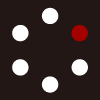
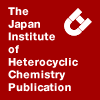
HETEROCYCLES
An International Journal for Reviews and Communications in Heterocyclic ChemistryWeb Edition ISSN: 1881-0942
Published online by The Japan Institute of Heterocyclic Chemistry
e-Journal
Full Text HTML
Received, 25th January, 2011, Accepted, 7th March, 2011, Published online, 11th April, 2011.
DOI: 10.3987/REV-11-695
■ Survey of Briarane-Type Diterpenoids – Part IV
Ping-Jyun Sung,* Jui-Hsin Su, Wei-Hsien Wang, Jyh-Horng Sheu, Lee-Shing Fang, Yang-Chang Wu, Yung-Husan Chen, Hsu-Ming Chung, Yin-Di Su, and Yu-Chia Chang
, National Museum of Marine Biology and Aquarium, 2 Houwan Road, Checheng, Pingtung, Taiwan 944, Taiwan, R.O.C.
Abstract
The structures, names, bioactivities, and references of 90 briarane-type diterpenoids, including 59 new compounds, are described in this review. All the briarane-type compounds mentioned in this review article were obtained from various octocorals including Briareum, Dichotella, Ellisella, Junceella, Menella, Verrucella, and Ptilosarcus. Some of these compounds showed potential bioactivities.1. INTRODUCTION
This review is of the literature from Sep. 2008 to Feb. 2011 and describes 90 briarane-type diterpenoids (including 59 new compounds), which possess a bicyclo[8.4.0] carbon skeleton and most possess a γ-lactone moiety in structures (Figure 1). As in previous reviews,1–3 we showed the structures, names, bioactivities, and references for these briaranes. Most briarane derivatives mentioned in this article were isolated from the octocorals belonging to the order Gorgonacea, including Briareum excavatum, Briareum sp., Dichotella gemmacea, Ellisella robusta, Junceella fragilis, Junceella juncea, Menella sp., and Verrucella sp.; and the order Pennatulacea, including Ptilosarcus gurneyi. The first iodine-containing briaranes, dichotellides A–E, were found in a gorgonian Dichotella gemmacea.4 This survey of briarane-type compounds will be presented taxonomically according to genus and species.
2. GORGONACEA
2.1. Briareum (family Briareidae)
A. Briareum excavatum
The gorgonian corals belonging to the genus Briareum played a main role to produce the briarane-type natural products. In the continuing studies on the Formosan octocorals, the gorgonian B. excavatum (including cultured type and field type), was further studied for their complex and interesting chemical constituents. 27 briarane derivatives, including 26 new compounds, briaexcavatins Q–Z (1–10),5–7 excavatoids A–P (11–26),8–12 and a known metabolite, briaexcavatin I (27),8,13 were isolated from Briareum excavatum (Table 1). The structures of new briaranes 1–26 were determined by spectroscopic methods and the structures for briaexcavatins U (5), W (7), and excavatoid A (11) were further confirmed by single-crystal X-ray diffraction analyses.6–8 The absolute configuration of 5 was also determined directly by its X-ray structure.6 The X-ray structure for the known briarane, briaexcavatin I (27), was reported for the first time.8 Briaranes 1–12 and 15–27 were isolated from a cultured type B. excavatum.5–12
Among the above compounds, briaexcavatin Y (9) is the first briarane containing a C-8/9 epoxy group.7 Excavatoid A (11) is the first briarane possessesing six hydroxy groups and a 17-methoxy group.8 Excavatoid C (13) is the first 12,13-secobriarane possessing a novel pentacyclic skeleton with an ε-lactone.8 Excavatoid P (26) is the only briarane known to possess a 6β-chlorine atom.12 The relationships between 13C NMR chemical shifts and the conformations of briaranes possessing an 11,12-epoxy group are also described.7 The 11,12-epoxide was assigned as α configuration because the 13C NMR chemical shifts for these two carbons were δC < 60 ppm (δC 57–60 ppm); while the chiral carbons C-11 and C-12 existed in S* and R* form, respectively, and leading the epoxy group to α-orientation. If the epoxy group was found to exist in β-configuration (11R* and 12S*), the 13C chemical shifts for C-11 were shifted downfield and appeared at δC 61–66 ppm, and most chemical shifts for C-12 in these briaranes were δC > 60 ppm.7 It is noteworthy to note that the natural briaranes possessing an 11,12-epoxy group in β form were all isolated from the octocorals distributed in the West Pacific Ocean.7
In the biological activity testing, briaexcavatin S (3) and excavatoids H–J (18–20) exhibited weak cytotoxicity toward various tumor cells.5,10 Briaexcavatins V (6), X (8), Y (9),7 and excavatoids D–F (14–16),8,9 L–P (22–26),11,12 showed various inhibitory effects on superoxide anion generation or elastase release by human neutrophils at 10 µg/mL. Briaexcavatin Z (10) was not active in inhibition of superoxide anion generation but this compound exhibited mild activity to enhance human neutrophil elastase release at 10 µg/mL.7
