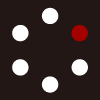
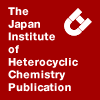
HETEROCYCLES
An International Journal for Reviews and Communications in Heterocyclic ChemistryWeb Edition ISSN: 1881-0942
Published online by The Japan Institute of Heterocyclic Chemistry
e-Journal
Full Text HTML
Received, 28th December, 2010, Accepted, 21st February, 2011, Published online, 1st March, 2011.
DOI: 10.3987/COM-10-12130
■ Synthesis of New Imidazole 3-Oxides; Unexpected Deoxygenation of Some Derivatives in the Reaction with 2,2,4,4-Tetramethylcyclobutane-1,3-dithione
Grzegorz Mlostoń,* Marcin Jasinski, Dorota Rygielska, and Heinz Heimgartner*
Department of Organic and Applied Chemistry, University of Łódź, Maritpwocza 68, PL-90-136 Łódź, Poland
Abstract
Whereas the reaction of a series of 1,4,5-trisubstituted imidazole 3-oxides with 2,2,4,4-tetramethylcyclobutane-1,3-dithione gave the corresponding imidazole-2-thiones by a sulfur-transfer reaction via [2+3] cycloaddition, an unexpected deoxygenation occurred in the case of 4-acetyl-1-(adamantan-1-yl)-5-methylimidazole 3-oxide. It was shown that the presence of an electron- withdrawing substituent at C(4) and the bulky 1-adamantyl group at N(1) are necessary to enable this reaction course. A reaction mechanism via a zwitterion, followed by a 1,3-cyclization and elimination of an oxathiirane, is proposed.INTRODUCTION
Differently functionalized imidazole derivatives are useful building blocks for the preparation of practically important compounds such as pharmaceuticals, agrochemicals, anti-corrosive agents, etc.2 Synthetic methods for the functionalization of the imidazole ring in the presence of a metal catalyst were summarized in a recent review.3 The condensation of α-hydroxyimino ketones of type (1) with primary amines and formaldehyde is widely applied for the synthesis of stable 2-unsubstituted imidazole 3-oxides (2) (Scheme 1).4 These compounds are related in their structure with aldonitrones and display reactivities analogous to this type of 1,3-dipoles. For example, they react easily with isocyanates, isothiocyanates,5 electron-deficient fluorinated olefins,6 and acetylene dicarboxylates4a,5 forming the corresponding products (3–5) via initial [2+3] cycloaddition. The same mechanism is attributed to the formation of imidazole-2-thiones (6) via the so-called sulfur-transfer reaction with 2,2,4,4-tetramethylcyclobutane-1,3-dithione (7a).7 Similar to other heterocyclic N-oxides, compounds (2) can be deoxygenated, e.g. by treatment with Raney-Ni.8
The goal of the present report was the synthesis of new imidazole 3-oxides bearing the electron-withdrawing acetyl group at C(4) (2, R2 = MeCO) and the study of the reactivity of these derivatives. In addition, some new imidazole 3-oxides bearing sterically crowded 1-adamantanyl residue at N(1) should be obtained as potentially useful substrates for preparation of stable, nucleophilic carbenes.9
RESULTS AND DISCUSSION
The preparation of 4-acetylimidazole 3-oxides 2a–d was carried out according to well established protocols. In the case of 2a–c, the condensation of 3-(hydroxyimino)pentane-2,4-dione (8a) with the corresponding 1,3,5-trisubstituted hexahydro-1,3,5-triazines (9a–c) occurred smoothly in ether at room temperature (Scheme 2). The crystalline products precipitated directly from the reaction mixture. The reaction of 8a with the monomeric N-methylidene adamantan-1-amine (10) was performed in acetic acid at room temperature.10
In the first series of reactions, imidazole 3-oxides (2) were treated with dithione (7a) in order to obtain the corresponding imidazole-2-thiones of type (6). The reactions with 2a–c occurred smoothly and led to the desired thiones (6a–c) in good yields (Scheme 3). Unexpectedly, the analogous reaction with 2d afforded a product, which in the 1H-NMR spectrum showed a singlet at 7.63 ppm, diagnostic for H–C(2) of an imidazole derivative. After crystallization, a colorless product was isolated and identified by means of spectroscopic methods as 4-acetyl-1-(adamantan-1-yl)-5-methylimidazole (11d).
The comparison of this result with those observed with 2a–c led us to the conclusion that the presence of the bulky adamantyl residue is of key importance for the change of the reaction course. In order to check the influence of substituents at C(4) and C(5) of the imidazole, two other model compounds 2e (R1 = Ad, R2 = R3 = Me) and 2f (R1 = Ad, R2 = R3 = Ph) were prepared11 and used in the reaction with 7a. In both cases, the corresponding imidazole-2-thiones (6e,f) were formed as major products. However, imidazoles (11e,f) were also found (by 1H NMR) in the reaction mixtures as minor products (<10%).
