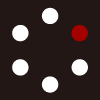
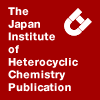
HETEROCYCLES
An International Journal for Reviews and Communications in Heterocyclic ChemistryWeb Edition ISSN: 1881-0942
Published online by The Japan Institute of Heterocyclic Chemistry
e-Journal
Full Text HTML
Received, 21st January, 2011, Accepted, 7th March, 2011, Published online, 10th March, 2011.
DOI: 10.3987/COM-11-12147
■ Synthesis of Various Substituted Spiro- and Bicyclethiazolidine-2-thiones by a Multicomponent Reaction and Biological Evaluation in vitro
Xiao-Juan Wang, Hai-Wei Xu, Lin-Lin Guo, En Zhang, Guang-Wei Chen, Xiao Guo, and Hong-Min Liu*
New Drug Research & Development Center, Zhengzhou University, Zhengzhou 450002, China
Abstract
A series of novel spiro[4.4] and bicycle[3.3.0]-N-substituted thiazolidine-2-thiones were obtained by a multicomponent reaction under mild conditions. Each of the synthesized compounds was evaluated for their cytotoxic activity and antibacterial activity. Some of them exhibited significantly inhibited proliferation of human tumor cell lines and moderate inhibitory effect on fungi.A major challenge of modern drug discovery is the design of highly efficient chemical reaction sequences which provide a maximum of structural complexity and diversity with just a minimum number of synthetic steps to assemble compounds with interesting properties.1 Among organic reactions, multicomponent reactions (MCRs) are highly convergent.2 During a multicomponent reaction, more than two starting materials are assembled to afford a complex product. Due to atom economy, simplicity and amenability to automated synthesis, MCRs constitute a superior tool for diversity oriented and complexity-generating synthesis for drug discovery.3
Heterocyclic structures received special attention as they belong to a class of compounds with proven utility in medicinal chemistry.4 There are numerous biologically active molecules with five-membered rings, containing two hetero atoms. Thiazolidine-2-thione (Figure 1) is an important scaffold found in numerous natural products and pharmaceuticals,5 and they have been identified as anti-HIV, antimitotic, anti-cancer and antibiotic agents.6 Therefor, their derivatives have been extensively studied. In addition, they are also versatile auxiliaries in many useful asymmetric organic reactions.7
Different approaches have been reported for the preparation of thiazolidine-2-thione unit,8 including β-aminoalkyl hydrogen sulfates with strong bases,9 cyclization of 1-isothiocyanato-2-thiocyanate,10 condensation of β-amino alcohols with carbon disulfide,11 especially a simple three-component reaction involving 1,2-addition of dithiocarbamate intermediates with a ketone.12 Despite these achievements, the one-pot multicomponent approach which constructs spiro[4.4] and bicycle[3.3.0]-N-substituted thiazolidine-2-thiones has not been documented to the best of our knowledge.
Butenolides and butyrolactones are ubiquitious chemical moieties found in many natural products and synthetic bioactive molecules.13 On the other hand, the conjugate addition of nucleophiles to α,β-unsaturated carbonyl groups is a very versatile synthetic reaction that successfully yields new carbon-carbon or carbon-hetero atom bonds.14 Based on our previous work on butenolides15 and the interest in the discovery of organic compounds with potential biological activity, we herein disclose a MCR of carbon disulfide, primary amine, γ-bromo-α,β-unsaturated ester or ketone giving novel spiro- [4.4] and bicycle[3.3.0]skeletons constituted by thiazolidine-2-thione in high yields (Scheme 1). The adducts thus obtained underwent simple chemical transformations16 providing a convenient, efficient, and practical synthetic approach to a wide range of thiazolidine-2-thiones.
In order to merge fragment of two biologically interesting motifs, initial experiments was designed to synthesize dithiocarbamates bearing butenolide (7, in Scheme 2) by the reaction of carbon disulfide, amines and 3-(bromomethyl)-butenolide 1 in the presence of Na3PO4·11H2O,17 aiming to discover some active lead compounds. Fortunately, a new framework of spirothiazolidine-2-thiones 2a-b was obtained during the reaction of compound 1 with methylamine or ethylamine (Scheme 2). Notably, no dithiocarbamate products similar to compounds 7a-b were formed.