B. Briareum sp.
In continuing studies on the chemical constituents of a gorgonian coral identified as Briareum sp., collected from a tank equipped with a flow-through water system, located in the National Museum of Marine Biology and Aquarium, Taiwan, a new hydroperoxybriarane, briarenolide D (28), and a known briarane, 2β-acetoxy-2-(debutyryloxy)stecholide E (29), were obtained (Table 2).14,15 The structure of hydroperoxybriarane 28 was established by spectroscopic methods and the structure of 29 was confirmed by a single-crystal X-ray diffraction analysis for the first time.14 In previous studies, only two hydroperoxybriaranes, brianthein B and briarenolide B, were isolated from the gorgonian corals Briareum excavatum and Briareum sp., collected off Indonesian and Taiwan waters, respectively.16,17 Briarenolide D (28) is the third briarane possessesing a hydroperoxy group. It is interesting to note that the methylenecyclohexane ring in 28 was found to possess a twist boat conformation by the interpretation of proton chemical shifts and NOESY correlations analyses.14,18 The natural products of this type (hydroperoxybriarane) could be a chemical marker for the gorgonian corals belonging to the genus Briareum. Briarenolide D (28) exhibited moderate cytotoxicity toward DLD-1 and CCRF-CEM cells.14
2.2. Dichotella (family Ellisellidae)
A. Dichotella gemmacea
The gorgonian corals belonging to the family Ellisellidae also played major sources of briarane-type natural products.1–3 The South China Sea gorgonian coral D. gemmacea was found to contain nine chlorinated briarane-related natural products, including five new compounds, dichotellides A–E (30–34) (Table 3),4 and four known briaranes, praelolide, juncin P, juncin ZI, junceellin A.19 The structures of new briaranes 30–34 were established by spectroscopic methods, and the absolute stereochemistry of dichotellide A (30) was further confirmed by a single-crystal X-ray diffraction analysis.4 Dichotellides A–E (30–34) are the first examples of iodine-containing briarane-type natural products.4 Dichotellide C (32) showed marginal cytotoxicity toward SW1990 cells.4
2.3. Ellisella (family Ellisellidae)
A. Ellisella robusta
Five chlorinated briaranes, including four new compounds, robustolides J–L (35–37) and 12-epi-fragilide G (38),5,20,21 along with a known compound, robustolide H (39),20,22 were further isolated from a Formosan gorgonian coral E. robusta (Table 4). The structures of new briaranes 35–38 were established by spectral data analysis. Both the six-membered rings in briaranes 35 and 37 were found to exist in twist boat conformation.5,20 The X-ray structure, including the absolute stereochemistry, for the known briarane, robustolide H (39), was also reported for the first time.20 The structure for a known briarane, juncin F,23 which was first isolated from a gorgonian coral Junceella juncea, collected off the Red Sea, was further established by comparison of the proton chemical shifts and coupling patterns with those of robustolide H (39).5
Robustolides J–L (35–37) and 12-epi-fragilide F (38) showed inhibitory effects on superoxide anion or elastase release by human neutrophils and robustolide L (37) exhibited weak cytotoxicity toward IMR-32 cells.5,20,21
2.4. Junceella (family Ellisellidae)
A. Junceella fragilis
