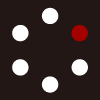
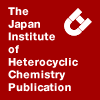
HETEROCYCLES
An International Journal for Reviews and Communications in Heterocyclic ChemistryWeb Edition ISSN: 1881-0942
Published online by The Japan Institute of Heterocyclic Chemistry
e-Journal
Full Text HTML
Received, 21st January, 2011, Accepted, 17th March, 2011, Published online, 1st April, 2011.
DOI: 10.3987/REV-11-693
■ N-Hydroxy- and N-Aminotetrazoles and Their Derivatives – Synthesis and Reactions
Dietrich Moderhack*
Institute of Pharmaceutical Chemistry, Technical University, Beethovenstrasse 55, D-38106 Braunschweig, Germany
Abstract
This account summarizes the preparative chemistry of the title compounds as it developed from the beginnings a century ago; though detailed, the report is intended to be illustrative rather than encyclopedic.INTRODUCTION
While tetrazole chemistry in all its branches is expanding with breath-taking speed,1 there is a growing demand for specialized reviews. Regarding surveys on functionalized representatives, the past decade has seen the publication of accounts on (i) 1,3- and 1,4-disubstituted tetrazolium salts,2 (ii) vinyltetrazoles,3 (iii) 2-substituted and 2,5-disubstituted tetrazoles,4 (iv) 1-substituted 5-alkyl(aryl)sulfanyltetrazoles and their derivatives,5 (v) Fe(II) complexes of 1-alkyltetrazoles,6 (vi) organometallic tetrazole derivatives,7 and (vii) metal derivatives of tetrazoles.8 Whereas the chemistry of N-hydroxytetrazoles has not been summarized at all, N-aminotetrazoles have been surveyed briefly twenty years ago when the entire family of N-aminoazoles was reviewed.9 The present concept, however, enables the author to provide more details. N-Attached O- or N-functionalities are found with three major categories of tetrazole rings (below).
A closer look shows that the left-hand class accommodates the widest variety of such groups [see overleaf Chart 1: Series (A), (C) and (B), (D)], whereas with the remaining ring types this diversity is reduced. Arrangement of material is as follows: After a joint retrospect of early findings the title systems are dealt with apart. Separation into sections on 'Synthesis' and 'Reactions' seemed only viable for the types shown in Chart 1/Part I. The first-named section describes those approaches that are generalized in Scheme 1, while transformations of the Y function (including most secondary processes) are found under 'Reactions.'
EARLY HISTORY
The first report on N-hydroxytetrazoles dates back to 1909 (Scheme 2). At that time the hydroximoyl derivatives (Ia) and (Ib, IV) were treated with sodium azide10 and nitrous acid,11 respectively, and the products isolated were thought to be III and VI. This view was maintained until the early 1960s, when IR spectroscopy revealed that the former materials were the open-chain azides (II) and (V) instead12,13 —members of a new class of compounds14–16 which proved surprisingly stable and, according to later findings, cyclize only under special conditions.17 A structure to be established, however, remains that of the product obtained by nitrosation (VIII) of the hydroxyamidrazone of polyacrylic acid (VII).18 The authors presented the material as the hydroxytetrazole (IX), but did not want to exclude that it existed in
ring-open form (VIII). Another early study (1910/13) was directed toward the reaction of fulminic acid with hydrogen azide.19 Two isomers were obtained and described as the hydroxytetrazole (XIa) and its N-oxide (XIb).19a,b While the structure of the first compound (XIa) appeared 'reasonable' inasmuch as Xa was the accepted constitution of fulminic acid in those days,20 the authors explaining XIb assumed the occurrence of a tautomerism between Xa and Xb19c — a possibility that had just been considered elsewhere.21 Judging from the melting points, the product (XIb) must be identical to the material that was to arise some time later from the nitrolic acid (XIII) and hydrogen azide22 and more recently from N-unsubstituted tetrazole and sodium perborate23a (see Chapter I/1); hence, its actual formula should be XIa. The nature of the lower melting substance, however, awaits clarification (XII ?24).
The class of N-aminotetrazoles was discovered in 1914 (Scheme 3): the benzylidene substituted hydrazonoyl azide (XV), prepared from XIV, cyclized25 on being heated to the derivative (XVI) which upon hydrolysis gave the representative (XVII).26a Next, the reaction of the aryl substituted hydrazidine (XVIII) with nitrous acid was studied, but the authors believed their product was the dihydropentazine (XIX).27 The prior workers,26a in view of the formation of XVI, rejected the pentazine structure immediately in favor of the constitution (XX)28 which was to be confirmed later.29 Moreover, another early approach to N-aminotetrazoles (XXI → XXII)30 clearly demonstrated that formation of a pentazine ring is disfavored.
I/1) N-OXYGEN FUNCTIONALITIES: SERIES (A), (A'), (B)
a) Synthesis
Treatment of tetrazole (1a) with sodium perborate in an acidic environment affords a 1 : 1.9 mixture of the hydroxytetrazoles (A1a) and (B1a) in 60% yield (Scheme 4) (cf. Scheme 1: Route 1). Separation of the isomers was effected via their benzyl ethers (→ Scheme 10) to eventually give 33% and 17% of pure A1a and B1a.23a As for B1a, an alternate access consists in oxygenation of the tetrazolide ion of 1b with oxone, which occurs quasi selectively at N(2) affording a ~ 1 : 70 mixture of A1g and B1b from which the latter component directly crystallized in 80% yield31a (cf. also ref.31b); hydrolysis (→ B1b'; 65%) followed by decarboxylation gave 40% of the target compound (B1a).31a, 32 While the overall yield of this sequence is comparable to that observed when starting from 1a, a major advantage of the procedure is the greater handiness which also allows multi-gram scale operations. As expected, the isomer (A1a) is readily available from the ester (A1g);31a the spontaneous decarboxylation of the product of hydrolysis (A1g') is typical of 1H-tetrazole-5-carboxylic acids.
Nitrolic acids such as (2a-c) react with hydrogen azide at ambient temperature to give the respective hydroxytetrazoles (A1a-c) in yields ranging from 50% (A1a)22 over 81% (A1c)33 to almost quantitative (A1b; Alk = Me, Et)22 (Scheme 5). The prior authors22 already recognized the reaction as proceeding via a nitrile oxide (cf. Scheme 1: Route 3a) and, in view of the results with fulminic acid (cf. Scheme 2),19b
described their products as N-oxides of type (G; NH in place of NR), believing that the N-hydroxy tautomers (A1) have formed in negligible amounts at best. A constitution (G), however, does not match established tetrazolium structures (cf. ref.34a). Indeed, a comparison of the energies of the tautomers (A1a), (Ea), (Fa), and (Ga ≈ XIb) calculated at the B3LYP/6-31G** level shows that the species in question is least favored (Chart 2);35a even N-oxides with a conventional substitution pattern, viz. Ea and Fa, are higher in energy than the N-hydroxy form [a similar relationship is also found with the family (B1a/Ha/Ja/Ka)].35b Nevertheless, N-oxides of that kind, in particular those of the F, H, and J series, might occur under conditions that favor the existence of azolium N-oxides in general.36,37
The most important entry to hydroxytetrazoles of class (A1) consists in cyclization of hydroximoyl azides (3) (Scheme 6; cf. Scheme 1: Route 2b). It was in the 1970s that this conversion could be accomplished for the first time.38a The authors found that the reaction of 3b (Ar = Ph) with acetyl or propionyl chloride in cyclohexane at ambient temperature directly yielded the hydroxytetrazole (A1d; Ar = Ph). In the case of 3c (Ar = Ph), propionyl and benzoyl chlorides were used, but here, instead of the respective product (A1e), the corresponding acyl derivatives (A3d; R'' = Et, Ph) were isolated; yet, heating of the propionyl compound in ethanol gave the free hydroxytetrazole very readily. Further examples of A1d,e (Ar = subst. Ph),38b A1f,38c and A3d (R'' = Me, CH2Cl) 38b were described in turn. These studies not only confirmed
the pronounced sensitivity of A3c (Alk = Me, Et) to hydrolysis38b (cf. above) and showed A3e to be labile as well;38c especially, they established that acid chlorides in the presence of base (as anticipated13) and also acetic anhydride lead to linear systems (like 4b,c). In acidic media, however, these compounds can be cyclized too (exemplified for 4b → A3da/A1ea).38b To explain this, the authors suggested that N(1) of the azido group is protonated whereby ring closure would be facilitated.38b However, more appropriate is a stereoelectronic approach, considering that ring closure requires a cis lone pair:39,40 It was found39a that treatment of the (Z)- or (E)-chloride (5a) with sodium azide in aqueous acetone at room temperature uniformly gave the (Z)-azide (4a). When this material was heated with excess acetyl chloride in benzene, the tetrazole (A3ca) was formed, i.e. the acid chloride, acting as a Lewis acid (!), acylates the imino nitrogen; this brings about 'C=N' bond rotation followed by dissociation of the acetyl group and cyclization of the (E)-isomer (4'). The same principle may underlie the ring closure of 3 since the acid chloride primarily causes Z/E inversion (→ 3') rather than acylation of the hydroxy group.39b Hence, contrasting with the sequence (3 → 4 → A3 → A1) put forward in ref.38b derivatives of type (A3) would constitute secondary products of A1. This view gains support by the observation that, on treatment of 3l with acetyl chloride, the bitetrazole (A1n) was formed prior to its acetyl derivative (A3j).41a
Expectedly, the preceding method for cyclization of 3 with an acid chloride38 found wide application by other workers. Such ring closures (mostly effected with acetyl chloride) include: 3a → A1b [Alk = i-Pr, (α-subst.) CH2Ph, cyclohex(en)yl];42a 3b → A1d [Ar = (subst.) Ph],42–44 3b → A1d (Ar = naphthyl),42a 3d → A1f,38c 3e → A1g,45 3g → A1i,42a 3h → A1j,42a and 3i → A1k.46 Only in the latter case the corresponding acyl derivatives (A3i: Alk = Me, Et) have been isolated and, in analogy to the questionable theory of ref.,38b have been viewed as arising from acylated 3i.46
A preparative alternative of more recent date consists in treating 3 with gaseous hydrogen chloride. Pertinent examples include conversions such as 3b → A1d [Ar = (subst.) Ph],41a 3f → A1h,47 3j → A1l,41b,48,49 3k → A1m,50 3l → A1n,41a and 3m → A1o51 (Scheme 7; note the suppression of the Boulton-Katritzky rearrangement which would lead to 6). Moreover, even acid impurities can be sufficient to induce ring closure [as observed for 3a → A1b (Alk = Bu)].41a As regards the general success of both cyclization methods (3 → A1), in most cases reasonable to excellent yields were observed. Finally, an entry of a different kind constitutes the ring transformation (7 → A1p) (cf. Scheme 1: Route 4a). The process is viewed as proceeding through a heteropentalene species which, remarkably, ring-opens between the positions 5 and 6.52
Entries to the series (A2) and (A'2) by ring closure have been verified as follows (Scheme 8): (i; cf. Scheme 1: Route 2b) The azide (8a), obtained from 3ca, cyclized to A2ba on treatment with acetyl chloride.38b The conversion occurred ca. 8 times more slowly than the cyclization of 3ca; this is due to the electron-donating ability of the methoxy group which slows down Z/E inversion of 8a (cf. also later Scheme 19). Similarly, 8b (obtained from 5b) could be transformed into A2aa, whereas trifluoroacetic acid mainly led to the corresponding urea.53 Yet, attempts at ring-closing 8c (made from 5c) failed; on treatment with electrophilic reagents there occurred only a change in geometry and the new (more stable) isomer reverted to the original form when irradiated with UV light.54 (ii; cf. Route 3c) Applying the concept for making 1-substituted 5-aminotetrazoles from cyanogen azide (9a) and primary amines,55a methoxyamine was reacted with 9a (generated in situ) to yield compound (A2d; 70%); while the linear intermediate has been presented in the Z-form,55a the direct precursor should be E. (iii; cf. Route 2a) Treatment of the geminal diazide (10a) with benzyloxyamine gave the tetrazole (A'2a; 67%), its dihydro structure is a corollary of the two acceptor groups at C(α); the SN product (11) was not isolated, it underwent a 1,3-H shift followed by cyclization.56a Monosubstituted hydrazines are capable of converting 10a in the same manner (see Chapter II/1).56a
b) Reactions
Reduction: Treatment of the hydroxytetrazoles (A1q) and (B1c) with titanium tetrachloride/lithium aluminium hydride led to the N-unsubstituted tetrazole (1c), i.e. the anti-asthma agent pranlukast (Scheme 9); the derivative (A1q) turned out to react more easily.31b Reductive removal of the methoxy group from A2aa57a and A2g58 leading to 1ea and 1da, respectively, has been effected with hydroiodic acid.
