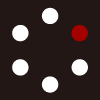
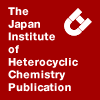
HETEROCYCLES
An International Journal for Reviews and Communications in Heterocyclic ChemistryWeb Edition ISSN: 1881-0942
Published online by The Japan Institute of Heterocyclic Chemistry
e-Journal
Full Text HTML
Received, 6th January, 2011, Accepted, 3rd February, 2011, Published online, 14th February, 2011.
DOI: 10.3987/COM-11-S(P)3
■ Synthesis of Stereodefined 3,4-Disubstituted Piperidines through Rearrangement of 2-(2-Bromo-1,1-dimethylethyl)azetidines
Karen Mollet, Leen Broeckx, Matthias D’hooghe,* and Norbert De Kimpe*
Faculty of Bioscience Engineering, Department of Sustainable Organic Chemistry and Technology, Ghent University, Coupure Links 653, B-9000 Ghent, Belgium
Abstract
cis-2-(2-Bromo-1,1-dimethylethyl)azetidines, synthesized by monochloroalane reduction of the corresponding β-lactams, were shown to be excellent building blocks for the synthesis of stereodefined 4-bromo-, 4-fluoro-, 4-acetoxy- and 4-hydroxypiperidines in DMSO. During these transformations, the initially formed bicyclic azetidinium intermediates underwent ring opening by a variety of nucleophiles.INTRODUCTION
As substituted six-membered azaheterocycles are among the most common building blocks in natural products and biologically active compounds,1 the preparation of piperidine-based organic scaffolds has been widely studied.2 To date, their synthesis still represents a major challenge in medicinal chemistry, as more and more complex piperidine-containing compounds are designed in order to improve the selectivity and reduce the side effects of potential new drugs.
Ring enlargements of nitrogen heterocycles comprise very useful reactions because they can provide a straightforward and efficient access to different nitrogen-containing target molecules.3 These reactions frequently involve strained nitrogen ring systems in which strain release acts as a driving force for the ring enlargement. As a result, substituted azetidines have for example been proven to be suitable starting materials to perform rearrangements towards pyrroles, pyrrolidines, pyrrolidinones, imidazolidinones, isoxazolidines, piperidines, 1,2-oxazines, piperidin-2-ones, 2-iminopiperidines, azepanes and azepan-2-ones.4 Moreover, the introduction of halogens in the substituents of these small-ring heterocycles creates a number of possibilities for intramolecular transformations towards bicyclic azetidinium ions, which are subsequently prone to undergo ring opening (mostly implying ring expansion) by the halogen or an additional nucleophile.4a,5 Although the four- to five-membered ring expansion of halogenated azetidines is well known, 4a,5 the corresponding four- to six-membered ring enlargement is much less studied.5d
In the present work, the scope and synthetic applicability of the latter methodology is examined towards the preparation of novel piperidines by treatment of functionalized azetidines with different nucleophiles. In particular, special attention is directed towards the synthesis of 4-fluorinated piperidines, as these compounds have become increasingly popular as building blocks towards bioactive compounds.
In general, fluorinated azaheterocyclic compounds attract widespread attention as important key structural features in pharmaceutical chemistry and agrochemistry. This is mainly due to the fact that the replacement of a hydrogen atom with fluorine often gives rise to drastic changes in biological activity because of the altered electronic distribution and changes in conformational properties.6,7 During the last decades, much effort has been devoted to synthesize various site-specific fluorinated azaheterocyclic compounds, and the development of new synthetic approaches and new commercial applications are the subject of intense research in organic chemistry and related disciplines.8 In particular, a rapidly growing interest in fluorinated piperidines exists, as these compounds possess interesting biological activities. The numerous patents concerning fluorinated piperidines emphasize the possibility of these compounds as substituents to modulate the activity of different active compounds, such as antidepressants,9 antibacterial agents,10 anti-inflammatory and immunomodulatory agents10,11 and compounds for the treatment of neurological and psychiatric diseases. 12
In continuation of our interest in the use of 4-(haloalkyl)azetidin-2-ones as versatile synthons,13 the applicability of these easily available β-lactams for the stereoselective preparation of novel 3,4-disubstituted piperidines via 2-(2-bromo-1,1-dimethylethyl)azetidines is investigated in this paper. Special attention is hereby directed towards the straightforward and stereoselective formation of valuable 4-fluoropiperidines.
RESULTS AND DISCUSSION
N-(3-Bromo-2,2-dimethylpropylidene)alkylamines 2 were synthesized through condensation of 3-bromo-2,2-dimethylpropanal 1 with 1.2 equiv of the corresponding primary amine in CH2Cl2 at room temperature (or at reflux in case of a bulky tert-butyl substituent) in the presence of MgSO4 as drying agent (Scheme 1).14 3-Bromo-2,2-dimethylpropanal 1 was prepared through oxidation of 3-bromo-2,2-dimethylpropan-1-ol with pyridinium chlorochromate mixed with silica in 98% yield.15 Subsequently, β-bromoimines 2 were used as substrates for the Staudinger synthesis of β-lactams, affording cis-4-(2-bromo-1,1-dimethylethyl)azetidin-2-ones 3a-d upon treatment with 1.3 equiv of benzyloxyacetyl chloride or phenoxyacetyl chloride in CH2Cl2 in the presence of Et3N as a base (Scheme 1, Table 1).16 This reaction concerns a [2+2] cycloaddition of imines 2 with the ketenes in situ generated from benzyloxy- and phenoxyacetyl chloride.
It should be noted that the Staudinger synthesis of β-lactams 3 proceeded in a highly diastereoselective way, which can be attributed to the electron-donating benzyloxy or phenoxy group present in the Boose-Evans ketenes, favouring direct conrotatory ring closure of the zwitterionic intermediates towards cis-β-lactams.17 The cis-stereochemistry could be deduced from the 1H NMR spectra of β-lactams 3, as the coupling constants between the 3-H and 4-H protons on the β-lactam ring varied between 5.3 and 5.8 Hz (CDCl3), which corresponds well with those reported in the literature for cis-β-lactams.18
In the next part, our interest was directed towards the reduction of the carbonyl moiety in the latter β-lactams 3 upon treatment with monochloroalane (AlH2Cl), as this method had already been proven to be a suitable method for the synthesis of functionalized azetidines.19 Also in the present report, reductions of highly functionalized β-lactams were performed successfully in that respect. Treatment of 4-(2-bromo-1,1-dimethylethyl)azetidin-2-ones 3 with one molar equiv of AlH2Cl, prepared in situ from 3 molar equiv of LiAlH4 and one equiv of AlCl3, in Et2O at 0 °C for 2 hours furnished the corresponding 2-(2-bromo-1,1-dimethylethyl)azetidines 4a-d5d in good yields (Scheme 2, Table 2). It was necessary to perform an inverse addition by adding 4-(2-bromo-1,1-dimethylethyl)azetidin-2-ones 3 to one molar equiv of AlH2Cl in diethyl ether.