Study on the gorgonian coral J. fragilis, collected off the Southern Taiwan coast, has afforded six new briarane derivatives, fragilides E–J (40–45) (Table 5-1),18,20,24,25 along with three known compounds, junceellonoid D, juncin Z, and (+)-11β,20β-epoxyjunceellolide D.26,27 The structures of new briaranes 40–45 were established by spectroscopic methods. The absolute configuration of fragilide F (41) was determined by its X-ray structure.18 Fragilide I (44) is the first briarane possessing a 9-isovaleroxy group.25 Fragilides E (40) and J (45) displayed weak inhibitory effects on elastase release by human neutrophils,20,24 and juncin Z exhibited significant cytotoxicity towad CCRF-CEM cells (IC50 = 1.6 µg/mL).18
In addition, seven new briaranes, frajunolides E–K (46–52) (Table 5-2),28 and 14 known briaranes, praelolide, junceellin, junceellolides A–E and K, (–)-11β,20β-epoxy-4-deacetoxyjunceellolide D,27 umbraculolide A, junceellonoid A, and juncins Y, Z, ZI,29 were isolated from the gorgonian coral J. fragilis, collected in Taitong County, Taiwan. The structures of new briaranes 46–52 were established by spectroscopic methods. It has to be noted that the structure of briarane 48 (frajunolide G) had been reported in a previous study and named as fragilide D.30 Frajunolides E (46), F (47), I (50), and J (51) exhibited weak inhibitory effects on superoxide anion generation or elastase release by human neutrophils.28 Based on the characteristics of chemical shifts for the briarane derivatives contained a C-11/20 carbon-carbon double bond, the chemical shifts for the olefin protons H2-20 were summed up; these appear at δH 4.95–5.30 ppm and δH 4.85–5.15 ppm, while the cyclohexane rings to show a twist boat conformation. Furthermore, the proton NMR data for H2-20 appeared at δH 4.95–5.10 ppm and δH 4.40–4.75 ppm, the cyclohexane rings were found to exist in a chair conformation.18
B. Junceella juncea
Fvie new 8-hydroxybriaranes, junceols D–H (53–57) (Table 6-1), were isolated from a Formosan gorgonian coral Junceella juncea.31 The structures of briaranes 53–57 were determined on the basis of spectroscopic methods and the methylenecyclohexane rings in 53–57 were found to exist in twist boat form. Junceols D (53) and F–H (55–57) exhibited cytotoxicity toward CCRF-CEM or DLD-1 cells and junceols E–H (54–57) displayed weak inhibitory effects on superoxide anion generation by human neutrophils at 10 µg/mL.31
In 2009, Qi et al., reported the occurrence of a new briarane derivative, juncin ZII (58) (Table 6-2),32 along with three known metabolties, gemmacolides C, F, and (+)-11β,20β-epoxyjunceellolide D,27,33 from a gorgonian coral Junceella juncea, collected at the South China Sea.32 The structure of briarane 58 was established by spectroscopic method and this compound showed potential antifeedant and antifouling activity.32 Moreover, a series of briarane derivatives, isolated previously from J. juncea by Qi’s group were assayed for their potential antifeedant activity, cytotoxicity, and antifouling activity.32,34,35
Chemical investigation on the gorgonian J. juncea, collected in the Taitong County, Taiwan, had yielded four new briaranes, juncenolides H–K (59–62) (Table 6-3).36 The structures for briaranes 59–62 were determined on the basis of spectral data analysis.36
2.5. Menella (family Ellisellidae)
A. Menella sp.
Two well known briaranes, junceellolides B and D, which were first isolated from the gorgonian coral Junceella fragilis,37 were isolated from a gorgonian coral, identified as Menella sp., collected off Meishan Island, Hainan province, China.38 Junceellolides B and D were the first two briarane-type natural products found in the gorgonian corals belonging to the genus Menella.38