Alkylation: O-Substituted derivatives of type (A2) have been prepared in great number by treatment of hydroxytetrazoles (A1) with alk(en)yl halides in the presence of base (Scheme 10). Also silver salts of A1 have been reacted [as shown for A2aa,58 A2g,58 A2h,33 A2j,59 and A2q (Alk = Me, i-Pr)45]. Contrasting with this plethora, examples of the isomeric series (→ B2a23a) seem to be rare.60 The reverse reaction, i.e. dealkylation, has been performed with A2e/B2a,23a A2j,59 and A2l,42b showing that certain R' ligands can be used as protective groups. An unexpected process was encountered when A1da and A1ea were heated with a large excess of ethyl bromoacetate in methanol: Instead of the 'regular' products (A2m) and (A2na) the 2H-tetrazoles (12aa) and (12ba) were obtained. It was thought that A2m,na underwent deprotonation followed by N–O bond cleavage to give the anions of 1ea and 1fa which were in turn alkylated at N(2). Likewise, the intermediate (A2o) [formed from A1ea and phenacyl bromide (1 eq.) in hot ethanol] lost its side chain.57b
Further O-alk(en)yl derivatives (A2) were prepared via Michael type additions (Scheme 11).61 Thus, the 1-hydroxytetrazoles (A1ea,b) reacted with methyl vinyl ketone to readily give A2sa,b. Extending this study to dimethyl butynedioate (DMAD), educts like A1da and A1eb not only led to the anticipated Michael compounds (A2t,u) but, surprisingly, also to the N-unsubstituted and 2-substituted tetrazoles (1ea,fb) and (12a,bb), respectively. The formation of these by-products was rationalized assuming a complex sequence of addition/elimination steps; separate experiments revealed that when A2t,u were refluxed in methanol/ triethylamine the formation of those materials occurred too, whereas heating A2t in the presence of 1fa
not only produced the mixture of 1ea and 12a but also gave rise to 12ba.61
Acylation: An early, rather isolated example constitutes the reaction of the sodium salt of A1a with benzoyl chloride to give the compound (A3a) (Scheme 12).19b However, the product was not described as such, but viewed as an N-benzoyl derivative, as the authors believed the substrate was the N-oxide
(XIb) (cf. Scheme 2). After the discovery38a how the hydroximoyl azides (3) could be cyclized the number of O-acyl derivatives of A1 increased: With acid chlorides compounds like A3d [Ar = (subst.) Ph; Alk = Me, CH2Cl, Et],38a,b A3da,38b,57b A3db,38a,b,57b and A3dc57b were made; usage of acetic anhydride led to derivatives such as A3c [Ar = (subst.) Ph]41a and A3k41b including A3da.57b Isocyanates smoothly gave carbamoyl compounds such as A4a,b,57b while sulfonyl derivatives like A5a,b were readily prepared from the sodium salt of A1ea.57b — In conjunction with the processes leading to A3 and A5 mention should be made of the efficacy of A1a/B1a23b and A1d (Ar = Ph, 3-NO2C6H4)43 as acylation catalysts in peptide and oligonucleotide synthesis, respectively.
I/2) N-OXYGEN FUNCTIONALITIES: SERIES (E) – (K')
Synthesis and Reactions
The discovery of tetrazolium N-oxides is associated with alkylation reactions of A1. Since the substrates, especially their anions,62a exhibit ambident character, alkylation can affect the ring atoms (Scheme 13). This was first observed when preparing the O-alkyl derivatives (A2aa) and (A2ab): While these materials were obtained in 76 and 89% yield, isomeric N-oxides like Fca and Fcb were isolated as side products (1 and 4%, respectively); their structures were inferred from deoxygenation of Fca to 2-methyl-5-phenyl-tetrazole (13c).57a The N-oxides (Fca,b) also arose (53 and 30% yield) when A2aa,b were briefly heated to 190–210°C (see later). A study especially concerned with methylation of the hydroxytetrazoles (A1ba) and (A1da) disclosed more details:58 The authors observed that N-methylation was insignificant when the silver or triethylammonium salts of A1ba and A1da were treated with methyl iodide or dimethyl sulfate, but action of diazomethane on the free hydroxytetrazoles led to a methylation ratio of N : O = 1 : 5–6.62b A substantial increase in N-methylation resulted on employment of trimethyloxonium tetrafluoroborate: Treatment of the ammonium salt of A1ba with this reagent caused 41% ring methylation (two N-oxides observed) and gave only 57% A2g. Based on 1H NMR and MS data including reduction to 1,5-dimethyltetrazole (14b), the N-oxides were viewed as Eb (27%) and 15a (14%).58 However, a structure like 15 is untenable on energetic grounds; possibly migration of oxygen had occurred leading to Kb (or Hb which is still lower in energy; cf. Chart 3).63
Passing to the reaction of A1da (ammonium salt) with trimethyloxonium tetrafluoroborate,58 the procedure, apart from giving 34% A2aa, afforded 42% of a mixture containing the phenyl analogue (Ec) [or (15b) (no decision)] and a new type viewed as Gc; the presence of these components was deduced from deoxy-genation giving a 2 : 1 mixture of 13c and 14c. The reaction of free A1da, however, yielded a total of 85% of N- and O-methyl derivatives (10 : 1 mixture) from which the alleged oxide (Gc) could be isolated.58 While the authors recognized this material as identical to the previously made compound (Fca),57a they
adhered to their structure for spectroscopic reasons58 – a view that, expectedly, proved to be erroneous soon (see below).
Concomitant O- and N-alkylation also occurred on reacting 1-hydroxy-5-phenyltetrazole (A1da) with oxirane; here a 2 : 1 mixture of A2j and an unspecified N-oxide was obtained. The same O : N proportion
was observed using methyloxirane, but the products were doubled in each class, with the derivatives where R1 = H/R2 = Me (e.g. A2va) predominating.59
Contrasting with the foregoing (and also with the findings outlined in Scheme 11), Michael addition of 1-hydroxy-5-methyltetrazole (A1ba) led exclusively to N-oxides like Ed and Fd (ratio 3 : 1). While treatment with phosphorus trichloride afforded the respective deoxygenation products (13d) and (14d), alkali hydroxide caused retro addition to yield the starting tetrazole (A1ba).64
An efficient entry to N-oxides of type (F) constitutes brief heating of O-substituted derivatives (A2)57a,c (Scheme 14). The rearrangement has been shown to proceed intermolecularly; and, regarding the above controversy (Gc vs. Fca),58 an X-ray analysis confirmed Fca to be correct.57c,65 This finding is also in line with the calculated energies of the N-oxides 'derived' from A2aa (Chart 3).63 The wide scope of the reaction has found a preparative complement since, with certain substituents, also trifluoroacetic acid is capable of inducing this rearrangement (e.g. A2l → Fg).42b
Authentic examples of type (H) have recently become available by direct functionalization: treatment of the 1,5-disubstituted tetrazoles (16a-d,f) with the hypofluorous acid ⋅ acetonitrile complex gave the derivatives (Hd-h) in high yields; also a representative of type (J), viz. Jd, could be obtained in this manner.66 Compounds of type (K) are unknown, except for a dihydro congener like K'a; this material arose in low yield by oxidative ring contraction of 17 but withstood attempts of deoxygenation to give the known 1,4-dimethyltetrazolinone (18).67
II/1) N-NITROGEN FUNCTIONALITIES: SERIES (C), (C'), (D)
a) Synthesis
Direct amination of N-unsubstituted tetrazoles (1) has been accomplished with hydroxylamine-O-sulfonic acid in the presence of an inorganic base (Scheme 15) (cf. Scheme 1: Route 1). The procedure leads to a mixture of 1- and 2-aminotetrazoles (C1/D1) with C1 predominating (except for C1ca).34a,68,69 As the reagent is prone to hydrolysis the overall yields of the products are relatively low. Hence, working in an aprotic medium with a phase-transfer catalyst might constitute a general improvement (as exemplified for the preparation of C1e and D1e70).
Very important are cyclization reactions, although they only provide an entry to series (C). Nevertheless, there is a wide variety of routes and here, not unlike the methodology for 1-hydroxytetrazoles, cyclization of hydrazonoyl azides and their derivatives reigns supreme. Compounds of type (C2) have been prepared as detailed below (Scheme 16) (cf. Scheme 1: Route 2b): (i) C2a [Ar = (subst.) Ph]29,71a and C2c72 were obtained directly by treatment of the respective hydrazidines (19) with nitrous acid, the intermediary azides (20) being unisolable;71b (ii) also directly accessible were C2d (Ar = 2-Cl/MeOC6H4)73 and C2f,74
viz. by action of sodium (or hydrogen) azide on 19e and 19g; (iii) contrasting with the foregoing, azides prepared from 19b,d [Ar = (subst.) Ph]72 and 19f [Ar = (subst.) Ph]75a have been isolated and, in analogy to the process (3 → A1) (cf. Scheme 6), could be cyclized with acid chlorides giving C2b,c [yields higher than via nitrosation of 19 (X = NHNH2)]72 and C2e;75a the applied reagents might act as a Lewis acid attacking the hydrazinic N(1) of 20 [rather than N(2) as shown in ref.72]. Interestingly, the reaction (20e → C2e) will terminate in a modified product when certain azides of this kind were cyclized under Vilsmeier conditions:75b,c While representatives with Ar = Ph (or an electronegatively substituted phenyl group) gave the 'regular' tetrazoles (C2e), those bearing electron-donating groups yielded the N-formyl derivatives (C3j). Since C2e turned out to be inert toward Vilsmeier reagent (as exemplified with Alk = Me/Ar = 2-MeC6H4), formylation was considered to precede cyclization. This assumption could be confirmed by a model study using 19f (Ar = 2-MeC6H4) which was converted into the respective tetrazole (C3j) via 21 and 22. It should be noted that 22 eluded isolation when C3j was prepared from 20e.