This reaction proceeded with retention of the stereochemistry as defined during the Staudinger synthesis of β-lactams 3. The obtained cis-stereochemistry of azetidines 4 was proven by the observation that in all cases the vicinal coupling constants (7.0–7.3 Hz, 1H NMR, CDCl3) between the 2-H and 3-H protons were similar to coupling constants found in the literature for azetidines with analogous stereochemistry.19c
Careful monitoring of the reaction conditions proved to be very important during this monochloroalane-mediated reduction, as minor changes (e.g. in terms of equiv, reaction temperature and reaction time) gave rise to complex reaction mixtures or incomplete conversion of the starting material. Moreover, it has to be noted that prolonged storage of azetidines 4 at room temperature or even at -18 °C led to the formation of small amounts of ring-expanded 4-bromo-5,5-dimethylpiperidines 6, which can be explained considering the formation and subsequent ring opening of intermediate 1-azoniabicyclo[2.2.0]hexanes 5 (Scheme 3), pointing to the relative instability of γ-bromoamines 4. For example, storage of azetidine 4c for 22 days at -18 °C resulted in the detection of 4% of piperidine 6c, and standing at room temperature for 13 hours of azetidine 4d furnished the corresponding piperidine 6d in 40% through spontaneous rearrangement.
Azetidines are an important class of azaheterocyclic systems, as the strained four-membered ring is found in many naturally occuring organic compounds with interesting biological and pharmacological properties.20 In addition, as mentioned before, azetidines have been shown to be excellent building blocks in organic synthesis.4a Also, 2-(2-bromo-1,1-dimethylethyl)azetidines 4 have previously been proven to be very useful intermediates towards the synthesis of stereodefined 4-hydroxy-, 4-cyano- and 4-azidopiperidines upon treatment with NaOH, KCN and NaN3 in DMSO.5d
Fluorinated piperidines bearing additional functional groups at the heterocyclic ring are important building blocks in pharmaceutical chemistry and agrochemistry because of their interesting biological properties.8-12 In that respect, several attempts were made in order to perform a ring enlargement of 2-(2-bromo-1,1-dimethylethyl)azetidines 4 as a convenient synthetic approach towards new 4-fluorinated piperidines. In a first approach, treatment of the latter compounds with tetrabutylammonium fluoride (TBAF or Bu4NF) was examined, as nucleophilic fluorinations with TBAF are well known in the literature.21 When azetidines 4 were treated with 10 equiv of TBAF in DMSO for 18 h at 100 °C, cis-4-fluoro-5,5-dimethylpiperidines 7 were obtained successfully. However, as TBAF is known to decompose at elevated temperatures,22 significant amounts of decomposed byproducts were present in the 1H NMR spectra of the crude reaction mixtures. Alternatively, treatment of cis-2-(2-bromo-1,1-dimethylethyl)azetidines 4 with 2 equiv of tetramethylammonium fluoride (TMAF or Me4NF) afforded cis-4-fluoro-5,5-dimethylpiperidines 7a-d in good yields and high purity after purification by column chromatography on silica gel (Scheme 4, Table 3). Nonetheless, small amounts of piperidine enol ethers were observed as well in the reaction mixtures, most probably resulting from a fluoride-induced dehydrobromination of the corresponding 4-bromopiperidines 6.
The cis-stereochemistry of piperidines 7 was experimentally confirmed through analysis of the vicinal coupling constants between the protons at C-3 and C-4 (1.8-2.2 Hz, CDCl3). These values are in accordance with coupling constants described in the literature for cis-4-fluoro-3-hydroxypiperidines (1.8-5.5 Hz, CDCl3),23 while the corresponding trans-4-fluoro-3-hydroxypiperidines have 3J-values of 2.5-4.3 Hz (CDCl3) for equatorial vicinal protons and 8.4-12.3 Hz (CDCl3) for axial vicinal protons.23a Considering the two possible chair conformations of the six-membered heterocyclic ring of piperidines 7 (Scheme 5), the predominant conformation was determined through analysis of the vicinal coupling pattern between the proton at position 3 and the fluoro atom at position 4. The observed 3JH-F-values of 27.7-28.6 Hz (1H NMR, CDCl3) imply axial positions for both the proton at C3 and the fluoro atom at C4 (chair conformation 8), as similar coupling constants have been described in the literature for analogous conformations.23a Moreover, a Karplus-type equation, describing the relation between vicinal proton-fluorine coupling constants and the corresponding H-C-C-F torsion angles, predicts a 3JH-F-value of 33.3-43.2 Hz for a trans-diaxial relationship between the two involved nuclei for a temperature range of 278-358 K (chair conformation 8),24 while equatorial positions (chair conformation 9) correspond with vicinal coupling constants between 9.5 Hz and 24.3 Hz.24
The observed cis-stereochemistry of piperidines 7 was rationalized considering the in situ formation and consecutive ring opening of bicyclic azetidinium intermediates 5 (Scheme 4). This reaction mechanism is based on the intramolecular displacement of bromide by the nucleophilic nitrogen lone pair of azetidines 4 towards reactive bicyclic intermediates 5, which are subsequently prone to undergo ring opening by the nucleophilic counterion, i.e. fluoride, at the bridgehead carbon atom in a SN2 fashion to furnish the thermodynamically more favoured six-membered piperidines 7 (Scheme 4).5d
The presence of a monofluorinated carbon center was unambiguously assigned based on the coupling constants between the proton and the fluoro atom at C4, as the observed J-values of 50.1-51.8 Hz (1H NMR, CDCl3) correspond well with those reported in the literature (46.4-54.0 Hz, 1H NMR, CDCl3).25 Also, the 13C NMR spectra revealed a coupling between the carbon and the fluorine at the C4-position, characterized by J-values between 179.9 and 182.3 Hz (13C NMR, CDCl3). These results are in good accordance with literature data.23b,25b
In order to provide additional evidence for this stereospecific azetidine to piperidine rearrangement, 4-bromopiperidine 6c as a selected example was transformed into the corresponding enol ether 10 through dehydrobromination, a process which requires a cis-stereochemistry to enable a trans-elimination. Thus, treatment of cis-4-bromo-1-isopropyl-5,5-dimethyl-3-phenoxypiperidine 6c, prepared according to a literature protocol5d involving ring enlargement of cis-2-(2-bromo-1,1-dimethyl)-1-isopropyl-3-phenoxyazetidine 4c upon stirring in acetonitrile under reflux, with 4 equiv of dimsyl sodium in DMSO resulted in the formation of 1-isopropyl-5,5-dimethyl-3-phenoxy-1,2,5,6-tetrahydropyridine 10 in 44% yield (Scheme 6).