2.6. Verrucella (family Ellisellidae)
A. Verrucella sp.
A well known chlorinated briarane, junceellin, which was first isolated from the gorgonian coral Junceella squamata,39 was obtained from a gorgonian coral, identified as Verrucella sp., collected off the South China Sea.40 Junceellin was the first briarane-type natural product found in the gorgonian corals belonging to the genus Verrucella.40
3. PENNATULACEA
3.1. Ptilosarcus (family Pennatulidae)
A. Ptilosarcus gurneyi
An insecticidal briarane, ptilosarcenone (63), which was first isolated from a North Pacific Ocean sea pen coral, Ptilosarcus gurneyi,41,42 was obtained from the same species collected near Juneau, Alaska, 2006 (Table 7). The structure, including the absolute stereochemistry of ptilosarcenone was determined directly by a single-crystal X-ray study for the first time.43
4. CONCLUSION
All the briarane-type compounds are proven to be marine origin, and up to date, over 500 new briarane-type natural diterpenoids had been isolated from various marine organisms, particularly with the soft corals belonging to the subclass Octocorallia. The structural novelty and interesting bioactivities of briarane derivatives are still prompted continuing attention. A series of semi-synthetic briarane derivatives are prepared from the well-known briaranes, such as briantheins X and Y, for their potential insecticidal activity.44 The highly functionalized fragment corresponding to the Northern hemisphere of the briaranes also has been synthesized employing a series of organic reactions.45 However, due to the structural complexity, it is difficult to synthesize the potential briaranes by chemical methods totally. Based on this point, we have to coordinate and make the best use of highly developed aquaculture technology to enhance in captivity mass production of raw material needed for extraction of marine natural product compounds such as briarane diterepnoids which also protect natural population and natural habits form over exploitation.
ACKNOWLEDGEMENT
This work was supported by grants from the National Museum of Marine Biology and Aquarium (Grant No. 100100101 and 100200311); National Dong Hwa University; Asia-Pacific Ocean Research Center, National Sun Yat-sen University (98C0317020; and National Science and Technology Program for Biotechnology and Pharmaceuticals, National Science Council (NSC 99-2323-B-291-001 and NSC 98- 2320-B-291-001-MY3), Taiwan, awarded to P.-J.S.
References
1. P.-J. Sung, J.-H. Sheu, and J.-P. Xu, Heterocycles, 2002, 57, 535. CrossRef
2. P.-J. Sung, P.-C. Chang, L.-S. Fang, J.-H. Sheu, W.-C. Chen, Y.-P. Chen, and M.-R. Lin, Heterocycles, 2005, 65, 195. CrossRef
3. P.-J. Sung, J.-H. Sheu, W.-H. Wang, L.-S. Fang, H.-M. Chung, C.-H. Pai, Y.-D. Su, W.-T. Tsai, B.-Y. Chen, M.-R. Lin, and G.-Y. Li, Heterocycles, 2008, 75, 2627. CrossRef
4. J.-F. Sun, H. Huang, X.-Y. Chai, X.-W. Yang, L. Meng, C.-G. Huang, X.-F. Zhou, B. Yang, J. Hu, X.-Q. Chen, H. Lei, L. Wang, and Y. Liu, Tetrahedron, 2011, 67, 1245. CrossRef