Applying the principle that above gave A'2a (cf. Scheme 8), the reaction of the geminal diazides (10a,b) with appropriate hydrazine derivatives afforded dihydrotetrazoles like C'2a and C'3a,b in ca. 80% yield (Scheme 17) (cf. Scheme 1: Route 2a). Isolation of the SN products (23) was not possible in all cases.56a,b
Cyclization to N-sulfonamidotetrazoles like C7a,b was observed when the respective hydrazonoyl azides (25a,b) [Ar = (subst.) Ph] were refluxed in benzene (Scheme 18) (cf. Scheme 1: Route 2b). The yields, however, are low, since loss of molecular nitrogen from 25 competes with the ring closure so as to give rise to sulfonylsemicarbazides and nitriles as major products.76 When starting from 24c, an azide was formed that spontaneously cyclized even at room temperature to afford the tetrazole (C7c) in 63% yield.77
Contrasting with hydrazonoyl azides (20), congeners having a second C=N substructure like 27 are prone to ring closure on being heated (Scheme 19) (cf. Scheme 1: Route 2b). The earliest example constitutes the reaction (27a → C14a ≈ XV → XVI; cf. Scheme 3) which in hot ethanol proceeds within 10–20 min.26a
Hence, the tetrazole (C14a) was obtained directly when the chloride (26b; X = Cl) was treated for some hours with the reagent (ii) in refluxing ethanol (or methanol)26b or with (iii) in hot acetonitrile;78 however, in the first case the process could be held at the azide stage when heating was limited to one hour.26c On application of relatively mild conditions also azides such as 27b (Ar = 3-/4-NO2C6H4)26c and 27c-g79a,b have been isolated and cyclized separately (see below), whereas C14a [from 26b (X = NO2)],80 C14b (Ar = 4-MeOC6H4) [from 26c (X = NO2)],80 C14h,81 C14ia,82 and C14ja78 were made in one step, either at elevated or even ambient temperature (as applies to the latter two products). It should be mentioned that it was via C14ia that the parent (C1a) had been obtained for the first time (see below Scheme 29).82 Later, C14ia was prepared by a convenient one-pot procedure using benzaldehyde hydrazone, ethyl orthoformate, and sodium azide.83 Kinetic studies,79 performed with 27c-g in toluene at 70 °C, have disclosed that the (Z)-azides (which resulted from synthesis) underwent – in a rate determining step –
nitrogen inversion to give 27' which cyclizes immediately. This was deduced from the observation that the process not only showed a low sensitivity to solvents but also a characteristic dependence on substituents: ligands at the remote phenyl group had a more pronounced effect on the rate.
Derivatives of type (C15) having an imidoyl cyanide group as in C15a,b were obtained on gentle heating of the respective chlorides (28a,b) with sodium azide in DMF (Scheme 20) (cf. Scheme 1: Route 2b).84 Although the presence of the open-chain isomers (28a,b; N3 for Cl) was not excluded by IR spectroscopy, the authors were sure to view their products as tetrazoles since the materials were colorless and treatment with alkali hydroxide and acid gave the benzamido derivatives (C3f) and (C3ba), respectively; C3f was then hydrolyzed to the free aminotetrazole (C1ca) using hydrobromic acid. The related imidoyl chloride (C15c) was made and identified analogously.85a It gained importance as an intermediate for constructing the bitetrazole (29), after attempts to approach this compound via 28d had met with failure.26b,85a While one imidoyl azide 'subfunction' of 28d cyclized smoothly (→ C15d) (cf. 28c → C15c), the other group lost molecular nitrogen to form a carbodiimide (C16a) which was intercepted by azide ion (→ C2g)26b,85a or ethanol (→ C17a).26b As this degradation also occurred when C15c was used as precursor,85a the latter had to be converted into the amidrazone (C15e)85b which on treatment with nitrous acid gave the target compound (29) very readily. Thermolysis of this material caused ring opening of one of the tetrazole nuclei giving rise to C16a (trapped as C17a; cf. above), whereas hydrogenation led to the amidine (C15g).85a
A classical entry to 1-aminotetrazoles (C1) and derivatives (C14) bearing a nitrogen function also at C(5) consists in joint action of lead(II) oxide and sodium azide on thiosemicarbazides (30) and -carbazones (33), respectively (Scheme 21) (cf. also 'Early History:' XXI → XXII).30 The reaction was viewed as proceeding via the carbodiimide stage (cf. Scheme 1: Route 3e; here shown for 30 → C1) and was advantageously carried out in a CO2 atmosphere. However, the procedure did not work with 33a since benzylidene semicarbazide was formed rather than the tetrazole (C14ka); only lead(II) azide proved successful (low yield). An attempt to follow this route also for the synthesis of C3k failed since the substrate (31) was converted into the 1,3,4-oxadiazole (32a).30 Interestingly, with thiosemicarbazones such as 33b cyclization of the intermediary amidrazono azide not only affected N(2) but also N(4) to give, besides C14l, traces of the isomers (34).86 This competing course is a consequence of the electron- donating influence of the methyl group at that nitrogen (see also later Scheme 32: Dimroth rearrangement).
Regarding the above synthesis of diaminotetrazole (C1e) from 30a, improvements have been made: (i) by replacing carbon dioxide with ammonium chloride and by using dimethylformamide instead of ethanol (yield 59%),87 (ii) by replacing sodium azide – ammonium chloride with trimethylsilyl azide (79%)88a,b (see ref.88a also for an X-ray analysis of C1e). Avoiding lead containing reagents, C1e has been prepared: in 58% yield from diaminoguanidinium chloride (35a; X = Cl) via 35b (cf. Scheme 1: Route 2b)89a–c or
– applying the principle that above gave A2d (Scheme 8)55a – from cyanogen azide (9a) and hydrazine in 79% yield (Scheme 22) (cf. Scheme 1: Route 3c).55b
4,5-Dihydro-1H-tetrazoles like C'1a, C'2b, C'4a, and C'14a-c having a thioxo group at C(5) are readily available by reacting appropriate dithiocarbazates (36) and (37) with an azide salt in ethanol (Scheme 21) (cf. Scheme 1: Route 2a).90a–c Studies in this field aimed at providing special ligands for a range of β-lactam antibiotics.91
An approach that recalls the historical process (Xa → XIa) (Scheme 2) consists in adding hydrogen azide onto the amide of isofulminic acid (38a) to give the parent (C1a) in low yield (Scheme 22) (cf. Scheme 1: Route 3b).92 Alkyl derivatives such as C2h (Alk = Et, i-Pr, Bu) and C2i are available in like manner (yields 54–79 and 24%, respectively).93 Substrates having a cyano function lead to the class of diaminotetrazoles (cf. Scheme 1: Routes 3c,d). Thus, hydrogen azide adds smoothly to cyanohydrazones like 9b (R in Het = 1-Alk, 1-Ph; 2-Alk) to afford the respective derivatives (C14p) in high yield.94 As shown recently, this entry can be performed 'inversely,' i.e. by reacting cyanogen azide (9a) with a hydrazine component. Depending on its substituent (R) and (X), respectively, not only C1e (as mentioned above) but also derivatives of the series (C2), (C3), (C5), and (C6) have been obtained in reasonable yield.55b
As a different route to diaminotetrazoles the Dimroth rearrangement has been followed (Scheme 23) (cf. Scheme 1: Route 4b). When the 5-sulfonyltetrazole (39a) was refluxed with hydrazine hydrate for 2–3 min the 5-hydrazinotetrazole (40a) could be isolated; however, heating the latter in boiling xylene, morpholine or benzylamine gave the rearranged tetrazole (C1ga) very rapidly.95 Accordingly, prolonged treatment of the 5-chlorotetrazoles (39b,c) with hydrazine hydrate in boiling ethanol led directly to the derivatives (C1ga) and (C1gb).96 Conceivably, this applies also to an experiment in which 39b was refluxed for 24 hours with hydrazine hydrate: the product, contrary to the authors' belief of having prepared 40a,97 proved to be pure C1ga.98
b) Reactions
A theoretical study (INDO level) on the structure of all parent N-aminoazoles accompanied by selected experimental work has established that the amino group is sp3 configurated and prefers a configuration that avoids (or minimizes) lone pair–lone pair repulsions99a (cf. also ref.100). With monocycles, the most stable configuration shows the lone pair in the ring plane ('parallel' orientation) and the hydrogens on opposite sides. Thus, one α-nitrogen implies ap conformation, but with two α-nitrogens the 'perpendicular' orientation becomes important.99a As for the tetrazoles (C1a) and (D1a), this has in part been confirmed at the MP2/6-31G* level.101a In pursuit of this, B3LYP/6-31** calculations were carried out on C1a/D1a including C1e/D1e (Figure 1).101b For C1a they showed that the ap conformation is favored over sp99b by 4.30 kcal; in the case of D1a, 'parallel' (sp) and 'perpendicular' (ac) conformers were found that are almost identical in energy, the geometry of the latter corresponds to that reported in ref.101a Regarding C1e/D1e, the spatial arrangement of the N-amino groups parallels that of C1a/D1a, while the C-amino groups are conjugated with the π-system. For C1e (ap conformer) this difference was earlier demonstrated by an X-ray analysis.88a As a consequence the N-attached amino group is more nucleophilic; quite a number of reactions of C1e described below will illustrate this.
Alkylation: Unlike alkylations of the N-hydroxy function, corresponding reactions at the amino counterpart are rare. When C1e was treated with 1 eq. of tert-butyl alcohol in perchloric acid, work-up at pH 8–9 gave the derivative (C2m) in 28% yield (Scheme 24).102 The stoichiometry must be obeyed rigorously since 1.5–2 eq. of the reagent affected also the ring (→ F2a; 25–30%) and 3.5 eq. alkylated the C-amino group in addition (→ F2b; 68%). The thioxo substrate (C'1a) likewise underwent threefold alkylation (→ F2c; 75%).102 Moreover, in conjunction with the development of new energetic materials (cf. also Chapter II / 2), compounds like C2n and C2o have been prepared.103 Because of the electron-
withdrawing influence exerted by the tetrazole ring (cf. ref.104) the C–C bond of the side chain is stabilized; as observed above,102 the N-amino group of C1e reacted more readily.