A plausible explanation for the observed results is presented in Scheme 3. It seems likely that the presence of the bulky adamantyl substituent at N(1) causes a stepwise mechanism leading to the zwitterionic intermediate A. Due to the steric hindrance, the 1,5-cyclization (route a)), leading finally to the sulfur transfer product (6) is disfavored and the competitive 1,3-cyclization (route b)) leads to the oxathiirane derivative (12a) and the deoxygenated imidazole (11d). Electronic factors are also important: the presence of an electron-withdrawing group at C(4) of the imidazole ring enhances the electrophilicity of the oxygen atom in the intermediate zwitterion A, and therefore route b) may become more advantageous in comparison with the alternative route a).
Oxathiiranes were postulated as unstable intermediates in some papers dealing with in situ generation of thiocarbonyl S-sulfides in trapping experiments with aromatic thioketones.12 In search of additional proof for the oxathiirane formation, a three-component reaction with 2d, 2,2,4,4-tetramethyl-3- thioxocyclobutan-1-one (7b), and thiobenzophenone (13) was carried out. As expected, imidazole (11d) was formed, but chromatographic workup also yielded a less polar fraction, in which the non-symmetrical 1,2,4-trithiolane (16) was identified as the major component and subsequently isolated by means of PLC (Scheme 4).12 On the other hand, none of the two possible 1,2,4-trithiolanes with symmetrical substitution pattern13,14 was found in the crude reaction mixtures based on the 1H-NMR analysis.
In this transformation, the monothione (7b) is believed to act as a deoxygenating agent (see Scheme 3, route b)), and the proposed oxathiirane (12b) transfers (S1) to either thiobenzophenone (13) or 7b.12a Thiocarbonyl S-sulfides (thiosulfines) (14) and (15), respectively, appear as in situ generated 1,3-dipoles,13 which are trapped by thioketones (7b) and (13), respectively, as C=S dipolarophiles. In both cases, the same 1,2,4-trithiolane (16)13 is formed as a stable product. Selective formation of the ‘non-symmetric’ 1,2,4-trithiolane (16) can be rationalized as follows: as evidenced in a series of papers by Huisgen,15 aromatic thioketones are ‘superdipolarophilic’ and for that reason it seems very likely that 13 can be trapped by both thiosulfines (14) and (15) faster than the cycloaliphatic, sterically hindered thioketone (7b). In addition, the ‘symmetrical’ 3,3,5,5-tetraphenyl-1,2,4-trithiolane undergoes easily the thermal [2+3] cycloreversion.14 In contrast, ‘mixed’ 1,2,4-trithiolanes, such as 16, are thermally more stable and do not undergo the [2+3] cycloreversion under the applied reaction conditions.13
Based on the presented observations, two other model N-oxides (2g) and (2h) with an adamantan-1-yl group as a bulky substituent at N(1) and an electron-withdrawing group at C(4) (ester or amide function) were prepared analogously to 2d and they were also tested in the reaction with dithioketone (7a). In both cases, the reactions occurred smoothly and the obtained products were identified as the corresponding deoxygenated imidazole derivatives (11g) and (11h), respectively (Scheme 5). An additional proof of the structure 11g was obtained by deoxygenation of 2g with Raney-Ni.
CONCLUSIONS
The results presented showed that imidazole 3-oxides (2) bearing an electron-withdrawing substituent at C(4) and the bulky 1-adamantyl residue at N(1) react with cycloaliphatic thiones (7a,b) via a stepwise mechanism and, instead of imidazole-2-thiones (6), deoxygenated imidazoles (11) are formed. In fact, [2+3] cycloaddition reactions of typical nitrones are common, and in the case of cycloaliphatic thioketones, e.g. adamantanethione or 7b as dipolarophiles, stable 1,3,5-oxathiazolidines (and not the regioisomeric 1,2,5-oxathiazolidines!) were isolated in high yields.16 Reactions of nitrones with aromatic thioketones, e.g. 13, occur with the same regioselectivity, i.e. with carbophilic attack of the oxygen atom onto the C=S bond.15c,17 The switch from the concerted to the non-concerted [2+3] cycloaddition requires steric hindrance in the 1,3-dipole or the dipolarophile.18a In addition, electronic effects, leading to stabilization of the resulting zwitterions, play also an important role.18 Apparently, both factors influence the course of the [2+3] cycloaddition of the ‘nitrone-like’ imidazole N-oxides 2d,g,h, and finally lead to a stepwise course of their reactions with cycloaliphatic thioketones 7a and 7b. The three-component reaction with 2d, 7b and thiobenzophenone (13) evidenced the intermediacy of an oxathiirane derivative19 acting as a (S1)-transferring reagent.