The facile construction of spirothiazolidine-2-thiones via this multicomponent reaction and the vantage point of their potential biological activities aroused our great interest.18 With this method, various primary amines, including aliphatic amines and aromatic amines used in this reaction, and a series of novel spiro- [4.4]-N-substituted thiazolidine-2-thiones were synthesized (Table 1).
However, not all the primary are suitable for this reaction, the electronic properties and the position of the substitutions on the benzene ring of aniline have significant effect on the reaction, such as anilines substituted by nitryl or carboxyl could not react. This phenomenon probably resulted from the decrease of nucleophilicity of the nitrogen atom.
The structures of spiro[4.4]-N-substituted thiazolidine-2-thiones products 2a-j were assigned on the basis of spectroscopic analysis. In addition, a single-crystal X-ray analysis of 2f19 further provided the exclusively solid evidence for the assigned structure (Figure 2).
According to the literatures,20 a possible mechanism for the synthesis of compound 2a-j was postulated. First, the reaction was initiated with the formation of the dithiocarbamates, which led to spiro-[4.4]-N-substituted thiazolidine-2-thiones through hetero-Michael cycloaddition. The reaction process was so smooth that the dithiocarbamate intermediate couldn’t be detected.
Based on the mechanism proposed above, the substrate scope and generality of this reaction system were further explored. 4-bromo-3-methylcyclopent-2-enone 3 and 5-bromo-4-methyl butenolide 5 were tested under the standard reaction conditions (Table 2). Both 3 and 5 reacted with primary amines and carbon disulfide, affording cis-fused bicyclic thiazolidine-2-thiones 4 and 6 in 70-90% yields. The cis fused-ring fromation in 4 was supported by their 1H NMR and NOESY analysis. The excellent diastereoselectivity was attributed to the well-known thermodynamic bias for a cis ring fusion in [3.3.0]bicycles.21
More important, all of these reactions can complete in half an hour under the condition mentioned above. Most products could be obtained by recrystallization in chloroform/methanol (1:1). These features has provided access to chemical libraries and enhanced the work efficiency.
The inhibitory effects of the above spiro and bicyclo thiazolidine-2-thiones on different human tumors growth in vitro were examined.22 Some of them showed significantly inhibited proliferation of EC9706, PC-3, Hela and SPCA-1 cell lines. Especially the compounds 2d (mean IC50 = 18.03 µM, Hela cell) and 4b (mean IC50 = 61.57 µM, SPCA-1 cell). These preliminary results indicated that the newly synthesized thiazolidine-2-thiones might be used for further development in drug discovery. In addition, their antibacterial activity was aslo evaluated,23 but most of them showed unconspicuous inhibitory activity on bacterium and moderate inhibitory effect on fungi.
In conclusion, we report a facile construction of spiro[4.4] and bicyclo[3.3.0]thiazolidine-2-thiones by a MCR. This method provides the basis for the construction of compound libraries and the screening of lead compounds. Further investigations including scope, biological activity and chiral separation of various substituted thiazolidine-2-thiones compouds are currently in progress.
ACKNOWLEDGEMENTS
We are grateful for the financial support from the National Natural Science Foundation of China (Project No. 20902086), as well as support from China Postdoctoral Science Foundation funded project (Project No. 20080430864).