Acylation: First experiments in the field pertain to C1ca,b (Scheme 25): the materials were heated with excess acetic anhydride which, because of the forcing conditions, caused exhaustive acetylation (→ C8b,c); a mono derivative (C3g) was detected as side product.26c Preparation of C8b was duplicated later34b,68 and analogues like C8a34b and C8d68,87 were made too. The structure of the latter – at one time deduced from the known failure of 5-aminotetrazoles to afford diacetylamino derivatives under these conditions68 – was recently confirmed by an X-ray analysis.105a The formation of compound (C8d) like that of C3h105b illustrates the enhanced reactivity of the 1-amino group over the 5-amino group. Interestingly, the 5-unsubstituted educt (C1a) failed to yield a compound of type (C8; R = H), nor did products of monoacylaion (C3) occur: reactions with several carboxylic acid chlorides and anhydrides gave, even under mild conditions, oxadiazoles (32b-cb) rather than C3a-bb.82b The surprising result was rationalized assuming a tetrazolium intermediate (O3f);82b this species having an extremely labile hydrogen at C(5) underwent proton loss and release of molecular nitrogen.106 Regarding the spectacular acyl derivative (C3i) which belongs to a family of dimeric IAP inhibitors, this material has presumedly been made using an appropriate hydroxyproline synthon.107 Acylations of the amino group of the
dihydrotetrazole (C'1a) with various anhydrides proceeded smoothly too (→ C'3c,d).90a,b The same applies to carbamoylation and sulfonylation of substrates (C1): not only could products like C5c,d34b and C7e34c be prepared, in this case it was also possible to obtain 5-unsubstituted derivatives such as C5b and C7d.82b
As a consequence of the stronger electron-withdrawing influence exerted by the tetrazol-2-yl system104 aminotetrazoles (D1) are less nucleophilic. Thus, on submission of D1ba and D1ca to the conditions suitable for making C3c-f the substrates turned out to be fully inert.34b Only working with triethylamine, in analogy to the process (D1ca → D8c),69 caused the reaction to occur (Scheme 26). But since usage of just 1 eq. of the acylating agent resulted in twofold functionalization – to give inseparable mixtures of the target derivatives (D3a-d) and unwanted diamides (D8a,b) or isoimides (D18a,b)34d –, preparation of D3a-d had to be performed stepwise: exhaustive acylation of the amino group followed by partial hydrolysis.34b The same procedure was required for a sulfonamide (D9a → D7a).34c The ureas (D5a,b) were accessible directly, but also here employment of a base was compulsory.34b
Reactions of acyl derivatives (beyond those shown above) are detailed below (Scheme 27). Side chain transformations include: (i) reduction of the amide functions in C'3c,d to secondary amino groups (→ C'2c,d);90a,b (ii) methylation of the amide functions in C3c,d and D3a,b as well as of the ureido groups in C5c and D5a to yield the derivatives (C3n,o), (C5e), (D3e,f), and (D5c), respectively;34b (iii) methylation of the sulfonamide functions in C7e and D7a to afford the derivatives (C7f) and (D7b).34c Ring opening
with evolution of nitrogen has been reported for the phthalimide derivative (D8c); the resultant nitrilimine was trapped as 41a,b and 42, while part of it decomposed into benzonitrile and phthalimidonitrene as evidenced by the adduct (43).69 It is worth noticing that, because of the electronegative substituent at N(2), cleavage of D8c occurs at much lower temperature than with 2,5-diaryltetrazoles. Also, comparing the behavior of 2-amino-5-phenyltetrazole (D1ca) and its 2-methyl congener (13c) in hot nitrobenzene (180–190 °C), the former compound has been found to ring open about 100 times faster.108
Nitrosation, Nitration: Typical of N-aminoazoles,9 compounds such as C1c (Ar = 2-Cl/4-NO2C6H4) underwent deamination (→ 1e) when treated with nitrous acid26c (Scheme 28); in the case of C1h, apparently the explosive azide (1i) arose.30 Accordingly, 1,5-diaminotetrazole (C1e) was defunctionalized to 1h (evidenced by the melting point) rather than cyclized to 44 as has been claimed.109 Treatment of the benzamide (C3f) with nitrosyl chloride also resulted in complete defunctionalization (→ 1ea).85a The recent technique of deaminating N-aminoheterocycles using a heteropoly acid (Preyssler's anion) has not been extended to tetrazoles (C1) or (D1).110 In conjunction with studies on the conformational mobility of certain (N-benzyl-N-nitrosoamino)azoles the derivatives (C11a) and (D11a) have been prepared (low yield; E/Z-isomerism not observable because of broadened signals).111a The novel reagent bismuth chloride–sodium nitrite, while in use for nitrosating sec. amines, amides, and even N-unsubstituted tetrazoles,111b has not been applied to the types (C2) and (D2).
Two isomeric (N-nitramino)tetrazoles (C12a) and (D12a) have been prepared by reacting the potassium salts of (C1a) and (D1a) with propyl nitrate. The products were converted into the ammonium and silver salts which were studied in detail as to their combustive behavior.112
Aldehydes, Ketones: Condensation of 1-aminotetrazoles (C1) with carbonyl compounds to give products of type (C14) constitutes the reaction most frequently performed, leaving a plethora of derivatives; also the tetrazolinethione (C'1a) has been transformed in that way (→ C'14) (Scheme 29). Often catalytic amounts of a strong acid have been employed.68,87,94,113,114 Regarding the behavior of C1e, the amino group at N(1) reacted in preference to that at C(5). This is evidenced by the derivatives (C14k,ka,p,w-y) including the hemiaminal (C13b); the latter resulted as the major product (60%) from 1-methyltetrazole-5-carbaldehyde.94, 119 Nevertheless, condensation reactions are possible at both amino groups: Apart from cyclic systems (see Scheme 33), this is shown by the derivatives (C14aa)116b and (C13a);87 also compound (C14bb) – remarkable for its unsymmetrical structure – belongs to this category.120 As listed in Scheme 29, acid hydrolysis of C14 (reaction [2]) has been reported for several examples; in addition, there exist some cases of hydrazinolysis. — Imines of type (D14) have been prepared too, albeit in relatively low number (Scheme 30).
Reactions occurring at the double bond of the aforementioned derivatives are shown in Scheme 31. Hydrogenation of C14a and D14f using sodium borohydride gave the (benzylamino)tetrazoles (C2p) and (D2a), respectively,111a in the same way the derivative (C'14b) was converted into C'2c.90a,b Treatment of the imines (C14u) and (D14h) with sodium borohydride or lithium aluminium hydride afforded the (propargylamino)tetrazoles (C2q) and (D2b), whereas action of Grignard reagent led to the derivatives (C2r) and (D2c).114 Joint action of 4-fluorobenzaldehyde and dibutyl phosphonate on diaminotetrazole (C1e) gave the aminophoshonate (C13c) (Kabachnik-Fields reaction).116a One-pot phosphonoalkylation of both amino groups (→ C13d) was possible in the presence of sulfuric acid; however, for higher yields the separately prepared bis-imine (C14aa) had to be submitted.116b
An important feature of compounds of type (C14) having a free amino group at C(5) constitutes their propensity to undergo the Dimroth rearrangement (Scheme 32): on being heated, the listed derivatives were converted into the hydrazones (34).94 Expectedly, electronegative groups at the imine carbon support this process as evidenced by comparing the half-lives of C14kc vs. C14kd and C14pb vs. C14pc. However, the reaction does not attain completion when the overall acceptor force of the imine function is too weak [as shown by the presence of C14ka,c (6%), C14y (9%), and C14kd (13%) in the final mixtures]. Also the parent amine (C1e) is capable of undergoing the Dimroth rearrangement: It has been found that heating of C1e with chalcones gave rise to tetrazolyl substituted dihydropyrazoles (46)121a,b [rather than to dihydrotetrazolotriazepines of type (45a) as was originally thought115].
Cyclization: As touched upon just before, substrates bearing an additional function at C(5) quasi invite to cyclization reactions. While the process (C1e → 45a), however, failed to materialize, a tetrazolotriazepine (45b) did arise in high yield when C1e was treated with acetylacetone (Scheme 33);87 also, a congener such as 45c could be prepared with ethyl acetoacetate.109 1,2-Dicarbonyl compounds led to the tetrazolotriazines (47a-d)120, 122 and (47e),109 whereas diethyl oxalate and pyruvic acid yielded the derivatives (49) and (50a), respectively.109 In line with this, the reaction of C1e with various 5-aryl-2,3-
dihydrofuran-2,3-diones produced the 7-aroyl substituted analogues (50b) [the intermediates (C3p) being unisolable].123 Yet, compounds like 50 (Q = R', SH), claimed as arising from C1e and carboxylic acids or carbon disulfide,109 should be regarded with doubt for two reasons: (i) In an attempt at duplicating the reaction (C1e → 48; Q = Me), i.e. by heating C1e in boiling acetic acid, the starting material was recovered unchanged;124 (ii) even though ring closures of that kind would occur, one should realize that fully conjugated azolotetrazoles having an NH ring group (any position) exist as monocyclic azidoazoles.125 This would also apply to the claimed109 tetrazolotetrazole (44) (cf. Scheme 28). — Cyclizations with C' are apparently unknown.
Miscellaneous: The amidine (C15h) and the ditriazolylamine (52) were obtained instead of the desired biazole (51) when the aminotetrazole (C1ba) reacted with "N,N-dimethylformamide azine" in the presence of an acid catalyst (Scheme 34). While C15h was viewed as arising from C1ba and dimethylformamide (supposedly formed by hydrolysis of the "azine" reagent), the pathway leading to 52
was not completely understood.126 Treatment of the aminotetrazoles (C1c,ca) with hypochlorous acid led to explosive (dichloroamino)tetrazoles (C10a,b); action of potassium iodide (or even storage) converted these materials into azo compounds (C19a,b).26c Another azo derivative (C19c) has been mentioned (but without characterization data) in conjunction with studies on the parent aminotetrazole (C1a);82b the authors also reported on the isocyanide (C20a) which was formed on treating C1a with chloroform and alkali.82a,b
Finally, degradation reactions of C1/D1 are briefly referred to: Action of lead tetraacetate on C1ca generated a tetrazolylnitrene which, in analogy to the cleavage of triazolylnitrenes,9 decomposed into molecular nitrogen and benzonitrile.78 Detailed studies, in part theoretical ones, exist on the thermolysis of C1a,127a C1ca/D1ca108 (cf. also 'Acylation'), C1e,127b–d and D1e.127a With C1a,e and D1e, two competing reaction modes giving complex mixtures were distinguished; the results of C1e were discussed in the light of an amino/imino tautomerism and compared to the thermal decomposition of certain other 5-aminotetrazoles.