EXPERIMENTAL
General remarks. Melting points were determined in capillaries using a MEL-TEMP II apparatus (Aldrich) and are uncorrected. The IR spectra were recorded with a FT-IR NEXUS spectrophotometer as KBr pellets or as films, and the absorptions (ν) are given in cm-1. 1H-NMR and 13C-NMR spectra were recorded on a BRUKER AVANCE III instrument (1H at 600 and 13C at 150 MHz) in CDCl3 solutions; chemical shifts (δ) in ppm; coupling constants (J) in Hz. The multiplicity of the 13C signals was deduced from DEPT spectra, supported by 1H-13C HMQC spectra. The mass spectra were recorded on a Finnigan MAT-95 instrument using electrospray ionization (ESI); m/z (rel. %).
Starting materials. Used reagents such as alkylamines, carbonyl compounds, inorganic reagents, and solvents are commercially available and were used as received. α-(Hydroxyimino)ketones were obtained according to known protocols as follows: 3-(hydroxyimino)pentane-2,4-dione20a (8a) and ethyl 2-hydroxyimino-3-oxobutanoate20b (8b) were prepared from acetoacetate and ethyl acetoacetate, respectively, by treatment with NaNO2 in glacial AcOH; 2-hydroxyimino-3-oxo-N-phenylbutyramide20c (8c) was synthesized in a two step procedure by acetoacetylation of aniline and subsequent nitrosylation. The 2,2,4,4-tetramethylcyclobutane-1,3-dithione (7a), and 2,2,4,4-tetramethyl-3-thioxocyclobutanone (7b) were obtained by thionation of 2,2,4,4-tetramethylcyclobutane-1,3-dione with P4S10.21 The synthesis of 1-(adamantan-1-yl)-4,5-dimethyl-1H-imidazole 3-oxide (2e) and 1-(adamantan-1-yl)-4,5-diphenyl- 1H-imidazole 3-oxide (2f) was previously described.11
Synthesis of 4-acetylimidazole 3-oxides (2a–c). – General procedure. To a magnetically stirred solution of a 1,3,5-trisubstituted hexahydro-1,3,5-triazine (9a–c, 12 mmol) in Et2O (20 mL), a solution of 3-(hydroxyimino)pentane-2,4-dione (8a, 10 mmol, 1.3 g) in Et2O (10 mL) was added portionwise at 0 °C. After stirring for 20 h at rt, the solvent was removed under reduced pressure. The crude product (2a, 2b) was triturated with Et2O (4 × ca. 20 mL), cooled (in the fridge, 16 h), filtered, and purified by recrystallization. In the case of 2c, the crude product obtained as yellow oil was purified chromatographically (SiO2 column).
4-Acetyl-1,5-dimethyl-1H-imidazole 3-oxide (2a). Yield: 1.36 g (91%). Colorless crystals, mp 136–138 °C (CHCl3). IR (KBr): 3277vs (br.), 3106s, 1673vs (C=O), 1564s, 1404s, 1376m, 1365m, 1338m, 1262s, 1212m, 1030m, 960m. 1H-NMR (CDCl3): 7.77 (s, 1H, HC(2)); 3.57 (s, 3H, MeN); 2.83 (s, 3H, MeCO); 2.51 (s, 3H, Me). 13C-NMR (CDCl3): 191.3 (s, C=O); 132.2, 126.0 (2s, C(4), C(5)); 129.5 (d, C(2)); 32.0, 30.6, 9.9 (3q, 3 Me). ESI-HRMS: 177.0633 (calcd. 177.0634 for C7H10N2NaO2, [M+Na]+), 155.0816 (calcd. 155.0815 for C7H11N2O2, [M+1]+).
4-Acetyl-1-cyclohexyl-5-methyl-1H-imidazole 3-oxide (2b). Yield: 1.31 g (59%). Colorless crystals, mp 147–148 °C (CHCl3/Et2O). IR (KBr): 3067s, 2945vs, 2928vs, 2856s, 1667vs (C=O), 1566vs, 1450m, 1423s, 1366m, 1326s, 1254s, 1234m, 1123m, 1040m. 1H-NMR (CDCl3): 7.82 (s, 1H, HC(2)); 3.92–2.88 (m, 1H, cHex); 2.84, 2.52 (2s, 6H, 2 Me); 2.04–1.95 (m, 4H, cHex); 1.80–1.78 (m, 1H, cHex); 1.57–1.39 (m, 4H, cHex); 1.29–1.19 (m, 1H, cHex). 13C-NMR (CDCl3): 191.6 (s, C=O); 130.9, 123.2 (2s, C(4), C(5)); 129.1 (d, C(2)); 55.3 (d, CH, cHex); 33.4, 25.3, 24.7 (3d, 5CH2, cHex); 30.6, 11.2 (2q, 2 Me). ESI-HRMS: 245.1257 (calcd. 245.1260 for C12H18N2NaO2, [M+Na]+), 223.1440 (calcd. 223.1441 for C12H19N2O2, [M+1]+).
