem., 2009, 57, 1677. CrossRef
4. F. A. Mac’as, N. Chinchilla, R. M. Varela, J. M. G. Molinillo, D. Marn, and J. M. De Siqueira, J. Agric. Food Chem., 2008, 56, 9941. CrossRef
5. J. Ilas, S. Petra, T. Anderluh, M. Sollner, and D. Kikelj, Tetrahedron, 2005, 61, 7325. CrossRef
6. J. Teller, ‘Houben-Weyl Methods of Organic Chemistry, Hetarenes IV: 1,4-(Benz)Oxazines’, ed by E. Schaumann, Georg Thieme: Stuttgart, 1997, E 9a, pp. 134-177.
7. X. Li, N. Liu, H. Zhang, S. E. Knudson, R. A. Slayden, and P. J. Tonge, Bioorg. Med. Chem. Lett., 2010, 20, 6306. CrossRef
8. D. G. Orphanos and A. Taurins, Can. J. Chem., 1966, 44, 1795. CrossRef
9. Z. G. Aliev, O. P. Krasnykh, A. N. Maslivets, and L. O. Atovmyan, Russ. Chem. Bull., 2000, 49, 2045. CrossRef
10. V. L. Gein, N. A. Rassudikhina, and E. V. Voronina, Pharm. Chem. J., 2006, 40, 554. CrossRef
11. St. Radl, ‘Advances in Heterocyclic Chemistry: Aromatic nucleophilic denitrocyclization reaction’, Vol. 83, ed. by A. R. Katritzky, Academic Press, Inc., London, 2002, pp. 189-257.
12. S. A. Mikhalenko, V. M. Derkacheva, and E. A. Luk’yanets, Russ. J. Gen. Chem., 1981, 51, 1405.
13. C. Pardo, M. Yuste, J. Elguero, and J. Porphyrins, Phthalocyanines, 2000, 4, 505. CrossRef
14. W. J. Youngblood, J. Org. Chem., 2006, 71, 3345. CrossRef
15. G. M. Barrett, G. R. Hanson, J. P. White, D. J. Williams, and A. S. Micallef, Tetrahedron, 2007, 63, 5244. CrossRef
16. Z. Iqbal, A. Lyubimtsev, and M. Hanack, Synlett, 2008, 15, 2287. CrossRef
17. V. E. Maizlish, A. E. Balakirev, O.V. Shishkina, and G. P. Shaposhnikov, Russ. J. Gen. Chem., 2001, 71, 246. CrossRef
18. O. V. Shishkina, V. E. Maizlish, and G. P. Shaposhnikov, Russ. J. Gen. Chem., 1998, 68, 813.
19. R. J. Herr, Bioorg. Med. Chem., 2002, 10, 3379. CrossRef
20. I. G. Abramov, A. V. Smirnov, S. A. Ivanovskii, M. B. Abramova, V. V. Plachtinsky, and M. S. Belysheva, Mendeleev Commun., 2001, 11, 80. CrossRef
21. I. G. Abramov, A. V. Smirnov, S. A. Ivanovskii, M. B. Abramova, and V. V. Plachtinsky, Heterocycles, 2001, 55, 1161. CrossRef
22. S. I. Filimonov, Zh. V. Chirkova, I. G. Abramov, A. S. Shashkov, S. I. Firgang, and G. A. Stashina, Mendeleev Com., 2009, 19, 332. CrossRef
23. R. Gompper and H. H. Vogt, Chemische Berichte, 1981, 114, 2866. CrossRef
24. J. F. Stambach, L. Jung, and R. Hug, Heterocycles, 1994, 38, 297. CrossRef
25. D. K. Pyun, W. J. Jeong, H. J. Jung, J. H. Kim, J. S. Lee, C. H. Lee, and B. J. Kim, Synlett, 2001, 1950. CrossRef
26. R. G. Pearson and E. A. Mayerle, J. Am. Chem. Soc., 1951, 73, 926. CrossRef
27. D. J. Bray, K. A. Jolliffe, L. F. Lindoy, and J. C. McMurtrie, Tetrahedron, 2007, 63, 1953. CrossRef
28. H. P. Buchstaller and U. Anlauf, Synthesis, 2005, 639. CrossRef
29. D. Sicker, B. Pratorius, G. Mann, and L. Meyer, Synthesis, 1989, 211. CrossRef
30. B. K. Banik, I. Banik, S. Samajdar, and M.Wilson, Heterocycles, 2004, 63, 283. CrossRef
31. M. Belley, E. Sauer, D. Beaudoin, P. Duspara, L. A. Trimble, and P. Dubé, Tetrahedron Lett., 2006, 47, 159. CrossRef
32. J. Atkinson, P. Morand, and T. Arnason, J. Org. Chem., 1991, 56, 1788. CrossRef
33. F. H. Allen, O. Kennard, D. G. Watson, L. Brammer, A. G. Orpen, and R. J. Taylor, Chem. Soc., Perkin Trans. 2, 1987, 1.
34. O. S. Wolfbeis, ‘Springer Series on Fluorescence. Springer’-Verlag Berlin Heidelberg, 2008.
35. S. A. Ivanovskii, M. V. Dorogov, I. G. Abramov, and A. V. Smirnov, Russian Patent, 2167855, 2001.
36. C. Maurin, F. Bailly, and Ph. Cotelle, Tetrahedron, 2004, 60, 6479. CrossRef