5. T.-L. Hwang, M.-R. Lin, W.-T. Tsai, H.-C. Yeh, W.-P. Hu, J.-H. Sheu, and P.-J. Sung, Bull. Chem. Soc. Jpn., 2008, 81, 1638. CrossRef
6. P.-J. Sung, M.-R. Lin, and M. Y. Chiang, Chem. Lett., 2009, 38, 154. CrossRef
7. P.-J. Sung, M.-R. Lin, M. Y. Chiang, and T.-L. Hwang, Bull. Chem. Soc. Jpn., 2009, 82, 987. CrossRef
8. P.-J. Sung, Y.-D. Su, G.-Y. Li, M. Y. Chiang, M.-R. Lin, I-C. Huang, J.-J. Li, L.-S. Fang, and W.-H. Wang, Tetrahedron, 2009, 65, 6918. CrossRef
9. P.-J. Sung, B.-Y. Chen, M.-R. Lin, T.-L. Hwang, W.-H. Wang, J.-H. Sheu, and Y.-C. Wu, Mar. Drugs, 2009, 7, 472. CrossRef
10. P.-J. Sung, B.-Y. Chen, M. Y. Chiang, C.-H. Hou, Y.-D. Su, T.-L. Hwang, Y.-H. Chen, and J.-J. Chen, Bull. Chem. Soc. Jpn., 2010, 83, 539. CrossRef
11. J.-H. Su, B.-Y. Chen, T.-L. Hwang, Y.-H. Chen, I.-C. Huang, M.-R. Lin, J.-J. Chen, L.-S. Fang, W.-H. Wang, J.-J. Li, J.-H. Sheu, and P.-J. Sung, Chem. Pharm. Bull., 2010, 58, 662. CrossRef
12. P.-J. Sung, G.-Y. Li, Y.-D. Su, M.-R. Lin, Y.-C. Chang, T.-H. Kung, C.-S. Lin, Y.-H. Chen, J.-H. Su, M.-C. Lu, J. Kuo, C.-F. Weng, and T.-L. Hwang, Mar. Drugs, 2010, 8, 2639. CrossRef
13. P.-J. Sung, M.-R. Lin, Y.-D. Su, M. Y. Chiang, W.-P. Hu, J.-H. Su, M.-C. Cheng, T.-L. Hwang, and J.-H. Sheu, Tetrahedron, 2008, 64, 2596. CrossRef
14. P.-J. Sung, M.-R. Lin, M. Y. Chiang, I.-C. Huang, S.-M. Syu, L.-S. Fang, W.-H. Wang, and J.-H. Sheu, Chem. Lett., 2010, 39, 1030. CrossRef
15. J.-H. Sheu, P.-J. Sung, L.-H. Huang, S.-F. Lee, T. Wu, B.-Y. Chang, C.-Y. Duh, L.-S. Fang, K. Soong, and T.-J. Lee, J. Nat. Prod., 1996, 59, 935. CrossRef
16. S. Aoki, M. Okano, K. Matsui, T. Itoh, R. Satari, S. Akiyama, and M. Kobayashi, Tetrahedron, 2001, 57, 8951. CrossRef
17. J.-H. Su, P.-J. Sung, Y.-H. Kuo, C.-H. Hsu, and J.-H. Sheu, Tetrahedron, 2007, 63, 8282. CrossRef
18. P.-J. Sung, S.-H. Wang, M. Y. Chiang, Y.-D. Su, Y.-C. Chang, W.-P. Hu, C.-Y. Tai, and C.-Y. Liu, Bull. Chem. Soc. Jpn., 2009, 82, 1426. CrossRef
19. All the known briaranes described in ref. 4 had been reviewed in previous articles. Please see ref. 1–3.
20. S.-H. Wang, Y.-C. Chang, M. Y. Chiang, Y.-H. Chen, T.-L. Hwang, C.-F. Weng, and P.-J. Sung, Chem. Pharm. Bull., 2010, 58, 928. CrossRef
21. Y.-C. Chang, T.-L. Hwang, S.-K. Huang, L.-W. Huang, M.-R. Lin, and P.-J. Sung, Heterocycles, 2010, 81, 991. CrossRef
22. P.-J. Sung, W.-T. Tsai, M.-R. Lin, Y.-D. Su, C.-H. Pai, H.-M. Chung, J.-H. Su, and M. Y. Chiang, Chem. Lett., 2008, 37, 88. CrossRef
23. S. Isaacs, S. Carmely, and Y. Kashman, J. Nat. Prod., 1990, 53, 596. CrossRef
24. P.-J. Sung, G.-Y. Li, Y.-P. Chen, I.-C. Huang, B.-Y. Chen, S.-H. Wang, and S.-K. Huang, Chem. Lett., 2009, 38, 454. CrossRef
25. P.-J. Sung, S.-H. Wang, Y.-C. Chang, Y.-H. Chen, M.-R. Lin, I.-C. Huang, J.-J. Chen, J.-J. Li, T.-H. Kung, L.-S. Fang, W.-H. Wang, and C.-F. Weng, Bull. Chem. Soc. Jpn., 2010, 83, 1074. CrossRef