4-Acetyl-1-benzyl-5-methyl-1H-imidazole 3-oxide (2c). Yield after CC (SiO2, AcOEt, then AcOEt/MeOH 1:1): 1.31 g (57%). Pale yellow semi-solid. IR (KBr): 3061m, 2990m, 2958m, 2926m, 1663vs (C=O), 1563s, 1468m, 1457m, 1425m, 1406m, 1376m, 1366m, 1330m, 1268m, 1227m, 1125m, 702s. 1H-NMR (CDCl3): 7.86 (s, 1H, HC(2)); 7.35–7.31 (m, 3 arom. H); 7.09–7.08 (m, 2 arom. H); 5.00 (s, 2H, CH2); 2.77 (s, 3H, MeCO); 2.41 (s, 3H, Me). 13C-NMR (CDCl3): 191.3 (s, C=O); 133.1, 132.0, 125.9 (3s, C(Ph), C(4), C(5)); 129.7 (d, C(2)); 129.3, 128.9, 127.0 (3d, 5 arom. CH); 49.2 (t, CH2); 30.6, 10.1 (2q, 2 Me). ESI-HRMS: 253.09479 (calcd. 253.09475 for C13H14N2NaO2, [M+Na]+), 231.11294 (calcd. 231.11280 for C13H15N2O2, [M+1]+).
Synthesis of 1-(adamantan-1-yl)-5-methyl-1H-imidazole 3-oxides (2d, 2g and 2h). – General procedure. A solution of N-methylidene adamantan-1-amine (10, 12.0 mmol, 1.96 g) and a corresponding α-(hydroxyimino)ketone (8a-c, 10.0 mmol) in glacial AcOH (40 mL) was stirred for 24 h at rt. The resulting mixture was filtered, gaseous HCl was bubbled through the filtrate for 1.5 h at rt, and the solvent removed under reduced pressure. The crude hydrochloride was triturated with Et2O (3 × 20 mL), and the solid material was dissolved in CHCl3/MeOH (4:1, 30 mL). The resulting solution was treated with excess solid NaHCO3, stirred for 2 h, and filtered. Then, solvents were removed in vacuo.
4-Acetyl-1-(adamantan-1-yl)-5-methyl-1H-imidazole 3-oxide (2d). The crude product was washed with Et2O and used in the next step without further purification (attempted recrystallization from CHCl3 led to the isomeric imidazole-2-one). Yield: 0.90 g (33%). Colorless solid, mp 170–172 °C. IR (KBr): 2915vs, 2855m, 1672vs (C=O), 1636m, 1552vs, 1457m, 1405m, 1363m, 1248s, 1134m, 1091m. 1H-NMR (CDCl3): 7.91 (s, 1H, HC(2)); 2.80, 2.69 (2s, 6H, 2 Me); 2.20-2.28 (m, 3H, Ad); 2.14-2.20 (m, 6H, Ad); 1.76, 1.71 (AB-like, J = 12.7, 6H, Ad). 13C-NMR (CDCl3): 191.7 (s, C=O); 131.8, 125.0 (2s, C(4), C(5)); 129.6 (d, C(2)); 61.0 (s, C(Ad)); 41.6 (t, 3 CH2(Ad)), 35.3 (d, 3 CH(Ad)); 29.5 (t, 3 CH2(Ad)); 28.9, 13.5 (2q, 2 Me). ESI-HRMS: 297.15692 (calcd. 297.15735 for C16H22N2NaO2, [M+Na]+), 275.17507 (calcd. 275.117540 for C16H23N2O2, [M+1]+).
N-Phenyl-1-(adamantan-1-yl)-5-methyl-3-oxido-1H-imidazole-4-carboxamide (2g). The crude product was purified by flash chromatography (SiO2, CHCl3/MeOH 95:5, Rf = 0.50) and recrystallized. Yield: 1.65 g (47%). Colorless crystals, mp 228-231 °C (decomp.) (CH2Cl2/Et2O). IR (KBr): 2923s, 2906s, 2858m, 2848m, 1671s (br., C=O), 1621s, 1599vs, 1581s, 1566s, 1498m, 1447m, 1407m, 1302s, 1138m, 761m. 1H-NMR (CDCl3): 13.17 (br.s, 1H, NH); 8.04 (s, 1H, HC(2)); 7.71–7.69 (m, 2 arom. H); 7.33–7.30 (m, 2 arom. H); 7.09–7.06 (m, 1 arom. H); 2.94 (s, 3H, Me); 2.25-2.30 (m, 3H, Ad); 2.17-2.21, 2.23-2.27 (2m, 6H, Ad); 1.79, 1.74 (AB-like, J = 12.7, 6H, Ad). 13C-NMR (CDCl3): 158.0 (s, C=O); 138.2, 131.5, 122.6 (3s, C(Ph), C(4), C(5)); 128.8, 123.9, 123.6, 120.6 (4d, 5 arom. CH, C(2)); 60.9 (s, C(Ad)); 41.9 (t, CH2(Ad)), 35.5 (d, CH(Ad)); 29.6 (t, CH2(Ad)); 13.3 (q, Me). ESI-HRMS: 374.18377 (calcd. 374.18390 for C21H25N3NaO2, [M+Na]+), 352.20187 (calcd. 352.20195 for C21H26N3O2, [M+1]+).