II/2) N-NITROGEN FUNCTIONALITIES: SERIES (L) – (Q)
Synthesis and Reactions
In principle, aminotetrazolium salts (O1), (P1), and (Q1) are accessible by electrophilic amination as are the amines (C1) and (D1). But since the starting substrates, i.e. (5-substituted) 1-/2-alkyl(aryl)tetrazoles, are distinctly weaker nucleophiles than tetrazolide ions used for C1 and D1 (cf. Scheme 15), the process, apart from being extremely slow, does not attain completion. Thus, on treatment of 14a-c with O-mesitylsulfonylhydroxylamine the extent of conversion did not exceed 30, 66, and 36%,34a,c respectively, and with the less nucleophilic 2H-tetrazoles (13a-c) a mere 15, 43, and 13%34a were observed. From these six experiments could be secured only: (i) the salts (O1aa) and (O1ba) [the minor components (Q1) eluded isolation] and (ii) the salt (P1ba) (Scheme 35). Hence, representatives unavailable by amination had to be made by quaternization of the appropriate aminotetrazoles (C1)34a,89a,128 and (D1);34a,128a this is especially true of the salts (Q1) which from the latter were obtained very readily. The substrate (C1), because of its ambident character, gave mixtures of O1 and P1, but only in the case of C1ca the proportion allowed isolation of both O1cc and P1cc. Access to O1da89a and O2a106b by methylation of C1e and C2ha proceeded equally well; the latter salt, in line with the behavior of 1,4-disubstituted tetrazoliums having a free 5-position, decomposed immediately into molecular nitrogen and a carbodiimide on addition of base (analogous to the cleavage of O3f in Scheme 25). This degradation can also be caused by anion exchange resin: thus, the liquid salt (O1aa) which was formed from 14a as the predominant isomer (80%) could not be transformed into a crystalline iodide or bromide derivative (X = I / Br in place of MeOSO3).34a
Aminotetrazoliums of class (O1) and (Q1) having NH for NR should arise on treatment of C1 and D1 with strong acids, i.e. according to the observation that, under these conditions, N-amino- and N-methylazoles gave the same type of cation.99c,d While experimental evidence for the process [D1 → Q1 (NH for NR)] is still lacking, the respective behavior of C1 is well documented (Scheme 36): Diaminotetrazole (C1e) has been converted into a variety of salts such as: (i) nitrate (O1ea),89a (ii) perchlorate (O1eb),89a,129 (iii) picrate (O1ec),130 (iv) trinitrophloroglucinolate (O1ed),131 (v) ethylene- or propylene-bis[(5-nitroimino)-4,5-dihydro-1H-tetrazolide] (O1ee),132 and, finally, (vi) 5-nitrotetrazolide,133 2,4,5-trinitroimidazolide,133b and 3,5-dinitro-1,2,4-triazolide (O1ef).133b X-Ray analyses were performed with O1ea,89a O1eb,129 O1ec,130a O1ed,131 and O1ef (Z = 5-nitrotetrazolide),133b uniformly showing that protonation had occurred at N(4). This is consistent with computational studies which, in addition, confirmed that the amino groups of C1e are least favored for proton uptake.88b,129,130a Hence, the (5-aminotetrazol-1-yl)ammonium ion featured in ref.134 is a misleading description. Nevertheless, when reacting with metal ions, the 1-amino group may be engaged too; a prominent example is the derivative (53) whose structure has been verified by X-ray diffraction.88b
Compounds like O1bd,e,db-h, O1eg-i, and Q1be,f resulted in good to excellent yields from metathetical reactions. Thus, the tetrazolium iodide (O1bc) served to provide the corresponding nitrate (O1bd)89a,128 and perchlorate (O1be),128 while from the isomeric iodide (Q1bc) the respective salts (Q1be) and (Q1bf) were obtained.128a Diaminotetrazoliums were sought for in higher number: Starting from the perchlorate salt (O1eb), the dinitramide (O1ei) was made;135 using the iodide (O1da), salts like the nitrate (O1de),89a dinitramide (O1df),89a,136 azide (O1dg),89a and 5-aminotetrazolide (O1dh)137 were prepared. From the iodide (O1da) and the nitrate (O1ea) derivatives containing hexanitratolanthanate(III) and -cerate(III) ions arose (→ O1dc,d and O1eg,h, respectively).138 All of the ionic compounds of Scheme 36 were synthesized in search for new energetic materials;139 their physical properties underwent in-depth studies of great number (experimental140,141 and theoretical ones142). The attractive topic, a rapidly developing field, cannot be covered here adequately.
Betainic structures such as L, M, and N, i.e. tetrazolium N-aminides, have been obtained by deprotonation of the corresponding cations in O, P, and Q (Scheme 37).34a–c Direct introduction of the Y – function,
as shown with certain oxygen analogues (Chapter I/2), has not been described till now. For stabilization all aminides of the L and M type need electronegative substituents (Z) at the side chain; practically, they were inserted by joint treatment of the salts (O1) and (P1) with an acylating agent and a base. The same technique applied to Q1 gave the respective derivatives of the isomeric N series. But here, because of the enhanced electron-withdrawal of the 1H-tetrazolium-3-yl system (cf. ref.143a), also N-unsubstituted aminides proved sufficiently stable to be isolated (→ N1a,b).34b
Since salts like O3/P3/Q3 and O5/P5/Q5 are the immediate aminide precursors, an alternate access constitutes quaternization of C3/C5 and D3/D5 (Scheme 38). However, owing to the extremely low nucleophilicity of the respective 2H-tetrazoles (cf. Scheme 35: 13 → P1) the concept works with C3 and C5 only, and, in addition, is limited to 5-methyl derivatives. Competing side-chain methylation giving
C3n,o and C5e (cf. Scheme 27) can be avoided by strictly adhering to the reaction conditions.34b Reprotonation of L3, M3, and N3 took place readily with hydrobromic acid (O3d, P3d, and Q3d showed pka ≈ 3), but isolation of acetamide derivatives such as O3c and P3c (R' = Me for Ph) was vitiated because of hydrolysis.34b Methylation of L3, M3, and N3 occurred equally well to give O3d,e, P3d,e, and Q3e,f, respectively; in the case of N3 the enhanced electron-withdrawal of the tetrazolium ring required prolonged action of the reagent.34b Unlike tetrazolium N-phenacylides,143a,b aminides of the L and M series (i.e. even the more reactive ones) have been found to be inert toward phenyl isocyanate.34c,e
CONCLUSION
The present overview has traced the considerable progress in preparative chemistry of the title systems since they came into focus around 1910. Yet, while work on N-aminotetrazoles is well advanced, gaps show up with the N-hydroxy counterparts: 2H-isomers (B) have barely been studied till now; the old entry to A1a using fulminic acid has not been clarified in full, and certain ring alkylations of A1 are dubious. Future research should allow for this and, maybe, extend its interest to comparative investigations between 1H- and 2H-isomers (A / B) as studies of that kind exist only in the class of N-aminotetrazoles (C / D).
References
1. As evidenced by the survey covering the period 1995–2007: V. A. Ostrovskii, G. I. Koldobskii, and R. E. Trifonov, 'Comprehensive Heterocyclic Chemistry III: Tetrazoles,' Vol. 6, ed. by V. V. Zhdankin (Series ed. by A. R. Katritzky, R. J. K. Taylor, C. A. Ramsden, and E. Scriven), Elsevier, Amsterdam, 2008, pp. 257–423.
2. S. V. Voitekhovich, P. N. Gaponik, and O. A. Ivashkevich, Usp. Khim., 2002, 71, 819; Russ. Chem. Rev., 2002, 71, 721. CrossRef
3. V. N. Kizhnyaev and L. I. Vereshchagin, Usp. Khim., 2003, 72, 159; Russ. Chem. Rev., 2003, 72, 143. CrossRef
4. G. I. Koldobskii and R. B. Kharbash, Zh. Org. Khim., 2003, 39, 489; Russ. J. Org. Chem., 2003, 39, 453. CrossRef
5. G. I. Koldobskii, A. Hrabalek, and K. A. Esikov, Zh. Org. Khim., 2004, 40, 479; Russ. J. Org. Chem., 2004, 40, 447. CrossRef
6. J. Kusz, P. Gütlich, and H. Spiering, 'Topics in Current Chemistry: Structural Investigations of Tetrazole Complexes of Iron(II),' Vol. 234, ed. by P. Gütlich and H. A. Goodwin, Springer, Berlin, 2004, pp. 129–153.
7. S. V. Voitekhovich, P. N. Gaponik, and G. I. Koldobskii, Zh. Org. Khim., 2005, 41, 1599; Russ. J.
Org. Chem., 2005, 41, 1565. CrossRef
8. P. N. Gaponik, S. V. Voitekhovich, and O. A. Ivashkevich, Usp. Khim., 2006, 75, 569; Russ. Chem. Rev., 2006, 75, 507. CrossRef
9. V. V. Kuzmenko and A. F. Pozharskii, 'Advances in Heterocyclic Chemistry: N-Aminoazoles,' Vol. 53, ed. by A. R. Katritzky, Academic Press, London, 1992; pp. 85–231.
10. M. O. Forster, J. Chem. Soc., 1909, 95, 184; M. O. Forster, Proc. Chem. Soc., 1909, 25, 25.
11. H. Wieland, Ber. Dtsch. Chem. Ges., 1909, 42, 4199. CrossRef
12. F. Eloy, J. Org. Chem., 1961, 26, 952. CrossRef
13. Also the benzoyl derivative obtained from alleged III with benzoyl chloride in the presence of alkali hydroxide10 proved to be linear [rather than a compound of type (A3)]; the same should hold for the derivatives made in that way with benzenesulfonyl and naphthaline-2-sulfonyl chloride10 (cf. ref.38b).
14. M. S. Chang and A. J. Matuszko, J. Org. Chem., 1963, 28, 2260. CrossRef
15. a) T. Sasaki and T. Yoshioka, Yuki Gosei Kagaku Kyokaishi, 1967, 25, 665; b) T. Sasaki and T. Yoshioka, Bull. Chem. Soc. Jpn., 1967, 40, 2608. CrossRef
16. Also, addition of sodium azide onto nitrile oxides affords hydroximoyl azides, not hydroxytetrazoles: C. Grundmann and H.-D. Frommeld, J. Org. Chem., 1966, 31, 157. CrossRef
17. For cyclization of hydroximoyl azides to hydroxytetrazoles, see Chapter I / 1.
18. C. Fetscher and S. Lipowski, U. S. 3 350 374; Oct. 31, 1967 (Chem. Abstr., 1968, 68, 3569). — Curiously, in this document the azide ligand still occurs as three-membered ring.
19. a) F. C. Palazzo, Atti Accad. Naz. Lincei, Classe Sci. Fis. Mat. Nat., Rend., 1910, 19 (I), 218; b) F. C. Palazzo and G. Marogna, Gazz. Chim. Ital., 1913, 43 (I), 69; c) F. C. Palazzo, Atti Accad. Naz. Lincei, Classe Sci. Fis. Mat. Nat., Rend., 1912, 21 (II), 713; d) F. C. Palazzo and G. Marogna, Gazz. Chim. Ital., 1913, 43 (I), 563.
20. For the history of fulminic acid, cf.: a) F. Kurzer, J. Chem. Educ., 2000, 77, 851; CrossRef b) D. Moderhack, Pharmaz. Ztg., 1979, 124, 2493; Dtsch. Apotheker-Ztg., 1979, 119, 2066.
21. H. Ley and H. Kissel, Ber. Dtsch. Chem. Ges., 1899, 32, 1357. CrossRef
22. S. Maffei and G. Bettinetti, Ann. Chim. (Rome), 1956, 46, 812.
23. a) M. Begtrup and P. Vedsø, J. Chem. Soc., Perkin Trans. 1, 1995, 243; CrossRef b) J. C. Spetzler, M. Meldal, J. Felding, P. Vedsø, and M. Begtrup, J. Chem. Soc., Perkin Trans. 1, 1998, 1727. CrossRef
24. Compared to their cyclic isomers, hydroximoyl azides show lower melting points; cf. F. R. Benson, 'The High Nitrogen Compounds,' J. Wiley and Sons, New York, 1983, p. 222.
25. Note the difference from the behavior of compound (II).
26. a) R. Stollé and F. Helwerth, Ber. Dtsch. Chem. Ges., 1914, 47, 1132; CrossRef b) R. Stollé and A. Netz, Ber. Dtsch. Chem. Ges., 1922, 55, 1297 [in the case Ar = 4-MeOC6H4, the chloride (26c) gave a mixture of the respective azide and tetrazole; reaction was performed with reagent (ii) in refluxing methanol; CrossRef c) R. Stollé, A. Netz, O. Kramer, S. Rothschild, H. Erbe, and O. Schick, J. Prakt. Chem., 1933, 138, 1; CrossRef d) no experimental details in ref.26c.