To broaden the scope of the above-described nucleophile-induced ring transformation of 2-(2-bromoethyl)azetidines 4 towards novel stereodefined piperidines, the feasibility of introducing other nucleophiles than bromine, fluorine, hydroxide, cyanide and azide was evaluated by employing sodium acetate. Thus, treatment of azetidines 4 with 10 equiv of NaOAc in DMSO at 100 °C for 18 h resulted in the selective formation of cis-4-acetoxy-5,5-dimethylpiperidines 11a-d in good yields (Scheme 7, Table 4). Again, the relative cis-stereochemistry controlled by the Staudinger synthesis of β-lactams 3 was transferred through the reaction sequence, affording piperidines 11 in a stereoselective way as demonstrated by the vicinal coupling constants between the protons at C3 and C4 (3.0-3.3 Hz, 1H NMR, CDCl3), which are in accordance with literature data concerning 3,4-dioxygenated piperidines.23b,26
The synthetic relevance of these novel cis-4-acetoxypiperidines 11 was demonstrated by means of their transformation into the biologically important class of 4-hydroxylated piperidines,27-29 producing the corresponding cis-4-hydroxypiperidines 12 upon hydrolysis of the ester moiety by means of 3 equiv of LiOH in methanol (Scheme 7, Table 4).
In accordance with a literature protocol concerning a one-step ring enlargement of 2-(haloalkyl)azetidines into 4-hydroxypiperidines by means of sodium hydroxide,5d different attempts were made to prepare the latter 4-hydroxypiperidines 12 selectively through NaOH- and/or H2O-mediated ring transformation of 2-(2-bromoethyl)azetidines 4 (Scheme 8). Thus, azetidines 4 were subjected to different reaction conditions, involving multiple variation of the number of equiv of NaOH and/or H2O (1-15 equiv), reaction time (3-19 h) and reaction temperature (80-90 °C). In all cases, the competition between hydroxide and bromide to induce ring enlargement resulted in a mixture of 4-hydroxypiperidines 12 and 4-bromopiperidines 6 (20-71/29-80, Scheme 8). Moreover, purification of piperidines 12 by column chromatography on silica gel was hampered by the similar retention time of 4-hydroxypiperidines 12 and 4-bromopiperidines 6, resulting in low isolated yields of 4-hydroxypiperidines 12 (28-49%).
As a result, it can be concluded that the two-step synthesis of 4-hydroxypiperidines 12 via 4-acetoxypiperidines 11 comprises an improved alternative in terms of selectivity and efficiency.
In summary, 2-(2-bromo-1,1-dimethylethyl)azetidines were proven to be useful starting materials to perform rearrangements towards substituted six-membered azaheterocycles. These reactions involve the intermediacy of 1-azoniabicyclo[2.2.0]hexanes, which are subsequently prone to undergo a nucleophile-induced ring enlargement towards a wide variety of highly functionalized piperidines. In particular, this methodology allowed the development of novel stereodefined 4-fluoro- and 4-hydroxypiperidines, which are nowadays of high importance in pharmaceutical chemistry and agrochemistry.
EXPERIMENTAL
1. General
1H NMR spectra were recorded at 300 MHz (JEOL ECLIPSE+) with tetramethylsilane as internal standard. 13C NMR spectra were recorded at 75 MHz (JEOL ECLIPSE+). Mass spectra were recorded on an Agilent 1100 series mass spectrometer using a direct inlet system (electron spray, 4000 V). IR spectra were recorded on a Perkin-Elemer Spectrum BX FT-IR spectrometer. All compounds were analysed in neat form with an ATR (Attenuated Total Reflectance) accessory. Melting points were measured using a Büchi B-540 apparatus and are uncorrected. Dichloromethane was distilled over calcium hydride, while diethyl ether and THF were distilled from sodium and sodium benzophenone ketyl before use. Other solvents were used as received from the supplier.
2. Synthesis of cis-1-alkyl-4-(2-bromo-1,1-dimethylethyl)-β-lactams 316
General procedure: To an ice-cooled solution of N-(3-bromo-2,2-dimethylpropylidene)alkylamine 214 (10 mmol) and triethylamine (30 mmol) in CH2Cl2 (25 mL) was added dropwise a solution of benzyloxy- or phenoxyacetyl chloride (13 mmol) in CH2Cl2 (10 mL). After stirring for 15 h at room temperature, the reaction mixture was poured into water (30 mL) and extracted with CH2Cl2 (2 × 25 mL). Drying (MgSO4), filtration of the drying agent, and removal of the solvent afforded cis-1-alkyl-4-(2-bromo-1,1-dimethylethyl)-β-lactam 3, which was further purified by column chromatography on silica gel.
2.1. cis-4-(2-Bromo-1,1-dimethylethyl)-1-isopropyl-3-phenoxyazetidin-2-one 3c
White crystals. Mp 60.1-62.1 °C. Yield 80%. Rf 0.23 (hexane/EtOAc 9/1). 1H NMR (300 MHz, CDCl3): δ 1.23 and 1.25 (2×3H, 2×s), 1.32 and 1.48 (2×3H, 2×d, J=6.9 Hz), 3.39 (1H, d, J=10,2 Hz), 3.53 (1H, septet, J=6.9 Hz), 3.69 (1H, d, J=10.2 Hz), 4.02 (1H, d, J=5.5 Hz), 5.16 (1H, d, J=5.5 Hz), 6.97-7.02, 7.09-7.12 and 7.25-7.30 (5H, 3×m). 13C NMR (75 MHz, CDCl3): δ 20.61, 20.80, 22.64, 24.35, 37.23, 43.96, 47.59, 63.47, 80.58, 116.04, 122.33, 129.49, 157.72, 166.99. IR (ATR): ν=1749 cm-1 (C=O). MS: m/z (%): 340/2 (M++1, 100). Anal. Calcd for C16H22BrNO2: C 56.48, H 6.52, N 4.12. Found: C 56.58, H 6.91, N 4.13.
2.2. cis-4-(2-Bromo-1,1-dimethylethyl)-1-cyclohexyl-3-phenoxyazetidin-2-one 3d
White crystals. Mp 77.2 °C. Yield 55%. Rf 0.36 (hexane/EtOAc 9/1). 1H NMR (300 MHz, CDCl3): δ 1.25 and 1.29 (2×3H, 2×s), 1.21-1.29, 1.64-1.92 and 2.01-2.07 (10H, 3×m), 3.08-3.18 (1H, m), 3.41 and 3.72 (2×1H, 2×d, J=9.9 Hz), 4.07 (1H, d, J=5.5 Hz), 5.20 (1H, d, J=5.5 Hz), 6.99-7.04, 7.10-7.14 and 7.22-7.33 (5H, 3×m). 13C NMR (75 MHz, CDCl3): δ 22.90, 24.65, 25.29, 25.43, 25.92, 30.77, 31.20, 37.57, 44.16, 55.97, 63.57, 80.64, 116.21, 122.49, 129.67, 157.93, 167.12. IR (ATR): ν=1737 cm-1 (C=O). MS: m/z (%): 380/2 (M++1, 100). Anal. Calcd for C19H26BrNO2: C 60.00, H 6.89, N 3.68. Found: C 59.77, H 6.92, N 3.72.