Ethyl 1-(adamantan-1-yl)-5-methyl-3-oxido-1H-imidazole-4-carboxylate (2h). Yield after CC (SiO2, AcOEt/MeOH 1:1): 1.03 g (34%). Colorless crystals, mp 185-187 °C (capillary must be placed into apparatus, preheated to ca. 180 °C) (CH2Cl2/Et2O). IR (KBr): 2974m, 2912s (br.), 2859m, 1693vs (C=O), 1581m, 1412m, 1276s, 1258s, 1141m, 1119m. 1H-NMR (CDCl3): 8.04 (s, 1H, HC(2)); 4.42 (q, J = 7.1, 2H, MeCH2O); 2.70 (s, 3H, Me); 2.25-2.29 (m, 3H, Ad); 2.12-2.16, 2.20-2.24 (2m,, 6H, Ad); 1.78, 1.73 (AB-like, J = 12.6, 6H, Ad); 1.40 (t, J = 7.1, 3H, MeCH2O). 13C-NMR (CDCl3): 159.7 (s, C=O); 131.6, 123.6 (2s, C(4), C(5)); 124.9 (d, C(2)); 61.2 (t, MeCH2O); 60.8 (s, C(Ad)); 41.9 (t, 3 CH2(Ad)), 35.5 (d, 3 CH(Ad)); 29.6 (t, 3 CH2(Ad)); 14.3 (q, MeCH2O); 13.5 (q, Me). ESI-HRMS: 327.16779 (calcd. 327.16791 for C17H24N2NaO3, [M+Na]+), 305.18596 (calcd. 305.18597 for C17H25N2O3, [M+1]+).
Reactions of imidazole 3-oxides (2a–c,e,f) with 2,2,4,4-tetramethylcyclobuta-1,3-dithione (7a) leading to the corresponding imidazole-2-thiones (6). – General procedure. To the solution of an imidazole 3-oxide (2, 1.0 mmol) in CHCl3 (5.0 mL), the solution of 7a (103 mg, 0.6 mmol) in CHCl3 (3.0 mL) was added dropwise at 0 °C, and the mixture was stirred overnight at rt. After evaporation of the solvent under reduced pressure, the crude products were washed with hexane, filtered, and purified by recrystallization from appropriate solvents.
4-Acetyl-1,5-dimethylimidazole-2(3H)-thione (6a). Yield: 117 mg (69%). Colorless crystals, mp 241-243 °C (EtOH). IR (KBr): 3100–2900vs (br.), 1652vs (C=O), 1600m, 1490m, 1431m, 1407m, 1386m, 1370m, 1344m. 1H-NMR (CDCl3): 10.40 (br.s, 1H, NH); 3.60 (s, 3H, MeN); 2.52, 2.46 (2s, 6H, 2 Me). 13C-NMR (CDCl3): 185.3 (s, C=O); 163.5 (s, C=S); 133.9, 124.3 (2s, C(4), C(5)); 31.4, 28.2, 11.4 (3q, 3 Me). ESI-HRMS: 193.0407 (calcd. 193.0406 for C7H10N2NaOS, [M+Na]+), 171.0587 (calcd. 171.0587 for C7H11N2OS, [M+1]+).
4-Acetyl-1-cyclohexyl-5-methylimidazole-2(3H)-thione (6b). Yield: 140 mg (59%). Colorless needles, mp 215–217 °C (EtOH). IR (KBr): 3114vs, 2934vs, 2851s, 1670vs (C=O), 1588m, 1497s, 1414s, 1368m, 1327m, 1257m, 1230m, 1158m, 1143m, 951m. 1H-NMR (CDCl3): 10.96 (br.s, 1H, NH); 5.24 (m, 1H, cHex); 2.64, 2.47 (2s, 6H, 2 Me); 1.93-1.21 (m, 10H, cHex). 13C-NMR (CDCl3): 186.2 (s, C=O); 162.6 (s, C=S); 134.3, 124.5 (2s, C(4), C(5)); 57.9 (d, CH, cHex); 28.7, 26.1, 25.2 (3d, 5CH2, cHex); 30.3, 12.3 (2q, 2 Me). ESI-HRMS: 261.1032 (calcd. 261.1032 for C12H18N2NaOS, [M+Na]+), 239.1210 (calcd. 239.1213 for C12H19N2OS, [M+1]+).