27. F. D. Chattaway and G. D. Parkes, J. Chem. Soc., 1926, 113. CrossRef
28. R. Stollé, J. Prakt. Chem., 1926, 114, 348. CrossRef
29. J. M. Burgess and M. S. Gibson, Tetrahedron, 1962, 18, 1001. CrossRef
30. R. Stollé and E. Gaertner, J. Prakt. Chem., 1931, 132, 209. CrossRef
31. a) R. G. Giles, N. J. Lewis, P. W. Oxley and J. K. Quick, Tetrahedron Lett., 1999, 40, 6093 [there is an error concerning the yield of B1b': the graphic shows 65%, whereas the text quotes 81%, which apparently refers to the conversion (A1g → A1g' → A1a)]; CrossRef b) R. G. Giles, N. J. Lewis, P. W. Oxley and J. K. Quick, (Smithkline Beecham PCL, UK), PCT Int. Appl., 1998, WO 30559 (Chem. Abstr., 1998, 129, 122667).
32. For this concept, compare: D. Moderhack and A. Lembcke, Chem.-Ztg., 1984, 108, 188.
33. O. A. Luk'yanov and N. I. Shlykova, Izv. Akad. Nauk, Ser. Khim., 1992, 1619; Russ. Chem. Bull., 1992, 41, 1250.
34. a) D. Moderhack and M. Noreiks, Heterocycles, 2004, 63, 2605; CrossRef b) D. Moderhack and M. Noreiks, Heterocycles, 2009, 78, 2509; CrossRef c) M. Noreiks, 'Tetrazolium-N-aminide – Synthese und Eigenschaften (mit einem Beitrag zur Phenylcarbamoylierung von 1,2,4-Triazolium-Analoga),' Dissertation, Technical University of Braunschweig (Germany), 2006; d) as shown also by compound (D18c), the formation of an isoimide (instead of a diamide) seems to be typical of D1 when acylating it with an aroyl chloride (cf. ref.34c); e) D. Moderhack and M. Noreiks, Heterocycles, 2006, 68, 2113. CrossRef
35. a) Total energies (absolute minima) of A1a/Ea/Fa/Ga and B1a/Ha/Ja/Ka: –333.41225/.40280/
.40868/.40058 and –333.41863/.41268/.41230/.40450 a.u. (all values: D. Moderhack, unpublished results; b) for the lower energy of B1a compared to A1a (calculated at B3LYP/6-31G* level), see also: Z. X. Chen, J. M. Xiao, H. M. Xiao, and Y. N. Chiu, J. Phys. Chem. (A), 1999, 103, 8062; CrossRef c) the reverse energetic relation (B1a>A1a) obtained at MP2/6-31G* level35d could not be confirmed (D. Moderhack, unpublished result); d) Z. Chen and H. Xiao, Propell., Explos., Pyrotech., 1999, 24, 319.
36. For examples, see: a) J. Elguero, C. Marzin, A. R. Katritzky, and P. Linda, 'Advances in Heterocyclic Chemistry, Suppl. 1: The Tautomerism of Heterocycles,' ed. by A. R. Katritzky and A. J. Boulton, Academic Press, London, 1976; pp. 485–490; b) V. I. Minkin, A. D. Garnovskii, J. Elguero, A. R. Katritzky, and O. V. Denisko, 'Advances in Heterocyclic Chemistry: The Tautomerism of Heterocycles: Five-Membered Rings with Two or More Heteroatoms,' Vol. 76, ed. by A. R. Katritzky, Academic Press, London, 2000; pp. 216–225.
37. In the tetrazole series experimental studies of the N-hydroxy/N-oxide tautomerism are limited to an isolated attempt at detecting a species of type (F) (which could not be found),38b whereas the authors of ref.33 addressed the problem but refrained from investigating it.
38. a) J. Plenkiewicz, Tetrahedron Lett., 1975, 341; CrossRef b) J. Plenkiewicz, Tetrahedron, 1978, 34, 2961; CrossRef c) J. Plenkiewicz and E. Osuchowska, Pol. J. Chem., 1978, 52, 1597.
39. a) A. F. Hegarty and M. Mullane, J. Chem. Soc., Chem. Commun., 1984, 913; CrossRef b) Cf. K. J. Dignam, A. F. Hegarty, and P. L. Quain, J. Org. Chem., 1978, 43, 388.
40. G. Leroy, M.-T. Nguyen, M. Sana, and J.-L. Villaveces, Bull. Soc. Chim. Belg., 1980, 89, 1023. CrossRef
41. a) I. V. Tselinskii, S. F. Mel'nikova, and T. V. Romanova, Zh. Org. Khim., 2001, 37, 455; Russ. J. Org. Chem., 2001, 37, 430; CrossRef b) I. V. Tselinskii, S. F. Mel'nikova, and T. V. Romanova, Zh. Org. Khim., 2001, 37, 1708; Russ. J. Org. Chem., 2001, 37, 1638.
42. a) A. J. Liepa, R. H. Nearn, and D. A. Jones, PCT Int. Appl., WO 98 39309; Sept. 11, 1998 (Chem. Abstr., 1998, 129, 230730) [in this document the first author is misspelt 'Leipa']; b) A. J. Liepa, D. A. Jones, T. D. McCarthy, and R. H. Nearn, Aust. J. Chem., 2000, 53, 619; CrossRef c) A. J. Liepa, R. H. Nearn, and E. R. T. Tiekink, Z. Kristallogr. – New Cryst. Struct., 2001, 216, 538.
43. C. B. Reese and Z. Pei-Zhuo, J. Chem. Soc., Perkin Trans. 1, 1993, 2291. CrossRef
44. K. Rehse and F. Brehme, Arch. Pharm. (Weinheim), 2000, 333, 157. CrossRef
45. O. A. Luk'yanov and N. I. Shlykova, Izv. Akad. Nauk SSSR, Ser. Khim., 1987, 2540; Bull. Acad. Sci. USSR, Div. Chem. Sci., 1987, 36, 2358.
46. M.-H. Shih, M.-J. Lee, and M.-Y. Yeh, J. Chin. Chem. Soc., 2002, 49, 361.
47. V. N. Yarovenko, S. A. Kosarev, I. V. Zavarzin, and M. M. Krayushkin, Izv. Akad. Nauk, Ser. Khim., 2002, 1387; Russ. Chem. Bull., 2002, 51, 1504. CrossRef
48. V. G. Andrianov, V. G. Semenikhina, and A. V. Eremeev, Khim. Geterotsikl. Soedin., 1992, 687; Chem. Heterocycl. Compd., 1992, 28, 581. CrossRef
49. A. B. Sheremetev, V. G. Andrianov, E. V. Mantseva, E. V. Shatunova, N. S. Aleksandrova, I. L. Yudin, D. E. Dmitriev, B. B. Averkiev, and M. Yu. Antipin, Izv. Akad. Nauk, Ser. Khim., 2004, 569; Russ. Chem. Bull., 2004, 53, 596. CrossRef
50. V. N. Yarovenko, O. V. Lysenko, and M. M. Krayushkin, Khim. Geterotsikl. Soedin., 1993, 529; Chem. Heterocycl. Compd., 1993, 29, 452. CrossRef
51. V. G. Andrianov, V. G. Semenikhina, and A. V. Eremeev, Khim. Geterotsikl. Soedin., 1989, 1700; Chem. Heterocycl. Compd., 1989, 25, 1419; CrossRef cf. also V. G. Andrianov and A. V. Eremeev, Khim.
Geterotsikl. Soedin., 1990, 1443; Chem. Heterocycl. Compd. (USSR), 1990, 26, 1199.
52. A. M. Churakov, S. L. Ioffe, V. S. Kuz'min, Yu. A. Strelenko, Yu. T. Struchkov, and V. A. Tartakovskii, Khim. Geterotsikl. Soedin., 1988, 1666; Chem. Heterocycl. Compd. (USSR), 1988, 24, 1378. CrossRef
53. D. D. Dolliver, T. Sommerfeld, M. L. Lanier, J. A. Dinser, R. P. Rucker, R. J. Weber, and A. S. McKim, J. Phys. Org. Chem., 2010, 23, 227.
54. Yu. B. Salamonov and O. A. Luk'yanov, Izv. Akad. Nauk SSSR, Ser. Khim., 1983, 207; Bull. Acad. Sci. USSR, Div. Chem. Sci., 1983, 32, 181. CrossRef
55. a) Y.-H. Joo and J. M. Shreeve, Org. Lett., 2008, 10, 4665; CrossRef b) Y.-H. Joo, B. Twamley, S. Garg, and J. M. Shreeve, Angew. Chem., 2008, 120, 6332; CrossRef Angew. Chem. Int. Ed., 2008, 47, 6236; CrossRef — Cf., however, the reaction with sec. amines: Y.-H. Joo and J. M. Shreeve, Eur. J. Org. Chem., 2009, 3573. CrossRef
56. a) R. W. Saalfrank and U. Wirth, Chem. Ber., 1989, 122, 519; CrossRef b) the configuration of C'2a and C'3a,b (in ref.56a supposed to be E, here shown accordingly) might be Z: cf. T. A. Kuz'menko, V. V. Kuz'menko, A. F. Pozharskii, O. V. Kryshtalyuk, and G. G. Aleksandrov, Khim. Geterotsikl. Soedin., 1992, 205; Chem. Heterocycl. Compd., 1992, 28, 167. CrossRef
57. a) J. Plenkiewicz, Tetrahedron Lett., 1978, 399; CrossRef b) J. Plenkiewicz and E. Stefańska-Halladin, Bull. Acad. Pol. Sci., Sér. Sci. Chim., 1978, 26, 189; c) J. Plenkiewicz and A. Roszkiewicz, Pol. J. Chem., 1993, 67, 1767.
58. O. A. Luk'yanov and N. I. Shlykova, Izv. Akad. Nauk SSSR, Ser. Khim., 1984, 181; Bull. Acad. Sci. USSR, Div. Chem. Sci., 1984, 33, 161.
59. O. A. Luk'yanov, N. I. Shlykova, and Yu. A. Strelenko, Izv. Akad. Nauk SSSR, Ser. Khim., 1987, 2376; Bull. Acad. Sci. USSR, Div. Chem. Sci., 1987, 36, 2202.
60. Divergent from the view of Chemical Abstracts, the present author did not find unequivocal evidence for the occurrence of derivatives of type (B2) in: W. Zambach, A. Steiger, P. Renold, and R. G. Hall, PCT Int. Appl., 2004, WO 020445 A2 (Chem. Abstr., 2004, 140, 433512). — The alleged materials (B2) are thought to be tetrazoles having the RO group at C(5), in analogy to RS derivatives reported.
61. H. Dziklinska, S. Dzierzgowski, A. Jezewski, and J. Plenkiewicz, Bull. Soc. Chim. Belg., 1989, 98, 277. CrossRef
62. a) The acidic nature of N-hydroxytetrazoles was recognized by the first workers in the field;19b yet, pka values do not seem to have been reported to date; b) N-methylation was also observed earlier on treatment of the alleged N-oxide (XIb) (Scheme 2) with diazomethane.19b.