26. All the known briaranes described in ref. 18 and 25 had been reviewed in previous articles. Please see ref. 1–3.
27. The briarane (+)-11β,20β-epoxyjunceellolide D and (–)-11β,20β-epoxy-4-deacetoxyjunceellolide D
was originally named as (+)-11α,20α-epoxyjunceellolide D and (–)-11α,20α-epoxy-4-deacetoxy- junceellolide D, but the stereochemistry of 11,20-epoxy group in these two compounds had been revised as having a β-orientation and should be renamed as (+)-11β,20β-epoxyjunceellolide D and (–)-11β,20β-epoxy-4-deacetoxyjunceellolide D, respectively. Please see J.-H. Sheu, Y.-P. Chen, T.-L. Hwang, M. Y. Chiang, L.-S. Fang, and P.-J. Sung, J. Nat. Prod., 2006, 69, 269. CrossRef
28. C.-C. Liaw, Y.-C. Shen, Y.-S. Lin, T.-L. Hwang, Y.-H. Kuo, and A. T. Khalil, J. Nat. Prod., 2008, 71, 1551. CrossRef
29. All the known briaranes described in ref. 28 had been reviewed in previous articles. Please see ref. 1–3.
30. P.-J. Sung, C.-H. Pai, Y.-D. Su, T.-L. Hwang, F.-W. Kuo, T.-Y. Fan, and J.-J. Li, Tetrahedron, 2008, 64, 4224. CrossRef
31. P.-J. Sung, C.-H. Pai, T.-L. Hwang, T.-Y. Fan, J.-H. Su, J.-J. Chen, L.-S. Fang, W.-H. Wang, and J.-H. Sheu, Chem. Pharm. Bull., 2008, 56, 1276. CrossRef
32. S. H. Qi, S. Zhang, P. Y. Qian, and H. H. Xu, Chem. Nat. Comp., 2009, 45, 49. CrossRef
33. All the known briaranes described in ref. 32 had been reviewed in previous articles. Please see ref. 1–3.
34. S.-H. Qi, S. Zhang, H. Huang, Z.-H. Xiao, J.-S. Huang, and Q.-X. Li, J. Nat. Prod., 2004, 67, 1907. CrossRef
35. S.-H. Qi, S. Zhang, P.-Y. Qian, Z.-H. Xiao, and M.-Y. Li, Tetrahedron, 2006, 62, 9123. CrossRef
36. S.-S. Wang, Y.-H. Chen, J.-Y. Chang, T.-L. Hwang, C.-H. Chen, A. T. Khalil, and Y.-C. Shen, Helv. Chim. Acta, 2009, 92, 2092. CrossRef
37. J. Shin, M. Park, and W. Fenical, Tetrahedron, 1989, 45, 1633. CrossRef
38. X.-Y. Chai, J.-F. Sun, L.-Y. Tang, X.-W. Yang, Y.-Q. Li, H. Huang, X.-F. Zhou, B. Yang, and Y. Liu, Chem. Pharm. Bull., 2010, 58, 1391. CrossRef
39. Y. Lin and K. Long, Zhongshan Daxue Xuebao, Ziran Kexueban, 1982, 46.
40. N. Wang, W. Wang, X.-J. Liao, and S.-H. Xu, J. Instrum. Anal., 2010, 29, 608.
41. S. J. Wratten, W. Fenical, D. J. Faulkner, and J. C. Wekell, Tetrahedron Lett., 1977, 18, 1559. CrossRef
42. R. L. Hendrickson and J. H. Cardellina II, Tetrahedron, 1986, 42, 6565. CrossRef
43. D. J. Nurco, D. E. Conklin, N. S. Shapiro, and E. Tran, Acta Cryst., 2011, E67, o181. CrossRef
44. J. M. Cronan, A. Lee, J. Liang, J. Clardy, and J. H. Cardellina II, J. Nat. Prod., 2010, 73, 346. CrossRef
45. R. W. Bates, A. Pinsa, and X. Kan, Tetrahedron, 2010, 66, 6340. CrossRef