4-Acetyl-1-benzyl-5-methylimidazole-2(3H)-thione (6c). Yield 177 mg (72%). Colorless solid, mp 225–226 °C (CHCl3/Et2O). IR (KBr): 3116s (br.), 3080m, 2962m, 1667vs (C=O); 1598s, 1504s, 1413s, 1372m, 1348m, 1272m, 731m. 1H-NMR (CDCl3): 10.29 (br.s, 1H, NH); 7.35–7.33 (m, 2 arom. H); 7.31–7.26 (m, 3 arom. H); 5.40 (s, 2H, CH2); 2.43, 2.38 (2s, 6H, 2 Me). 13C-NMR (DMSO-d6): 188.4 (s, C=O); 164.6 (s, C=S); 137.2, 135.9, 125.4 (3s, C(Ph), C(4), C(5)); 47.8 (t, CH2); 29.6, 12.2 (2q, 2 Me). ESI-HRMS: 269.07207 (calcd. 269.07190 for C13H14N2NaOS, [M+Na]+), 247.08994 (calcd. 247.08996 for C13H15N2OS, [M+1]+).
1-(Adamantan-1-yl)-4,5-dimethylimidazole-2(3H)-thione (6e). Yield: 185 mg (71%). Colorless solid, mp 320–322 °C (CHCl3). IR (KBr): 3158m, 3093m, 2916s, 2891m, 2855m, 1648m, 1501m, 1443m, 1366m, 1327m, 1309m, 1222s. 1H-NMR (CDCl3): 9.65 (br.s, 1H, NH); 2.72-2.76, 2.80-2.84 (2m, 6H, Ad); 2.23 (s, 3H, Me); 2.18-2.22 (m, 3H, Ad); 2.01 (s, 3H, Me); 1.81, 1.69 (AB-like, J = 11.4, 6H, Ad). 13C-NMR (CDCl3): 159.2 (s, C=S); 122.9, 120.6 (2s, C(4), C(5)); 64.3 (s, C(Ad)); 40.5 (t, 3 CH2(Ad)), 36.0 (d, 3 CH(Ad)), 30.3 (t, 3 CH2(Ad)), 14.9, 9.5 (2q, 2 Me). ESI-HRMS: 285.13946 (calcd. 285.13959 for C15H22N2NaS, [M+Na]+), 263.15760 (calcd. 263.15765 for C15H23N2S, [M+1]+).
1-(Adamantan-1-yl)-4,5-diphenylimidazole-2(3H)-thione (6f). Yield: 247 mg (64%). Colorless solid, mp 284–285 °C (CHCl3). IR (KBr): 3054m, 2909vs, 2851m, 1495m, 1445m, 1370m, 1326m, 1225m. 1H-NMR (CDCl3): 9.44 (br.s, 1H, NH); 7.41–7.31 (m, 5 arom. H); 7.17–7.14 (m, 3 arom. H); 6.91–6.89 (m, 2 arom. H); 2.23-2.57, 2.61-2.65 (2m, 6H, Ad); 2.02-2.06 (m, 3H, Ad); 1.67, 1.57 (AB-like, J = 11.4, 6H, Ad). 13C-NMR (CDCl3): 161.1 (s, C=S); 133.0, 128.2, 128.1, 126.0 (4s, 2 C(Ph), C(4), C(5)); 132.4, 129.1, 128.5, 128.4, 127.8, 126.7 (6d, 10 arom. CH); 65.6 (s, C(Ad)); 41.1 (t, 3 CH2(Ad)); 35.8 (d, 3 CH(Ad)); 30.4 (t, 3 CH2(Ad)). ESI-HRMS: 409.17050 (calcd. 409.17089 for C25H26N2NaS, [M+Na]+), 387.18886 (calcd. 387.18895 for C25H27N2S, [M+1]+).
Reactions of imidazole 3-oxides (2d,g,h) with 2,2,4,4-tetramethylcyclobuta-1,3-dithione (7a) leading to the corresponding imidazoles (11). – General procedure. To the solution of imidazole 3-oxide (1.0 mmol) in CHCl3 (5.0 mL), the solution of 7a (309 mg, 1.8 mmol) in CHCl3 (3.0 mL) was added dropwise at 0 °C, and the mixture was stirred at rt until the starting N-oxide was completely consumed (usually ca. 30 min, TLC monitoring: SiO2, AcOEt/MeOH 1:1; in the case of 2h, stirring was continued overnight). After evaporation of the solvent under reduced pressure, the crude products were washed with hexane, filtered, and purified by recrystallization from appropriate solvents.
4-Acetyl-1-(adamantan-1-yl)-5-methylimidazole (11d). Yield: 165 mg (64%). Colorless needles, mp 185–187 °C (MeOH/CH2Cl2). IR (KBr): 2920s, 2857m, 1667vs (C=O), 1538s, 1373m, 1265m, 1227m, 1146m, 921m. 1H-NMR (CDCl3): 7.53 (s, 1H, HC(2)); 2.80, 2.54 (2s, 6H, 2 Me); 2.21-2.27 (m, 3H, Ad); 2.20-2.26 (m, 6H, Ad); 1.78, 1.74 (AB-like, J = 12.5, 6H, Ad). 13C-NMR (CDCl3): 196.7 (s, C=O); 138.7, 134.3 (2s, C(4), C(5)); 133.2 (d, C(2)); 58.3 (s, C(Ad)); 42.0 (t, 3 CH2(Ad)); 35.7 (d, 3 CH(Ad)); 29.5 (t, 3 CH2(Ad)); 27.7, 13.7 (2q, 2 Me). ESI-HRMS: 281.16218 (calcd. 281.16243 for C16H22N2NaO, [M+Na]+), 259.18048 (calcd. 259.18049 for C16H23N2O, [M+1]+).