63. Total energies (absolute minima) of A2g/Eb/Fb/Gb/B2b/Hb/Jb/Kb and A2aa/Ec/Fca/Gc/15b/ B2c/Hc/Jc/Kc (absolute minima): –412.05050/.05163/.05839/.05283/.05518/. 05848/.06204/.05365 and –603.79274/.79289/.80160/.79739/.72888/.79921/.79869/.80560/.79489 a.u. — Calculations at HF/6-31G** level showed the methoxy derivatives (A2g) and (A2aa) to be lowest in energy, whereas the values of 15a and 15b were higher by 47.14 and 46.41 kcal/mol, respectively (all values: D. Moderhack, unpublished results).
64. O. A. Luk'yanov, N. I. Shlykova, and Yu. A. Strelenko, Izv. Akad. Nauk SSSR, Ser. Khim., 1989, 1619; Bull. Acad. Sci. USSR, Div. Chem. Sci., 1989, 41, 1484.
65. This X-ray analysis was to exclude that the product of deoxygenation (13b)57a originated from Gc; possibly the experiment was suggested by the findings of ref.58 (that paper not mentioned in ref.57c).
66. T. Harel and S. Rozen, J. Org. Chem., 2010, 75, 3141. — The authors do not mention the literature dealing with other types of tetrazolium N-oxides. CrossRef
67. D. Seebach, R. Dach, D. Enders, B. Renger, M. Jansen, and G. Brachtel, Helv. Chim. Acta, 1978, 61, 1622. CrossRef
68. R. Raap, Can. J. Chem., 1969, 47, 3677 — Preparation of C1e and D1e was mentioned just before, but experimental details were not disclosed; CrossRef H. B. Jonassen, T. Paukert, and R. A. Henry, Appl. Spectroscopy, 1967, 21, 89. CrossRef
69. T. L. Gilchrist, G. E. Gymer, and C. W. Rees, J. Chem. Soc., Perkin Trans. 1, 1975, 1747. CrossRef
70. A. L. Kovalenko, V. I. Krutikov, and I. V. Tselinskii, Zh. Org. Khim., 1991, 27, 882; Russ. J. Org. Chem., 1991, 27, 760.
71. a) A. F. Hegarty, J. B. Aylward, and F. L. Scott, J. Chem. Soc. (C), 1967, 2587 [the authors duplicated the findings of ref.,29 but were not fully convinced that the products are tetrazoles (in contrast to the view of ref.28)]; CrossRef b) azides like 20a, however, were isolable when made from the respective bromides (19a; X = Br in place of NHNH2) and azide ion, but, interestingly, could not be cyclized under a variety of conditions (inter alia, by treatment in hydrochloric acid with sodium nitrite);71a this failure might have entertained the authors' doubt toward C2a decisively.
72. J. Plenkiewicz, Pol. J. Chem., 1979, 53, 521.
73. V. N. Bodnar, M. O. Lozinskii, and P. S. Pel'kis, Ukr. Khim. Zh. (Russ. Ed.), 1982, 48, 1308 (Chem. Abstr., 1983, 98, 126041). – In the case of Ar = 2-NO2C6H4, the azide (20d) did not cyclize.
74. R. Raap, Can. J. Chem., 1968, 46, 2255. CrossRef
75. a) L. Bruché, L. Garanti, and G. Zecchi, Synth. Commun., 1992, 22, 309; CrossRef b) G. Zecchi, Synlett, 1992, 858; CrossRef c) L. Bruché, L. Garanti, and G. Zecchi, J. Chem. Res. (S), 1994, 318.
76. a) S. Ito, Y. Tanaka, and A. Kakehi, Chem. Lett., 1982, 59; CrossRef b) S. Ito, Y. Tanaka, and A. Kakehi, Bull. Chem. Soc. Jpn., 1984, 57, 539. CrossRef
77. K. A. Jensen, A. Holm, and S. Rachlin, Acta Chem. Scand., 1966, 20, 2795. CrossRef
78. K. Sakai and J.-P. Anselme, Org. Prep. Proced. Int., 1975, 7, 61. CrossRef
79. a) A. F. Hegarty, K. Brady, and M. Mullane, J. Chem. Soc., Chem. Commun., 1978, 871; CrossRef b) A. F. Hegarty, K. Brady, and M. Mullane, J. Chem. Soc., Perkin Trans. 2, 1980, 535. CrossRef
80. G. V. Nekrasova, T. N. Lyamina, É. S. Lipina, G. A. Berkova, T. Ya. Paperno, V. P. Pozdnyakov, and V. V. Perekalin, Zh. Org. Khim., 1992, 28, 1024; Russ. J. Org. Chem., 1992, 28, 794; a) M. Wagatsuma, S. Hatsuno, T. Yamaguchi, and S. Ohshima (Tanabe Seiyaku Co., Ltd., Japan), Eur. Pat. Appl., 1983, EP 81971 A2 (Chem. Abstr., 1983, 99, 122179).
81. J. Donovan, J. Cronin, F. L. Scott, and A. F. Hegarty, J. Chem. Soc., Perkin Trans. 2, 1972, 1050. CrossRef
82. a) I. Hagedorn, K. E. Lichtel, and H.-D. Winkelmann, Angew. Chem., 1965, 77, 726; CrossRef Angew. Chem. Int. Ed., 1965, 4, 702; b) I. Hagedorn and H.-D. Winkelmann, Chem. Ber., 1966, 99, 850. CrossRef
83. a) P. N. Gaponik and V. P. Karavai, SU Pat., 1982, 915423 (Chem. Abstr., 1983, 98, 34585); b) P. N. Gaponik and V. P. Karavai, Khim. Geterotsikl. Soedin., 1983, 841; Chem. Heterocycl. Compd. (USSR), 1983, 19, 681. CrossRef
84. R. Fusco and S. Rossi, Ann. Chim. (Rome), 1960, 50, 277.
85. a) H. Behringer and H. J. Fischer, Chem. Ber., 1962, 95, 2546; CrossRef b) on this occasion the related hydroxyamidine (C15f) has also been made.
86. R. N. Butler and F. L. Scott, J. Org. Chem., 1966, 31, 3182. CrossRef
87. P. N. Gaponik and V. P. Karavai, Khim. Geterotsikl. Soedin., 1984, 1683; Chem. Heterocycl. Compd. (USSR), 1984, 20, 1388. — Duplicating the procedure for C1e in ref.30 (no yield therein), the authors obtained the product as hydrochloride salt in 35–40% yield.
88. a) A. S. Lyakhov, P. N. Gaponik, and S. V. Voitekhovich, Acta Cryst., 2001, C57, 185; b) P. N. Gaponik, S. V. Voitekhovich, A. S. Lyakhov, V. E. Matulis, O. A. Ivashkevich, M. Quesada, and J. Reedijk, Inorg. Chim. Acta, 2005, 358, 2549. CrossRef
89. a) J. C. Gálvez-Ruiz, G. Holl, K. Karaghiosoff, T. M. Klapötke, K. Löhnwitz, P. Mayer, H. Nöth, K. Polborn, C. J. Rohbogner, M. Suter, and J. J. Weigand, Inorg. Chem., 2005, 44, 4237; CrossRef for compound (C1e), see in addition: (i) ibid., p. 5192; (ii) M. A. Hiskey, Chem. Eng. News, 2005, 83, 5; b) compound (35a; X = NO3) could not be converted in that way; c) for large-scale preparation of C1e, cf.: Chem. Abstr., 2009, 152, 12254 [C. He, Z. Du, X. Cong, Z. Tang, and L. Meng, Proceedings of the 8th International Autumn Seminar on Propellants, Explosives and Pyrotechnics (Kunming, China), 2009, 8, 673; this document inavailable to the author].
90. a) M. Wagatsuma, S. Hatsuno, T. Yamaguchi, and S. Ohshima (Tanabe Seiyaku Co., Ltd., Japan), Eur. Pat. Appl., 1983, EP 81971 A2 (Chem. Abstr., 1983, 99, 122179); b) K. Azuma, M. Noguchi, S. Saijo, S. Hatsuno, M. Gaino, and M. Sofugawa (Tanabe Seiyaku Co., Ltd., Japan), Jpn. Kokai Tokkyo Koho, 1984, 59-106475 (Chem. Abstr., 1984, 101, 211156); c) Shionogi Co., Ltd., Japan, Jpn. Kokai Tokkyo Koho, 1984, 59-128377 (Chem. Abstr., 1985, 102, 6500).
91. Y. Nishitani, T. Aoki, T. Yoshida, and W. Nagata, J. Antibiotics, 1988, 41, 316.
92. E. Müller, R. Beutler, and B. Zeeh, Liebigs Ann. Chem., 1968, 719, 72. CrossRef
93. a) H. Bredereck, B. Föhlisch, and K. Walz, Angew. Chem., 1964, 76, 580; CrossRef Angew. Chem. Int. Ed., 1964, 3, 647; b) H. Bredereck, B. Föhlisch, and K. Walz, Liebigs Ann. Chem., 1965, 688, 93. CrossRef
94. D. Moderhack, K.-H. Goos, and L. Preu, Chem. Ber., 1990, 123, 1575. CrossRef
95. V. L. Nirenburg and I. Ya. Postovski, Khim. Geterotsikl. Soedin., 1965, 946; Chem. Heterocycl. Compd. (USSR), 1965, 1, 647. CrossRef
96. G. L'abbé, A. Vandendriessche, G. Van Essche, and S. Toppet, Bull. Soc. Chim. Belg., 1988, 97, 543.
97. M. A. A. Mustafa, A. M. Ismaiel, H. M. Eisa, and A. A. El-Emam, J. Chin. Chem. Soc., 1991, 38, 199.
98. Compound (40a) could be obtained only on brief heating of 39b (2 min at 160–170 °C), but even then the product was contaminated by ≤ 10% of C1ga (D. Moderhack, unpublished result).
99. a) C. Foces-Foces, F. Hernández Cano, R. M. Claramunt, D. Sanz, J. Catalán, F. Fabero, A. Fruchier, and J. Elguero, J. Chem. Soc., Perkin Trans. 2, 1990, 237; CrossRef b) the reverse relationship (ap higher in energy than sp) as shown in ref.99a is an obvious misprint (cf. ref.99c); c) R. M. Claramunt, D. Sanz, J. Catalán, F. Fabero, N. A. García, C. Foces-Foces, A. L. Llamas-Saiz, and J. Elguero, J. Chem. Soc., Perkin Trans. 2, 1993, 1687; CrossRef d) basic pKa values of C1/D1 do not seem to have been reported.
100. V. G. Andrianov, M. A. Shokhen, and A. V. Eremeev, Khim. Geterotsikl. Soedin., 1989, 1221; Chem. Heterocycl. Compd. (USSR), 1989, 25, 1021. CrossRef
101. a) Z.-X. Chen, H.-M. Xiao, and B.-H. Gao, Chin. J. Chem., 1999, 17, 114; CrossRef b) total energies (absolute minima) of C1a (ap)/C1a (sp)/D1a (sp)/D1a (ac) as well as C1e (ap)/C1e (sp)/D1e (sp)/D1e (ac): –313.57950/.57265/.58237/.58234 and –368.94610/.93688/.94679/.94600 a.u. (all values: D. Moderhack, unpublished results); c) ref.,35b while showing the lower energy of D1a compared to C1a, does not comment on the geometry of the species.