3. Synthesis of cis-1-alkyl-2-(2-bromo-1,1-dimethylethyl)azetidines 419
General procedure: To a solution of aluminium(III) chloride (10 mmol) in dry Et2O (50 mL) was added carefully lithium aluminium hydride (30 mmol) at 0 °C. The reaction mixture was stirred at room temperature for 1 h. Subsequently, a solution of cis-1-alkyl-4-(2-bromo-1,1-dimethylethyl)azetidine-2-one 3 (10 mmol) in dry Et2O (15 mL) was added slowly and, after the addition was complete, the reaction mixture was stirred for 2 h at 0 °C, after which water (10 mL) was added cautiously at 0 °C in order to neutralize the excess of LiAlH4. Afterwards, the reaction mixture was filtered and extracted with Et2O (3 × 25 mL). Drying (MgSO4), filtration of the drying agent, and removal of the solvent afforded cis-1-alkyl-2-(2-bromo-1,1-dimethylethyl)azetidine 4, which was further purified by column chromatography on silica gel.
3.1. cis-2-(2-Bromo-1,1-dimethylethyl)-1-isopropyl-3-phenoxyazetidine 4c
Colourless oil. Yield 60%. Rf 0.13 (hexane/EtOAc 19/1). 1H NMR (300 MHz, CDCl3): δ 0.98 and 1.01 (2×3H, 2×d, J=6.4 Hz), 1.21 and 1.24 (2×3H, 2×s), 2.92 (1H, septet, J=6.4 Hz), 3.23 (1H, d×d×d, J=9.1, 4.1, 1.3 Hz), 3.45 (1H, d×d, J=9.1, 7.0 Hz), 3.57 (1H, d, J=9.6 Hz), 3.65 (1H, d×d, J=7.0, 1.3 Hz), 3.83 (1H, d, J=9.6 Hz), 4.91 (1H, d×d×d, J=7.0, 7.0, 4.1 Hz), 6.72-6.78, 6.91-7.02 and 7.21-7.33 (5H, 3×m). 13C NMR (75 MHz, ref=CDCl3): δ 14.91, 21.32, 24.07, 24.15, 38.23, 45.97, 51.22, 51.33, 68.84, 70.64, 114.95, 121.09, 129.62, 157.36. IR (ATR): νmax=2963, 2930, 1599, 1494, 1238, 906, 751, 730 cm-1. MS: m/z (%): 326/8 (M++1, 40), 246 (100). Anal. Calcd for C19H26BrNO2: C 58.90, H 7.41, N 4.29. Found: C 59.13, H 7.72, N 4.19.
3.2. cis-2-(2-Bromo-1,1-dimethylethyl)-1-cyclohexyl-3-phenoxyazetidine 4d
Colourless oil. Yield 66%. Rf 0.21 (hexane/EtOAc 19/1). 1H NMR (300 MHz, CDCl3): δ 1.20 and 1.23 (2×3H, 2×s), 1.12-1.31, 1.58-1.68, 1.71-1.94 (10H, 3×m), 2.39-2.50 (1H, m), 3.28 (1H, d×d, J=9.2, 4.5 Hz), 3.51 (1H, d×d, J=9.2, 7.2 Hz), 3.57 (1H, d, J=9.9 Hz), 3.76 (1H, d, J=7.3 Hz), 3.83 (1H, d, J=9.9 Hz), 4,93 (1H, d×d×d, J=7.3, 7.2, 4.5 Hz), 6.72-6.87, 6.91-6.97 and 7.20-7.29 (5H, 3×m). 13C NMR (75 MHz, ref=CDCl3): δ 24.10, 24.16, 25.43, 25.98, 26.18, 26.36, 31.86, 38.41, 46.03, 52.88, 60.50, 68.33, 70.9, 115.01, 121.15, 129.68, 157.45. IR (ATR): νmax=2926, 2853, 1598, 1493, 1237, 751 cm-1. MS: m/z (%): 366/8 (M++1, 100). Anal. Calcd for C19H28BrNO: C 62.29, H 7.70, N 3.82. Found: C 62.37, H 7.94, N 3.75.
4. Synthesis of cis-4-fluoro-5,5-dimethylpiperidines 7
General procedure: To a solution of cis-1-alkyl-2-(2-bromo-1,1-dimethylethyl)azetidine 4 (10 mmol) in DMSO (50 mL) was added Me4NF·4H2O (20 mmol) at room temperature. After stirring at 100 °C for 18 h, the reaction mixture was poured into water (40 mL) and extracted with Et2O (3 × 25 mL). Afterwards, the organic phase was washed intensively with brine (4 × 30 mL). Drying (MgSO4), filtration of the drying agent, and removal of the solvent afforded cis-4-fluoro-5,5-dimethylpiperidine 7, which was further purified by column chromatography on silica gel.
4.1. cis-1-Allyl-3-benzyloxy-4-fluoro-5,5-dimethylpiperidine 7a
Yellow oil. Yield 56%. Rf 0.12 (hexane/EtOAc 24/1). 1H NMR (300 MHz, CDCl3): δ 0.98 (3H, s (br)), 1.00 (3H, d, J=1.1 Hz), 2.07 and 2.24 (2×1H, 2×d, J=11.6 Hz), 2.26 (1H, d×d, J=11.3, 11.2 Hz), 2.86 (1H, d×d, J=11.3, 4.9 Hz), 2.96 (1H, d×d, J=13.8, 6.5 Hz), 3.04 (1H, d×d, J=13.8, 6.2 Hz), 3.75 (1H, d×d×d×d, J=28.4, 11.2, 4.9, 1.8 Hz), 4.32 (1H, d×d, J=51.5, 1.8 Hz), 4.60 and 4.64 (2×1H, 2×d, J=12.1 Hz), 5.08-5.21 (2H, m), 5.80 (1H, d×d×d×d, J=17.0, 10.5, 6.5, 6.2 Hz), 7.27-7.38 (5H, m). 19F NMR (282 MHz, CDCl3): δ (-205.18)-(-206.66) (m). 13C NMR (75 MHz, CDCl3): δ 23.71 (d, J=7.0 Hz), 23.93 (d, J=5.8 Hz), 35.34 (d, J=17.3 Hz), 52.13, 58.93, 61.13, 70.87, 73,22 (d, J=17,3 Hz), 93,78 (d, J=181,2 Hz), 117,49, 127,69, 128,39, 135.31, 138.19. IR (ATR): νmax=2953, 2923, 2871, 2806, 1117, 1101, 1068, 981, 919, 735, 697 cm-1. MS: m/z (%): 278 (M++1, 100). Anal. Calcd for C17H24FNO: C 73.61, H 8.72, N 5.05. Found: C 73.39, H 8.91, N 5.11.