N-Phenyl-1-(adamantan-1-yl)-5-methylimidazole-4-carboxamide (11g). Yield 291 mg (87%). Colorless crystals, mp 189–191 °C (CH2Cl2/MeOH). IR (KBr): 3360m, 2911vs (br.), 2853m, 1671s, 1593s, 1569s, 1522s, 1498s, 1438s, 1310m, 1242m, 1149m, 750m, 692m. 1H-NMR (CDCl3): 9.16 (br.s, 1H, NH); 7.69–7.67 (m, 2 arom. H); 7.54 (s, 1H, HC(2)); 7.34–7.31 (m, 2 arom. H); 7.08–7.06 (m, 1 arom. H); 2.92 (s, 3H, Me); 2.24-2.30 (m, 3H, Ad); 2.23-2.29 (m, 6H, Ad); 1.80, 1.76 (AB-like, J = 12.6, 6H, Ad). 13C-NMR (CDCl3): 162.2 (s, C=O); 138.5, 133.4, 132.7 (3s, C(Ph), C(4), C(5)); 132.6 (d, C(2)); 128.8, 123.4, 119.5 (3d, 5 arom. CH); 58.5 (s, C(Ad)); 42.1 (t, 3 CH2(Ad)), 35.8 (d, 3 CH(Ad)); 29.6 (t, 3 CH2(Ad)); 13.3 (q, Me). ESI-HRMS: 358.18877 (calcd. 358.18898 for C21H25N3NaO, [M+Na]+), 336.20666 (calcd. 336.20704 for C21H26N3O, [M+1]+).
Ethyl 1-(adamantan-1-yl)-5-methylimidazole-4-carboxylate (11h). Yield 153 mg (53%). Colorless solid, mp 188–189 °C (CH2Cl2/hexane). IR (KBr): 2919vs, 2855m, 1697vs (C=O), 1554m, 1378m, 1368m, 1338m, 1306m, 1231s, 1204m, 1185s, 1153m, 1101m, 1075m. 1H-NMR (CDCl3): 7.61 (s, 1H, HC(2)); 4.35 (q, J = 7.0, 2H, MeCH2O); 2.81 (s, 3H, Me); 2.24-2.28 (m, 3H, Ad); 2.22-2.27 (m, 6H, Ad); 1.79, 1.76 (AB-like, J = 12.6, 6H, Ad); 1.39 (t, J = 7.0, 3H, MeCH2O). 13C-NMR (CDCl3): 164.2 (s, C=O); 135.7, 130.9 (2s, C(4), C(5)); 134.1 (d, C(2)); 60.0 (s, C(Ad)); 58.4 (t, MeCH2O); 42.1 (t, 3 CH2(Ad)), 35.8 (d, 3 CH(Ad)); 29.6 (t, 3 CH2(Ad)); 14.5 (q, MeCH2O); 13.3 (q, Me). ESI-HRMS: 289.19096 (calcd. 289.19105 for C17H25N2O2, [M+1]+).
Deoxygenation of 2h with Raney-nickel. To a magnetically stirred mixture of imidazole 3-oxide (2g, 281 mg, 0.80 mmol) in EtOH (45.0 mL), a suspension of freshly prepared Raney-nickel in EtOH was added in small portions at rt and stirring was continued until the starting material was completely consumed (TLC). The crude mixture was filtered through Celite, washed with several portions of CHCl3, the solvents of the filtrate were evaporated. The resulting residue was purified by preparative TLC (SiO2, CHCl3/MeOH 95:5, Rf = 0.88) to give 201 mg (75%) of 11g as colorless solid. The isolated material was identical with that obtained in the reaction of 2g with 7a.
Three-component (trapping) experiment. To the mixture of 7b (312 mg, 2.0 mmol) and thiobenzophenone (198 mg, 1.0 mmol) in CHCl3 (1.0 mL), a solution of imidazole 3-oxide (2d, 274 mg, 1.0 mmol) in CHCl3 (2.0 mL) was added dropwise at 0 °C and the mixture stirred for 3 h at rt. Then, the solvent was removed, the resulting mixture was treated with Et2O, and the colorless precipitate of crude 11d (172 mg, 67%) was filtered off. The solvent of the filtrate was evaporated, the resulting mixture was separated by PLC (SiO2) using CH2Cl2/petroleum ether 3:7 as the solvent to give 1,2,4-trithiolane (16, Rf = 0.75, 45 mg, 12%) as colorless solid. 1H-NMR (CDCl3): 7.62–7.60 (m, 4 arom. H); 7.31–7.28 (m, 6 arom. H); 1.51 (s, 6H, 2 Me); 1.42 (s, 6H, 2 Me).