102. S. V. Voitekhovich, P. N. Gaponik, A. S. Lyakhov, and O. A. Ivashkevich, Tetrahedron, 2008, 64, 8721. CrossRef
103. M. Göbel and T. M. Klapötke, Adv. Funct. Mater., 2009, 19, 347. CrossRef
104. R. Bouchet, C. Coquelet, and J. Elguero, J. Chem. Soc., Perkin Trans. 2, 1974, 449. CrossRef
105. a) C.-L. He, Z.-M. Du, Z.-Q. Tang, X.-M. Cong, and L.-Q. Meng, Acta Cryst., 2009, E65, o1902; b) C.-L. He, Z.-M. Du, Z.-Q. Tang, X.-M. Cong, and L.-Q. Meng, Acta Cryst., 2009, E65, o2901.
106. For this degradation, cf.: a) R. A. Olofson, W. R. Thompson, and J. S. Michelman, J. Am. Chem. Soc., 1964, 86, 1865; CrossRef A. C. Rochat and R. A. Olofson, Tetrahedron Lett., 1969, 3377; CrossRef b) D. M. Zimmerman and R. A. Olofson, Tetrahedron Lett., 1970, 3453. CrossRef
107. S. M. Condon (Tetralogic Pharmaceuticals Corporation, USA), PCT Int. Appl., 2008, WO 014238
A2 (Chem. Abstr., 2008, 148, 175846). — Document without synthetic details.
108. V. G. Prokudin, V. S. Poplavsky, and V. A. Ostrovskii, Izv. Akad. Nauk, Ser. Khim., 1996, 2209; Russ. Chem. Bull., 1996, 45, 2094. CrossRef
109. M. A. M. Taha, J. Indian Chem. Soc., 2005, 82, 172. — For further unlikely cyclizations of C1e, see later (Scheme 33), including a note on the general existence of azolotetrazoles.
110. M. H. Heravi, S. Sadjadi, R. Hekmatshoar, H. A. Oskooie, and F. F. Bamoharram, Monatsh. Chem., 2008, 139, 107. CrossRef
111. a) O. V. Dyablo, A. V. Mikhailus, A. F. Pozharskii, and D. S. Trishkin, Khim. Geterotsikl. Soedin., 2005, 379; Chem. Heterocycl. Compd., 2005, 41, 329; CrossRef b) A. C. Chaskar, B. P. Langi, A. Deorukhkar, and H. Deokar, Synth. Commun., 2009, 39, 604. CrossRef
112. a) M. A. Ilyushin, A. N. Terpigorev, and I. V. Tselinskii, Zh. Obshch. Khim., 1999, 69, 1727; Russ. J. Gen. Chem., 1999, 69, 1654; b) M. A. Ilyushin and I. V. Tselinskii, Centr. Eur. J. Energ. Mater., 2006, 3, 39.
113. For example: T. J. Nitz and D. C. Pevear (Viropharma Inc., USA), PCT Int. Appl., 1999, WO 38508 (Chem. Abstr., 1999, 131, 144606).
114. A. V. Khramchikhin, V. A. Ostrovskii, and M. D. Stadnichuk, Zh. Obshch. Khim., 1991, 61, 1617; J. Gen. Chem., 1991, 61, 1484.
115. S. M. Desenko, V. D. Orlov, P. N. Gaponik, and V. P. Karavai, Khim. Geterotsikl. Soedin., 1990, 1533; Chem. Heterocycl. Compd. (USSR), 1990, 26, 1278. CrossRef
116. a) V. I. Krutikov, A. L. Kovalenko, and E. V. Sukhanovskaya, Zh. Obshch. Khim., 1991, 61, 257; J. Gen. Chem., 1991, 61, 234; b) V. I. Krutikov, A. L. Kovalenko, I. A. Tsar'skova, and E. V. Sukhanovskaya, Zh. Obshch. Khim., 1992, 62, 1671; J. Gen. Chem., 1992, 62, 1373.
117. M. Tremblay, Can. J. Chem., 1965, 43, 1154. CrossRef
118. K.-H. Goos, 'Synthese und Eigenschaften 1- und 2-substituierter 5-(Diazomethyl)tetrazole,' Dissertation, Technical University of Braunschweig (Germany), 1985.
119. For the formation of a stable covalent adduct, cf. D. Moderhack, Liebigs Ann. Chem., 1972, 758, 29. CrossRef
120. R. L. Willer and R. A. Henry, J. Org. Chem., 1988, 53, 5371. CrossRef
121. a) N. N. Kolos, B. V. Paponov, and V. D. Orlov, Khim. Geterotsikl. Soedin., 2003, 310; Chem. Heterocycl. Compd., 2003, 39, 280; CrossRef b) N. N. Kolos, B. V. Paponov, V. D. Orlov, M. I. Lvovskaya, A. O. Doroshenko, and O. V. Shishkin, J. Mol. Struct., 2006, 785, 114. CrossRef
122. Compounds (47b) and (47c) were obtained as an inseparable 1 : 1 mixture. Remarkably, the glyoxaldiimine (C14bb) – rather than 47a – was formed when 2 eq. of C1e were used (cf. Scheme 29).
123. D. D. Nekrasov, S. N. Shurov, O. I. Ivanenko, and Yu. S. Andreichikov, Zh. Org. Khim., 1994, 30,
126; Russ. J. Org. Chem., 1994, 30, 136.
124. D. Moderhack and M. Noreiks, unpublished result.
125. Cf. D. Moderhack, 'Comprehensive Heterocyclic Chemistry II: Bicyclic 5-5 Systems with One Ring Junction Nitrogen Atom: Four Extra Heteroatoms 3:1,' Vol. 8, ed. by G. Jones (Series ed. by A. R. Katritzky, C. W. Rees, and E. F. Scriven), Pergamon, Oxford, 1996, pp. 227–236; see in addition: I. Alkorta, F. Blanco, and J. Elguero, Tetrahedron, 2010, 66, 5071. CrossRef
126. H. Xue, B. Twamley, and J. M. Shreeve, Inorg. Chem., 2005, 44, 7009. CrossRef
127. a) A. Gao, Y. Oyumi, and T. B. Brill, Combust. Flame, 1991, 83, 345; CrossRef b) S. V. Levchik, A. I. Balabanovich, O. A. Ivashkevich, A. I. Lesnikovich, P. N. Gaponik, and L. Costa, Thermochim. Acta, 1993, 225, 53; CrossRef c) A. I. Lesnikovich, O. A. Ivashkevich, S. V. Levchik, A. I. Balabanovich, P. N. Gaponik, and A. A. Kulak, Thermochim. Acta, 2002, 388, 23; CrossRef d) L.-N. Feng, J.-G. Zhang, T.-L. Zhang, Y.-J. Shu, L. Yang, and H.-H. Zheng, Hanneng Cailiao, 2009, 17, 113 (Chem. Abstr., 2009, 150, 494327).
128. a) H. Xue, S. W. Arritt, B. Twamley, and J. M. Shreeve, Inorg. Chem., 2004, 43, 7972; CrossRef b) H. Xue, Y. Gao, B. Twamley, and J. M. Shreeve, Chem. Mater., 2005, 17, 191. CrossRef
129. G. W. Drake, T. W. Hawkins, J. Boatz, L. Hall, and A. Vij, Propell., Explos., Pyrotech., 2005, 30, 156. CrossRef
130. a) V. E. Matulis, A. S. Lyakhov, P. N. Gaponik, S. V. Voitekhovich, and O. A. Ivashkevich, J. Mol. Struct., 2003, 649, 309; CrossRef b) T. M. Klapötke and C. Miró Sabaté, Eur. J. Inorg. Chem., 2008, 5350.
131. Y. Cui, T. L. Zhang, J. G. Zhang, X. C. Hu, J. Zhang, and H. S. Huang, Chin. J. Chem., 2008, 26, 426. CrossRef
132. Y.-H. Joo and J. M. Shreeve, Chem. Eur. J., 2009, 15, 3198. CrossRef
133. a) H. Xue, H. Gao, B. Twamley, and J. M. Shreeve, Chem. Mater., 2007, 19, 1731; CrossRef b) G.-H. Tao, Y. Guo, D. A. Parrish, and J. M. Shreeve, J. Mater. Chem., 2010, 20, 2999. CrossRef
134. S. D. Shaposhnikov, T. V. Romanova, N. P. Spiridonova, S. F. Mel'nikova, and I. V. Tselinskii, Zh. Org. Khim., 2004, 40, 922; Russ. J. Org. Chem., 2004, 40, 884. CrossRef
135. T. M. Klapötke and J. Stierstorfer, Eur. J. Inorg. Chem., 2008, 4055.
136. T. M. Klapötke, P. Mayer, A. Schulz, and J. J. Weigand, J. Am. Chem. Soc., 2005, 127, 2032. CrossRef
137. G.-H. Tao, Y. Guo, Y.-H. Joo, B. Twamley, and J. M. Shreeve, J. Mater. Chem., 2008, 18, 5524. CrossRef
138. G.-H. Tao, Y. Huang, J. A. Boatz, and J. M. Shreeve, Chem. Eur. J., 2008, 14, 11167. CrossRef
139. Cf. a) R. P. Joo, R. D. Verma, D. T. Meshri, and J. M. Shreeve, Angew. Chem., 2006, 118, 3664; Angew. Chem. Int. Ed., 2006, 45, 3584; b) R. P. Singh, H. Gao, D. T. Meshri, and J. M. Shreeve, 'Structure and Bonding / High Energy Density Materials: Nitrogen-Rich Heterocycles,' Vol. 125, ed. by T. M. Klapötke, Springer, Berlin, 2007, pp. 35–83. — See also; c) T. M. Klapötke, 'Structure and Bonding / High Energy Density Materials: New Nitrogen-Rich Explosives,' Vol. 125, ed. by T. M. Klapötke, Springer, Berlin, 2007, pp. 85–121; d) G. Steinhauser and T. M. Klapötke, Angew. Chem., 2008, 120, 3376; Angew. Chem. Int. Ed., 2008, 47, 3330. CrossRef
140. For O1ea, see in addition: a) J. Geith, T. M. Klapötke, J. Weigand, and G. Holl, Propell., Explos., Pyrotech., 2004, 29, 3; CrossRef b) J. A. Ciezak, Propell., Explos., Pyrotech., 2010, 35, 24.
141. For O1de-g, see in addition: G. Fischer, G. Holl, T. M. Klapötke, and J. J. Weigand, Thermochim. Acta, 2005, 437, 168. CrossRef
142. See in addition: a) H. Gao, C. Ye, C. M. Piekarski, and J. M. Shreeve, J. Phys. Chem. (C), 2007, 111, 10718; CrossRef b) D. D. Zorn, J. A. Boatz, and M. S. Gordon, J. Phys. Chem. (B), 2006, 110, 11110; CrossRef c) X. Zhang, W. Zhu, T. Wei, C. Zhang, and H. Xiao, J. Phys. Chem. (C), 2010, 114, 13142. CrossRef
143. a) D. Moderhack and A. Lembcke, J. Chem. Soc., Perkin Trans. 1, 1986, 1157 (see also p. 2009); CrossRef b) D. Moderhack and D.-O. Bode, J. Chem. Soc., Perkin Trans. 1, 1992, 1483. CrossRef