4.2. cis-3-Benzyloxy-1-tert-butyl-4-fluoro-5,5-dimethylpiperidine 7b
Yellow crystals. Mp 35.2 °C. Yield 61%. Rf 0.18 (hexane/EtOAc 41/1). 1H NMR (300 MHz, CDCl3): δ 0.96 (3H, s(br)), 0.99 (3H, d, J=1.7 Hz), 1.02 (9H, s), 2.19 (1H, d, J=13.2 Hz), 2.24-2.36 (2H, m), 2.97 (1H, d×d, J=9.9, 4.4 Hz), 3.67 (1H, d×d×d×d, J=28.6, 10.4, 4.4, 1.9 Hz), 4.32 (1H, d×d, J=51.8, 1.9 Hz), 4.58 and 4.65 (2×1H, 2×d, J=11.8 Hz), 7.27-7.41 (5H, m). 19F NMR (282 MHz, CDCl3): δ -205.95 (s(br)). 13C NMR (75 MHz, ref=CDCl3): δ 23.66 (d, J=7.0 Hz), 24.12 (d, J=6.9 Hz), 26.50, 35.65 (d, J=17.4 Hz), 45.26, 52.13, 53.40, 70.91, 74.68 (d, J=17.3 Hz), 94.05 (d, J=179.9 Hz), 127.74, 127.83, 128.49, 138.49. IR (ATR): νmax=2971, 1100, 1080, 970 cm-1. MS: m/z (%): 294 (M++1, 100). Anal. Calcd for C18H28FNO: C 73.68, H 9.62, N 4.77. Found: C 73.91, H 9.88, N 4.54.
4.3. cis-4-Fluoro-1-isopropyl-5,5-dimethyl-3-phenoxypiperidine 7c
Yellow oil. Yield 45%. Rf 0.07 (hexane/EtOAc 33/1). 1H NMR (300 MHz, CDCl3): δ 1.01 and 1.02 (2×3H, 2×d, J=6.6 Hz), 1.02 (3H, s(br)), 1.10 (3H, d, J=1.1 Hz), 2.17 (1H, d, J=11.0 Hz), 2.37 (1H, d×d, J=11.0, 1.4 Hz), 2.60 (1H, d×d×d, J=10.4, 10.4, 1.4 Hz), 2.80 (1H, septet, J=6.6 Hz), 2.91 (1H, d×d, J=10.4, 4.9 Hz), 4.42 (1H, d, J=50.1 Hz), 4.55 (1H, d×d×d×d, J=27.7, 10.4, 4.9, 2.2 Hz), 6.94-7.08 and 7.26-7.32 (5H, 2×m). 19F NMR (282 MHz, CDCl3): δ (-205.22)-(-206.90) (m). 13C NMR (75 MHz, CDCl3): δ 17.63, 18.57, 23.49, 23.93 (2×d, J=5.8 Hz), 35.77 (d, J=17.3 Hz), 47.42, 53.79, 54.22, 72.94 (d, J=17.3 Hz), 94.12 (d, J=181.1 Hz), 116.07, 121.35, 129.60, 157.37. IR (ATR): νmax=2966, 2928, 2851, 1640, 1611, 1495, 1454, 1350, 1320, 750 cm-1. MS: m/z (%): 266 (M++1, 100), 246 (70). Anal. Calcd for C16H24FNO: C 72.42, H 9.12, N 5.28. Found: C 72.20, H 9.41, N 5.44.
4.4. cis-1-Cyclohexyl-4-fluoro-5,5-dimethyl-3-phenoxypiperidine 7d
White crystals. Mp 67.3-68.8 °C. Yield 49%. Rf 0.17 (hexane/EtOAc 24/1). 1H NMR (300 MHz, CDCl3): δ 1.01 and 1.09 (2×3H, 2×s), 1.14-1.29, 1.55-1.65 and 1.71-1.84 (10H, 3×m), 2.21 (1H, d, J=11.3 Hz), 2.26-2.40 (1H, m), 2.45 (1H, d, J=11.3 Hz), 2.67 (1H, d×d, J=10.5, 10.5 Hz), 2.95 (1H, d×d, J=10.5, 5.0 Hz), 4.41 (1H, d, J=51.7 Hz), 4.45-4.53 and 4.54-4.62 (1H, 2×m), 6.94-7.01 and 7.24-7.31 (5H, 2×m). 19F NMR (282 MHz, CDCl3): δ (-205.40)-(-207.30) (m). 13C NMR (75 MHz, CDCl3): δ 23.51 (d, J=6.9 Hz), 23.89 (d, J=5.8 Hz), 25.96, 26.03, 26.38, 28.51, 29.25, 35.89 (d, J=17.3 Hz), 47.84, 54.69, 63.45, 73.07 (d, J=17.3 Hz), 94.23 (d, J=182.3 Hz), 116.08, 121.34, 129.60, 157.41. IR (ATR): νmax=2925, 2851, 2360, 1718, 1599, 1492, 1239, 1050, 751, 732, 691 cm-1. MS: m/z (%): 306 (M++1, 100). Anal. Calcd for C19H28FNO: C 74.72, H 9.24, N 4.59. Found: C 74.84, H 9.39, N 4.68.
5. Synthesis of 1-isopropyl-5,5-dimethyl-3-phenoxy-1,2,5,6-tetrahydropyridine 10
To a solution of cis-4-bromo-1-isopropyl-5,5-dimethyl-3-phenoxypiperidine 6c (10 mmol) in DMSO (50 mL) was added NaH (40 mmol, 60% dispersion in mineral oil), after which the resulting suspension was stirred for 15 h at 100 °C. Subsequently, the reaction mixture was poured into water (40 mL) and extracted with Et2O (3 × 25 mL). Afterwards, the organic phase was washed intensively with brine (4 × 30 mL). Drying (MgSO4), filtration of the drying agent, and removal of the solvent afforded 1-isopropyl-5,5-dimethyl-3-phenoxy-1,2,5,6-tetrahydropyridine 10, which was further purified by column chromatography on silica gel (hexane/EtOAc 33/1).
Yellow oil. Yield 44%. Rf 0.19 (hexane/EtOAc 33/1). 1H NMR (300 MHz, CDCl3): δ 1.01 (6H, s), 1.05 (6H, d, J=6.6 Hz), 2.27 (2H, s), 2.80 (1H, septet, J=6.6 Hz), 3.10 (2H, d, J=1.1 Hz), 4.76 (1H, s(br)), 6.98-7.09 and 7.27-7.35 (5H, 2×m). 13C NMR (75 MHz, CDCl3): δ 18.32, 28.09, 32.67, 49.42, 53.47, 58.66, 114.74, 118.80, 122.82, 129.47, 150.41, 156.18. IR (ATR): ν=1678 cm-1 (C=C). MS: m/z (%): 246 (M++1, 100). Anal. Calcd for C16H23NO: C 78.32, H 9.45, N 5.71. Found: C 78.63, H 9.67, N 5.55.