ACKNOWLEDGEMENTS
The authors thank PD Dr. L. Bigler, University of Zürich, for ESI-HRMS. Financial support by the Rector of the University of Lodz (Grant # 505/712/R) and technical assistance in the laboratory work by Mrs Małgorzata Celeda, University of Lodz, are gratefully acknowledged.
References
1. Part of the Master Thesis of D. R., University of Lodz, 2010.
2. N. X. Huang and L. Liu, 'Compr. Heterocycl. Chem. III', Vol. 4, Chapter 4.02, ed. by A. R. Katritzky, C. A. Ramsden, E. F. V. Scriven, and R. J. K. Taylor, Pergamon Press, Oxford UK, 2008, pp. 143–364.
3. F. Bellina and R. Rossi, Adv. Synth. Catal., 2010, 352, 1223. CrossRef
4. a) I. J. Ferguson and K. Schofield, J. Chem. Soc., Perkin Trans. 1, 1975, 275; CrossRef b) R. Bartnik, W. E. Hahn, and G. Mloston, Rocz. Chem., 1977, 51, 49.
5. G. Mloston, T. Gendek, and H. Heimgartner, Tetrahedron, 2000, 56, 5405. CrossRef
6. a) G. Mloston, M. Jasinski, A. Linden, and H. Heimgartner, Helv. Chim. Acta, 2006, 89, 1304; CrossRef b) R. Loska and M. Makosza, Mendeleev Commun., 2006, 161; CrossRef c) R. Loska and M. Makosza, Chem. Eur. J., 2008, 14, 2577. CrossRef
7. G. Mloston, T. Gendek, and H. Heimgartner, Helv. Chim. Acta, 1998, 81, 1585. CrossRef
8. a) M. Jasinski, G. Mloston, P. Mucha, A. Linden, and H. Heimgartner, Helv. Chim. Acta, 2007, 90, 1765; CrossRef b) P. Mucha, G. Mloston, M. Jasinski, A. Linden, and H. Heimgartner, Tetrahedron: Asymmetry, 2008, 19, 1600. CrossRef
9. a) W. A. Herrmann, Angew. Chem. Int. Ed., 2002, 41, 1290; CrossRef b) D. Bourissou, O. Guerret, F. P. Gabbaï, and G. Bertrand, Chem. Rev., 2000, 100, 39. CrossRef
10. M. Jasinski, G. Mloston, A. Linden, and H. Heimgartner, Helv. Chim. Acta, 2008, 91, 1916. CrossRef
11. G. Mloston and M. Jasinski, ARKIVOC, 2011, (vi), 162.
12. a) R. Huisgen, G. Mloston, K. Polborn, and F. Palacios-Gambra, Liebigs Ann./Rec., 1997, 187; b) W. Adam, R. M. Bargon, and G. Mloston, Eur. J. Org. Chem., 2003, 4012. CrossRef
13. G. Mloston and H. Heimgartner, Helv. Chim. Acta, 1995, 78, 1298. CrossRef
14. R. Huisgen and J. Rapp, Tetrahedron, 1997, 53, 939. CrossRef
15. a) R. Huisgen and E. Langhals, Tetrahedron Lett., 1989, 30, 5369; CrossRef b) R. Huisgen and E. Langhals, Heteroatom Chem., 2006, 17, 433; CrossRef c) R. Huisgen, L. Fisera, H. Giera, and R. Sustmann, J. Am. Chem. Soc., 1995, 117, 9671. CrossRef
16. a) D. S. C. Black and K. G. Watson, Aust. J. Chem., 1973, 26, 2491; CrossRef b) see also in a review: D. S. C. Black, R. F. Crozier, and V. Ch. Davis, Synthesis, 1975, 205. CrossRef
17. R. Sustmann, W. Sicking, and R. Huisgen, J. Am. Chem. Soc., 1995, 117, 9679. CrossRef
18. a) H. Giera, R. Huisgen, E. Langhals, and K. Polborn, Helv. Chim. Acta, 2002, 85, 1523; CrossRef b) G. Mloston, R. Huisgen, H. Giera, and E. Langhals, Tetrahedron, 2002, 58, 507. CrossRef
19. P. R. Schreiner, H. P. Reisenauer, J. Romanski, and G. Mloston, J. Am. Chem. Soc., 2010, 132, 7240. CrossRef
20. a) X.-H. Cai, H.-J. Yang, and G.-L. Zhang, Synthesis, 2005, 10, 1569; CrossRef b) I. R. Corrêa Jr. and P. J. S. Moran, Tetrahedron, 1999, 55, 14221; CrossRef c) G. Mloston and M. Jasinski, Collect. Czech. Chem. Commun., 2010, 75, 871. CrossRef
21. E. V. Elam and H. W. Davis, J. Org. Chem., 1967, 32, 1562. CrossRef