References
1. C. Hulme and T. Nixey, Curr. Opin. Drug Discovery Dev., 2003, 6, 921; A. Dömling, Curr. Opin. Chem. Biol., 2002, 6, 306; CrossRef S. L. Schreiber, Science, 2000, 287, 1964. CrossRef
2. B. Beck, S. Hess, and A. Dömling, Biorg. Med. Chem. Lett., 2000, 10, 1701. CrossRef
3. A. Dömling, Chem. Rev., 2006, 106, 17; CrossRef R. V. A. Orru and M. de Greef, Synthesis, 2003, 1471; CrossRef B. Beck, M. M. Lachaux, E. Herdtweck, and A. Dömling, Org. Lett., 2001, 3, 2875; CrossRef A. Dömling and I. Ugi, Angew. Chem. Int. Ed., 2000, 39, 3168; CrossRef B. M. Trost, Angew. Chem., Int. Ed. Engl., 1995, 34, 259. CrossRef
4. L. A. Thompson and J. A. Ellman, Chem. Rev., 1996, 96, 555; CrossRef J. S. Fruchtel and G. Jung, Angew. Chem., Int. Ed. Engl., 1996, 35, 17; CrossRef A. Nefzi, J. M. Ostresh, and R. A. Houghten, Chem. Rev., 1997, 97, 449; CrossRef R. G. Frazen, J. Comb. Chem., 2000, 2, 195. CrossRef
5. A. Verma and S. K. Saraf, Eur. J. Med. Chem., 2008, 43, 897; CrossRef A. Geronikaki, P. Eleftheriou, P. Vicini, I. Alman, A. Dixit, and A. K. Saxena, J. Med. Chem., 2008, 51, 5221. CrossRef
6. L. Tao, J. T. Xu, D. Gell, and T. P. Davis, Macromolecules, 2010, 43, 3721; CrossRef A. R. Katritzky, S. R. Tala, H. Lu, A. V. Vakulenko, Q. Y. Chen, J. Sivapackiam, K. Pandya, S. B. Jiang, and A. K. Debnath, J. Med. Chem., 2009, 52, 7631; CrossRef M. M. Alhamadsheh, N. C. Waters, D. P. Huddler, M. K. Deitrick, G. Florova, and K. A. Reynolds, Biorg. Med. Chem. Lett., 2007, 17, 879; CrossRef H. Chen, Y. H. Fan, A. Natarajan, Y. H. Guo, J. Iyasere, F. Harbinski, L. W. Christ, H. Aktas, and J. A. Halperin, Bioorg. Med. Chem. Lett., 2004, 14, 5401. CrossRef
7. B. Checa, E. Gálve, R. Parelló, M. Sau, P. Romea, F. Urpŕ, M. Font-Bardia, and X. Solans, Org. Lett., 2009, 11, 2193; CrossRef I. Larrosa, P. Romea, and F. Urpıĺ, Org. Lett., 2006, 8, 527; CrossRef Y. K. Wu, X. Shen, C. J. Tang, Z. L. Chen, Q. Hu, and W. J. Shi, Org. Chem., 2002, 67, 3802; CrossRef Y. Nagao, W. M. Dai, M. Ochiai, S. Tsukagoshi, and E. Fujita, J. Org. Chem., 1990, 55, 1148. CrossRef
8. R. W. Lamon, W. J. Humphlett, and W. P. Blum, J. Heterocycl. Chem., 1967, 4, 349; CrossRef J. J. D’Amico, F. G. Bollinger, J. J. Freeman, and W. E. Dahl, J. Heterocycl. Chem., 1986, 23, 105; CrossRef W. J. Humphlett and R. W. Lamon, J. Org. Chem., 1964, 29, 2146; CrossRef T. A. Foglia, L. M. Gregory, G. Maerker, and S. F. Osman, J. Org. Chem., 1971, 36, 1068. CrossRef
9. C. S. Dewey and R. A. Bafford, J. Org. Chem., 1965, 30, 491. CrossRef
10. See for instance: R. J. Maxwell, G. G. Moore, and L. S. Silbert, J. Org. Chem., 1977, 42, 1517. CrossRef
11. Y. K. Wu, Y. Q. Yang, and Q. Hu, J. Org. Chem., 2004, 69, 3990; CrossRef D. Delaunay, L. Toupet, and M. L. Corre, J. Org. Chem., 1995, 60, 6604; CrossRef M. Moreno-Mafias and I. Padros, J. Heterocycl. Chem., 1993, 30, 1235. CrossRef
12. A. Z. Halimehjani, H. Maleki, and M. R. Saidi, Tetrahedron Lett., 2009, 50, 2747; CrossRef Z. M. Ge, Q. Li, S. J. Zheng, T. M. Cheng, Y. X. Cui, and R. T. Li, Chin. J. Chem., 2006, 24, 381; CrossRef Z. Ge, J. Cui, Y. Wang, T. Cheng, and R. Li, Synthesis, 2004, 1257. CrossRef
13. A. Schall and O. Reiser, Eur. J. Org. Chem., 2008, 12, 31; H. M. R. Hoffman and J. Rabe, Angew. Chem., Int. Ed. Engl., 1985, 24, 94; CrossRef C. J. Cavallito and T. H. Haskell, J. Am. Chem. Soc., 1946, 68, 2332. CrossRef
14. A. Hassner, E. Ghera, T. Yechezkel, V. Kleiman, T. Balasubramanian, and D. Ostercamp, Pure Appl. Chem., 2000, 72, 1671; CrossRef S. Woodward, Chem. Soc. Rev., 2000, 29, 393; CrossRef M. P. Sibi and S. Manyem, Tetrahedron, 2000, 56, 8033; CrossRef F. Busqué, P. de March, M. Figueredo, J. Font, and L. González, Eur. J. Org. Chem., 2004, 1492. CrossRef