6. Synthesis of cis-4-acetoxy-1-alkyl-5,5-dimethylpiperidines 11
General procedure: To a solution of cis-1-alkyl-2-(2-bromo-1,1-dimethylethyl)azetidine 4 (10 mmol) in DMSO (50 mL) was added NaOAc (100 mmol). After stirring at 100 °C for 18 h, the reaction mixture was poured into water (40 mL) and extracted with Et2O (3 × 25 mL). Afterwards, the organic phase was washed intensively with brine (4 × 30 mL). Drying (MgSO4), filtration of the drying agent, and removal of the solvent afforded cis-4-acetoxy-1-alkyl-5,5-dimethylpiperidine 11, which was further purified by column chromatography on silica gel.
6.1. cis-4-Acetoxy-1-allyl-3-benzyloxy-5,5-dimethylpiperidine 11a
Colourless oil. Yield 64%. Rf 0.08 (hexane/EtOAc 10/1). 1H NMR (300 MHz, CDCl3): δ 0.88 and 1.07 (2×3H, 2×s), 2.06 (1H, d, J=11.0 Hz), 2.11 (3H, s), 2.22-2.26 (2H, m), 2.76-2.78 (1H, m), 2.95 (1H, d×d, J=13.8, 6.3 Hz), 3.04 (1H, d×d, J=13.8, 6.1 Hz), 3.80 (1H, d×d×d, J=10.5, 4.7, 3.0 Hz), 4.43 and 4.65 (2×1H, 2×d, J=11.9 Hz), 5.10-5.20 (3H, m), 5.81 (1H, m), 7.23-7.36 (5H, m). 13C NMR (75 MHz, ref=CDCl3): δ 21.03, 24.35, 24.88, 35.22, 53.28, 59.91, 61.37, 70.84, 73.02, 73.59, 117.50, 127.64, 127.82, 128.43, 135.56, 138.46, 170.52. IR (ATR): ν=1739 cm-1 (C=O). MS: m/z (%): 318 (M++1, 100). Anal. Calcd for C19H27NO3: C 71.89, H 8.57, N 4.41. Found: C 72.08, H 8.43, N 4.29.
6.2. cis-4-Acetoxy-3-benzyloxy-1-tert-butyl-5,5-dimethylpiperidine 11b
Colourless oil. Yield 71%. Rf 0.11 (hexane/EtOAc 6/1). 1H NMR (300 MHz, CDCl3): δ 0.87 (3H, s), 1.03 (9H, s), 1.05 (3H, s), 2.11 (3H, s), 2.18 (1H, d, J=11.0 Hz), 2.28-2.32 (2H, m), 2.87-2.95 (1H, m), 3.72 (1H, d×d×d, J=10.4, 4.7, 3.0 Hz), 4.40 and 4.66 (2×1H, 2×d, J=11.6 Hz), 5.09 (1H, s(br)), 7.28-7.36 (5H, m). 13C NMR (75 MHz, ref=CDCl3): δ 21.09, 24.42, 24.76, 26.55, 35.13, 46.39, 53.02, 53.29, 70.88, 73.89, 74,43, 127.59, 127.85, 128.43, 138.60, 170.65. IR (ATR): ν=1737 cm-1 (C=O). MS: m/z (%): 334 (M++1, 100). Anal. Calcd for C20H31NO3: C 72.04, H 9.37, N 4.20. Found: C 71.83, H 9.65, N 4.12.
6.3. cis-4-Acetoxy-1-isopropyl-5,5-dimethyl-3-phenoxypiperidine 11c
Colourless oil. Yield 62%. Rf 0.09 (hexane/EtOAc 14/1). 1H NMR (300 MHz, CDCl3): δ 0.92 (3H, s), 1.01 (6H, d, J=6.6 Hz), 1.13 (3H, s), 2.09 (3H, s), 2.14 and 2.34 (2×1H, 2×d, J=11.3 Hz), 2.61 (1H, d×d, J=10.2, 10.0 Hz), 2.72-2.85 (2H, m), 4.60 (1H, d×d×d, J=10.0, 4.5, 3.2 Hz), 5.05 (1H, s(br)), 6.81-6.95 and 7.21-7.28 (5H, 2×m). 13C NMR (75 MHz, CDCl3): δ 17.77, 18.43, 20.90, 23.85, 24.75, 35.32, 48.67, 54.20, 55.13, 72.83, 75.06, 116.07, 121.20, 129.46, 157.78, 170.45. IR (ATR): ν=1742 cm-1. MS: m/z (%): 306 (M++1, 100). Anal. Calcd for C18H27NO3: C 70.79, H 8.91, N 4.59. Found: C 70.62, H 9.14, N 4.77.
6.4. cis-4-Acetoxy-1-cyclohexyl-5,5-dimethyl-3-phenoxypiperidine 11d
Colourless oil. Yield 72%. Rf 0.20 (hexane/EtOAc 19/1). 1H NMR (300 MHz, CDCl3): δ 0.91 and 1.13 (2×3H, 2×s), 1.16-1.31, 1.55-1.65 and 1.68-1.87 (10H, 3×m), 2.09 (3H, s), 2.19 (1H, d, J=11.3 Hz), 2.27-2.38 (1H, m), 2.42 (1H, d, J=11.3 Hz), 2.68 (1H, d×d, J=10.2, 9.7 Hz), 2.87 (1H, d×d, J=10.2, 4.1 Hz), 4.59 (1H, d×d×d, J=9.7, 4.1, 3.3 Hz), 5.04 (1H, s(br)), 6.79-6.97 and 7.15-7.29 (5H, 2×m). 13C NMR (75 MHz, CDCl3): δ 20.90, 23.80, 24.75, 25.91, 25.99, 26.40, 28.58, 29,12, 35.41, 49.07, 56.00, 63.41, 72.92, 75.20, 116.08, 121.18, 129.46, 157.80, 170.50. IR (ATR): ν=1744 cm-1 (C=O). MS: m/z (%): 346 (M++1, 100). Anal. Calcd for C21H31NO3: C 73.01, H 9.04, N 4.05. Found: C 72.83, H 9.39, N 4.26.
7. Synthesis of cis-1-alkyl-4-hydroxy-5,5-dimethylpiperidines 12
General procedure: To a solution of cis-4-acetoxy-1-alkyl-5,5-dimethylpiperidine 11 (10 mmol) in methanol (50 mL) was added LiOH·H2O (30 mmol). After a reflux period of 15 h, the solvent was removed in vacuo and the residue was extracted with Et2O (1 × 30 mL) and water (2 × 30 mL). The aqueous phase was washed with Et2O (2 × 25 mL). Drying (MgSO4), filtration of the drying agent, and removal of the solvent afforded cis-1-alkyl-4-hydroxy-5,5-dimethylpiperidine 12, which was further purified by column chromatography on silica gel.
7.1. cis-1-Allyl-3-benzyloxy-4-hydroxy-5,5-dimethylpiperidine 12a
Colourless oil. Yield 62%. Rf 0.05 (hexane/EtOAc 6/1). 1H NMR (300 MHz, CDCl3): δ 1.00 (2×3H, s), 2.14 (2H, s), 2.23-2.35 (1H, m), 2.72 (1H, d×d, J=9.4, 4.5 Hz), 2.93 (1H, d×d, J=13.8, 5.9 Hz), 3.02 (1H, d×d, J=13.8, 5.7 Hz), 3.53 (1H, s(br)), 3.80 (1H, d×d×d, J=10.1, 4.5, 3.2 Hz), 4.58 (2H, s), 5.10-5.20 (2H, m), 5.81 (1H, m), 7.27-7.38 (5H, m). 13C NMR (75 MHz, CDCl3): δ 24.18, 24.80, 35.25, 51.82, 59.09, 61.26, 70.54, 72.83, 74.75, 117.15, 127.52, 127.66, 128.34, 135.53, 138.10. IR (ATR): ν=3558 cm-1 (OH). MS: m/z (%): 276 (M++1, 100). Anal. Calcd for C17H25NO2: C 74.14, H 9.15, N 5.09. Found: C 74.01, H 9.32, N 4.93.
7.2. cis-3-Benzyloxy-1-tert-butyl-4-hydroxy-5,5-dimethylpiperidine 12b
Yellow oil. Yield 28%. Rf 0.10 (hexane/EtOAc 4/1). 1H NMR (300 MHz, CDCl3): δ 0.97 and 0.99 (2×3H, 2×s), 1.02 (9H, s), 2.19 and 2.27 (2×1H, 2×d, J=11.0 Hz), 2.26-2.42 and 2.77-2.88 (2×1H, 2×m), 3.51 (1H, d, J=2.2 Hz), 3.69-3.75 (1H, m), 4.56 and 4.58 (2×1H, 2×d, J=11.6 Hz), 7.25-7.38 (5H, m). 13C NMR (75 MHz, ref=CDCl3): δ 24.25, 24.85, 26.45, 35.35, 45.02, 52.35, 53.45, 70.75, 73.19, 76.23, 127.77, 127.88, 128.58, 138.34. IR (ATR): ν=3404 cm-1 (OH). MS: m/z (%): 292 (M++1, 100). Anal. Calcd for C18H29NO2: C 74.18, H 10.03, N 4.81. Found: C 74.44, H 10.29, N 4.62.
7.3. cis-4-Hydroxy-1-isopropyl-5,5-dimethyl-3-phenoxypiperidine 12c
White crystals. Mp 81.5-83.5 °C. Yield 86%. Rf 0.11 (hexane/EtOAc 9/1). 1H NMR (300 MHz, CDCl3): δ 0.99 and 1.00 (2×3H, 2×d, J=6.3 Hz), 1.02 and 1.08 (2×3H, 2×s), 2.07 and 2.41 (2×1H, 2×d, J=11.1 Hz), 2.24 (1H, s(br)), 2.61 (1H, d×d, J=10.2, 9.8 Hz), 2.67-2.83 (2H, m), 3.62 (1H, d, J=3.0 Hz), 4.58 (1H, d×d×d, J=9.8, 4.6, 3.0 Hz), 6.87-7.00 and 7.27-7.32 (5H, 2×m). 13C NMR (75 MHz, CDCl3): δ 17.53, 18.61, 24.12, 24.78, 35.50, 47.32, 53.85, 54.20, 74.02, 74.63, 116.19, 121.49, 129.63, 157.15. IR (ATR): ν=3197 cm-1 (OH). MS: m/z (%): 264 (M++1, 100). Anal. Calcd for C16H25NO2: C 72.96, H 9.57, N 5.32. Found: C 72.94, H 9.77, N 5.27.
7.4. cis-1-Cyclohexyl-4-hydroxy-5,5-dimethyl-3-phenoxypiperidine 12d
Colourless oil. Yield 71%. Rf 0.19 (hexane/EtOAc 14/1). 1H NMR (300 MHz, CDCl3): δ 1.01 and 1.08 (2×3H, 2×s), 1.12-1.29, 1.56-1.63 and 1.68-1.81 (10H, 3×m), 2.11 (1H, d, J=11.0 Hz), 2.20-2.34 (1H, m), 2.49 (1H, d, J=11.0 Hz), 2.68 (1H, d×d, J=10.2, 9.9 Hz), 2.80 (1H, d×d, J=10.2, 4.4 Hz), 3.61 (1H, d, J=2.6 Hz), 4.56 (1H, d×d×d, J=9.9, 4.4, 2.6 Hz), 6.92-6.98 and 7.24-7.30 (5H, 2×m). 13C NMR (75 MHz, CDCl3): δ 24.09, 24.80, 25.96, 26.06, 26.41, 29.28, 29.71, 35.62, 47.68, 54.78, 63.45, 74.11, 74.72, 116.19, 121.46, 129.61, 157.19. IR (ATR): ν=3589 cm-1 (OH). MS: m/z (%): 304 (M++1, 100). Anal. Calcd for C19H29NO2: C 75.21, H 9.63, N 4.62. Found: C 75.36, H 9.82, N 4.77.
ACKNOWLEDGEMENTS
The authors are indebted to Ghent University (GOA) and the Fund for Scientific Research-Flanders (FWO-Vlaanderen) for financial support.
References
1. (a) F.-X. Felpin and J. Lebreton, Eur. J. Org. Chem., 2003, 3693; CrossRef (b) D. O’Hagan, Nat. Prod. Rep., 1997, 14, 637; CrossRef (c) D. O’Hagan, Nat. Prod. Rep., 2000, 17, 435; CrossRef (d) S. A. M. W. van den Broek, P. G. W. Rensen, F. L. van Delft, and F. P. J. T. Rutjes, Eur. J. Org. Chem., 2010, 5906. CrossRef