15. G. Z. Liu, H. W. Xu, G. W. Chen, P. Wang, Y. N. Wang, H. M. Liu, and D. Q. Yu, Bioorg. Med. Chem., 2010, 18, 1626; CrossRef H. W. Xu, J. F. Wang, G. Z. Liu, G. F. Hong, and H. M. Liu, Org. Biomol. Chem., 2007, 5, 1247; CrossRef H. W. Xu, G. F. Dai, G. Z. Liu, J. F. Wang, and H.-M. Liu, Bioorg. Med. Chem., 2007, 15, 4247. CrossRef
16. General procedure: CS2 (5 equiv) was added dropwise to the solution of amine (1 equiv) and Na3PO4·11H2O (0.6 equiv) in acetone. The reaction mixture was stirred at room temperature for 0.5 h. Then α, β-unsaturated ketones or esters (1 equiv) was added to the mixture, the reaction mixture was stirred at room temperature for 0.5 h (monitored by TLC). Upon completion, the reaction mixture was filtered and the filtrate concentrated under reduced pressure, extracted with CHCl3 (3×15 mL), dried over sodium sulfate, and concentrated under reduced pressure. The residue was purified by flash column chromatography on silica gel or recrystallized (in CHCl3/MeOH 1/1) to afford the product.
17. S. L. Cao, Y.-P. Feng, Y.-Y. Jiang, S.-Y. Liu, G.-Y. Ding, and R. T. Li, Bioorg. Med. Chem. Lett., 2005, 15, 1915. CrossRef
18. X. Y. Tang and M. Shi, Tetrahedron, 2009, 65, 9336; CrossRef P. Canonne, D. Bélanger, G. Lemay, and G. B. Foscolos, J. Org. Chem., 1981, 46, 3091; CrossRef C. Starkenmann, EP, 1342775, 2005.
19. CCDC 787505 contains the supplementary crystallographic data for this single crystal.
20. L. Jamir, A. R. Ali, H. Ghosh, F. A. S. Chipem, and B. K. Patel, Org. Biomol. Chem., 2010, 8, 1674; CrossRef T. K. Hansen, J. Becher, T. Jørgensen, K. S. Varma, R. Khedekar, and M. P. Cava, Org. Synth., 1998, 9, 203.
21. B. T. Chandler, J. T. Roland, Y.-K. Li, and E. Sorensen, Org. Lett., 2010, 12, 2746; CrossRef K. B. Wiberg, Angew. Chem., 1986, 98, 312. CrossRef
22. Materials: All Human cancer lines were kindly provided by Laboratory of Cancer Biology, the first affiliated hospital of Zhengzhou university. All cancer cells were grown in RPMI 1640 medium supplemented with 10% fetal bovine serum, 2 mm glutamine, 50 units/mL penicillin, and 50mg/mL streptomycin. Phosphate buffer saline (PBS) could be prepared by dissolving KH2PO4, Na2HPO4·12H2O, NaCl, KCl in distilled water.
Method: Exponentially growing cells were seeded into 96-well flat-bottomed plates at a concentration of 5×103 cells per well. After 24 h incubation at 37 °C, removed the culture medium and replaced with fresh medium containing the studied compounds in different concentrations to the wells, and the cells were incubated for another 72 h. Afterwards, 10µL of MTT solution (5mg/mL in phosphate buffered solution) was added to all wells and incubated for 4 h at 37 °C. During this period, formazon crystals would be formed at the bottom of each well. Pipetted out the spent media along with suspension of cultured cells and unconverted MTT, 150 μL of dimethyl sulfoxide (DMSO) was added to each well and shaked the plates to dissolve the dark blue crystals (formazan); their absorbance was measured using a spectrophotometric microplate reader at a wavelength of 490 nm. Each concentration was analyzed in triplicate and the experiment was repeated three times. The average 50% inhibitory concentration (IC50) was determined from the dose–response curves according to the inhibition ratio for each concentration.
In vitro antibacterial activity was studied with broth microdilution method. Staphylococcus aureus, Escherichia coli, Monilia albican and Aspergillus niger were chose for this study. The minimum inhibitory concentration (MIC) was measured. All the experiment was carried out in triplicate.