2. M. G. P. Buffat, Tetrahedron, 2004, 60, 1701. CrossRef
3. M. Hesse, Ring Enlargements in Organic Chemistry I, 1991.
4. (a) F. Couty, F. Durrat, and G. Evano, Targets in Heterocyclic Systems, 2005, 9, 186; (b) T. M. Bott, J. A. Vanecko, and F. G. West, J. Org. Chem., 2009, 74, 2832; CrossRef (c) W. Van Brabandt, Y. Dejaegher, R. Van Landeghem, and N. De Kimpe, Org. Lett., 2006, 8, 1101; CrossRef (d) J. A. Vanecko and F. G. West, Org. Lett., 2005, 7, 2949; CrossRef (e) F. Couty, F. Durrat, G. Evano, and J. Marrot, Eur. J. Org. Chem., 2006, 4214. CrossRef
5. (a) F. Couty, F. Durrat, and D. Prim, Tetrahedron Lett., 2003, 44, 5209; CrossRef (b) F. Couty and M. Kletskii, J. Molec. Struct., 2009, 908, 26; (c) S. Dekeukeleire, M. D’hooghe, K. W. Törnroos, and N. De Kimpe, J. Org. Chem., 2010, 75, 5934; CrossRef (d) W. Van Brabandt, R. Van Landeghem, and N. De Kimpe, Org. Lett., 2006, 8, 1105; CrossRef (e) G. R. Krow, G. Lin, K. P. Moore, A. M. Thomas, C. DeBrosse, C. W. Ross, and H. G. Ramjit, Org. Lett., 2004, 6, 1669; CrossRef (f) F. Outurquin, X. Pannecoucke, B. Berthe, and C. Paulmier, Eur. J. Org. Chem., 2002, 1007. CrossRef
6. D. O’Hagan, Chem. Soc. Rev., 2008, 37, 308. CrossRef
7. S. Purser, P. R. Moore, S. Swallow, and V. Gouverneur, Chem. Soc. Rev., 2008, 37, 320. CrossRef
8. (a) G. Verniest, R. Surmont, E. Van Hende, A. Deweweire, and N. De Kimpe, J. Org. Chem., 2008, 73, 5458; CrossRef (b) E. Van Hende, G. Verniest, J. W. Thuring, G. Macdonald, F. Deroose, and N. De Kimpe, Synlett, 2009, 1765; CrossRef (c) R. Surmont, G. Verniest, A. Deweweire, J. W. Thuring, G. Macdonald, F. Deroose, and N. De Kimpe, Synlett, 2009, 1933; CrossRef (d) R. Surmont, G. Verniest, J. W. Thuring, G. Macdonald, F. Deroose, and N. De Kimpe, J. Org. Chem., 2010, 75, 929; CrossRef (e) R. Surmont, G. Verniest, J. W. Thuring, P. ten Holte, F. Deroose, and N. De Kimpe, Org. Biomol. Chem., 2010, 8, 4514. CrossRef
9. J. L. Maurel, J.-M. Autin, P. Funes, A. Newman-Tancredi, F. Colpaert, and B. Vacher, J. Med. Chem., 2007, 50, 5024. CrossRef
10. .
11. R. J. Friary, J. A. Kozlowski, B. B. Shankar, M. K. C. Wong, G. Zhou, B. J. Lavey, N.-Y. Shih, L. Tong, L. Chen, and Y. Shu, PCT Int. Appl. WO/2003/042174, 2003.
12. Z.-Q. Yang, J. C. Barrow, W. D. Shipe, K. S. Schlegel, Y. Shu, F. V. Yang, C. W. Lindsley, K. E. Rittle, M. G. Bock, G. D. Hartman, V. N. Uebele, C. E. Nus, S. V. Fox, R. L. Kraus, S. M. Doran, T. M. Connolly, C. Tang, J. E. Ballard, Y. Kuo, E. D. Adarayan, T. Prueksaritanont, P. B. Bunting, R. F. Woltmann, M. M. Magee, K. S. Koblan, and J. Renger, J. Med. Chem., 2008, 51, 6471. CrossRef
13. (a) M. D’hooghe, S. Dekeukeleire, E. Leemans, and N. De Kimpe, Pure Appl. Chem., 2010, 82, 1749; CrossRef (b) S. Dekeukeleire, M. D’hooghe, K. W. Törnroos, and N. De Kimpe, J. Org. Chem., 2010, 75, 5934. CrossRef
14. (a) P. Sulmon, N. De Kimpe, R. Verhé, L. De Buyck, and N. Schamp, Synthesis, 1986, 192; CrossRef (b) P. Sulmon, N. De Kimpe, N. Schamp, B. Tinant, and J.-P. Declercq, Tetrahedron, 1988, 44, 3653. CrossRef
15. (a) E. J. Corey and J. W. Suggs, Tetrahedron Lett., 1975, 31, 2647; CrossRef (b) E. Leemans, M. D’hooghe, Y. Dejaegher, K. W. Törnroos, and N. De Kimpe, Eur. J. Org. Chem., 2010, 352. CrossRef
16. Y. Dejaegher and N. De Kimpe, J. Org. Chem., 2004, 69, 5974. CrossRef
17. (a) L. Jiao, Y. Liang, and J. Xu, J. Am. Chem. Soc., 2006, 128, 6060; CrossRef (b) J. Xu, ARKIVOC, 2009, 21.
18. (a) K. D. Barrow and T. M. Spotswood, Tetrahedron Lett., 1965, 6, 3325; CrossRef (b) J. Decazes, J. L. Luche, and H. B. Kagan, Tetrahedron Lett., 1970, 11, 3361. CrossRef
19. (a) Y. Dejaegher, S. Mangelinckx, and N. De Kimpe, J. Org. Chem., 2002, 67, 2075; CrossRef (b) I. Ojima, M. Zhao, T. Yamato, and K. Nakahashi, J. Org. Chem., 1991, 56, 5263; CrossRef (c) B. Alcaide, P. Almendros, C. Aragoncillo, and N. R. Salgado, J. Org. Chem., 1999, 64, 9596. CrossRef
20. (a) N. De Kimpe, In Comprehensive Heterocyclic Chemistry II, 1996, 1B, 507; (b) N. H. Cromwell and B. Philips, Chem. Rev., 1979, 79, 331; CrossRef (c) J. A. Moore and R. S. Ayers, Chemistry of Heterocyclic Compounds-Small Ring Heterocycles, 1983, 2, 1. CrossRef
21. (a) K. L. Kirk, Org. Process Res. Dev., 2008, 12, 305; CrossRef (b) M. D’hooghe and N. De Kimpe, Synlett, 2006, 2089. CrossRef
22. (a) H. Sun and S. G. DiMagno, J. Am. Chem. Soc., 2005, 127, 2050; CrossRef (b) R. K. Sharma and J. L. Fry, J. Org. Chem., 1983, 48, 2112. CrossRef
23. (a) A. Arnone, P. Bravo, A. Donadelli, and G. Resnati, Tetrahedron, 1996, 52, 131; CrossRef (b) R. H. Furneaux, G. J. Gainsford, J. M. Mason, and P. C. Tyler, Tetrahedron, 1997, 53, 245. CrossRef
24. C. Thibaudeau, J. Plavec, and J. Chattopadhyaya, J. Org. Chem., 1998, 63, 4967. CrossRef
25. (a) D. J. Hallett, U. Gerhard, S. C. Goodacre, L. Hitzel, T. J. Sparey, S. Thomas, and M. Rowley, J. Org. Chem., 2000, 65, 4984; CrossRef (b) Y. Kishi, S. Inagi, and T. Fuchigami, Eur. J. Org. Chem., 2009, 103. CrossRef
26. L. Bartali, A. Casini, A. Guarna, E. G. Occhiato, and D. Scarpi, Eur. J. Org. Chem., 2010, 5831. CrossRef
27. H. Vanderhaeghe, G. Janssen, and F. Compernolle, Tetrahedron Lett., 1971, 12, 2687. CrossRef
28. A. Aiello, E. Fattorusso, A. Giordano, M. Menna, W. E. G. Müller, S. Perovic-Ottstadt, and H. C. Schröder, Bioorg. Med. Chem., 2007, 15, 5877. CrossRef
29. B. Ho and T. M. Zabriskie, Bioorg. Med. Chem. Lett., 1998, 8, 739. CrossRef