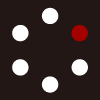
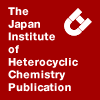
HETEROCYCLES
An International Journal for Reviews and Communications in Heterocyclic ChemistryWeb Edition ISSN: 1881-0942
Published online by The Japan Institute of Heterocyclic Chemistry
e-Journal
Full Text HTML
Received, 1st February, 2011, Accepted, 3rd March, 2011, Published online, 10th March, 2011.
DOI: 10.3987/COM-11-12162
■ Xanthones in Heterocyclic Synthesis. An Efficient Route for the Synthesis of C-3 o-Hydroxyaryl Substituted 1,2-Benzisoxazoles and Their N-Oxides, Potential Scaffolds for Angiotensin(II) Antagonist Hybrid Peptides
Yiannis Gardikis, Petros G. Tsoungas,* Constantinos Potamitis, Maria Zervou, and Paul Cordopatis*
Research & Technology, Ministry of Education, 14-18 Messogeion Ave., Athens, GR-115 10, Greece
Abstract
Regioselective substitution of xanthone and its nucleophilic cleavage allow the synthesis of C-3 o-hydroxyaryl substituted 1,2-benzisoxazoles or their N-oxides by cyclodehydration or oxidative cyclization of their corresponding ketoxime precursors, respectively. Molecular modeling analysis and 1H NMR spectra indicate an intramolecular H-bonding engaging phenol OH and the isoxazole ring N atom.INTRODUCTION
Molecules possessing the 1,2-oxazole (isoxazole) ring exhibit a wide range of biological activities and pharmacological properties1 making the ring an eminent target for elegant and efficient ways to its synthesis. The ring is also a precursor to useful synthetic intermediates such as γ-amino alcohols,2 β-hydroxy ketones,3 β-hydroxy nitriles4 or β,γ-unsaturated ketones.5
The most common reaction to form the isoxazole structure is 1,3-dipolar cycloaddition of alkynes with nitrile oxides, generated in situ, usually by dehydration of nitro compouds6 or by dehydrogenation of oximes.7 The formation of nitrile oxides from either β-keto esters or α,β-unsaturated ketones, using hydroxylamine, is known as a one-pot isoxazole synthesis.8,9
1,2-Benzoxazoles (benzisoxazoles) have long stood prominently in this class of heterocycles. Indeed, some recent reports on the synthesis of this structure, elegant in their simplicity and efficiency, serve as an irrefutable testimony to the continuous interest in the field. They involve, either cyclodehydration of o-hydroxyaryl aldo/ketoximes, triggered by PPh3/DDQ,10 TsCl/iPr2NEt,11 microwave in an ionic liquid12 or CuI/DMEDA/tBuONa13 or a [3+2] cycloaddition of in situ generated nitrile oxides with arynes,14 all under mild conditions.
1,2-Benzisoxazoles, substituted at C-3 with pharmacophores, on the other hand, is an area of intense research, driven by potential applications in pharmaceutics.15 Derivatives have been recently investigated as inhibitors of LTB4 binding to human neutrophiles,16 affinity ligands for serotonergic and dopaminergic receptors,17 selective inhibitors of acetylcholinesterase18 or for atypical antipsychotic activity.19
Bearing in mind the significance of substitution at that position, incorporation of a phenol, a medically reputed core unit,20 has been sought. This molecular scaffold will provide a diverse array of lead structures, amino acids included, for the synthesis of hybrid peptides as Angiotensin (II) antagonists.21
Accordingly, we have developed and report, herein, an efficient protocol for the synthesis of 3-[o- hydroxyaryl] substituted 1,2-benzisoxazoles (10-22) and their N-oxides (23-34) (Schemes 1 and 2).
RESULTS AND DISCUSSION
The adopted methodology makes use of xanthone 1 (Scheme 1). Its reactivity profile, developed by us, has been recently reported.22 Accordingly, converting 1 to its derivatives 2 or 5, leads to substitution patterns with a synthetically useful degree of regioselectivity.
Conventional electrophilic substitution was performed first. Nitration of 123 introduces the NO2 group at C-2 (or C-7) (Scheme 1, 2a). Bromination, on the other hand, introduces bromine at C-2, predominantly, to give 2b but C-7 is also attacked to a lesser extent to give the dibromo derivative 2c. Friedel-Crafts conditions gave 2b and 2c in 50% and 43% yields, respectively. Using a 10-fold excess of bromine in acetic acid at 100 ºC for 4h changed the yields of the bromo derivatives to 72% and 18%, respectively. 2c was also obtained stepwise from 2b in 40% yield. Clearly, the entries at C-2 and C-7 are facilitated and directed from the pyran O lone pair. These entries may serve as sites of further functionalisation, for example, a phenyl group can be incorporated into C-2 or C-7, under Suzuki conditions, giving 2d and 2e in 90% and 78% yields, respectively.
Complementary to the above described functionalisation of 1 is a lithiation-electrophilic quench protocol22 to 5 (Scheme 1). By means of this protocol, 1 is converted to its ketal derivative 4 via 9,9-dichloroxanthene 3.24 It is worth noting that 4 is stable enough to the substitution operations but it is rapidly hydrolysed to 5, upon work up.22
Having xanthones 2 and 5 regioselectively substituted, they undergo nucleophilically triggered ring opening to ketones 6 and 8 (Scheme 2).
The cleaving nucleophile, through an SNAr process, ends up ortho- to the ketone carbonyl. The cleavage is efficiently performed with alkali in ca. 80% yield while a moderate yield of ca. 50% is obtained when an alkoxide is used. In the latter case one of the OH groups is protected as its alkyl ether (Scheme 2, 6a or 6b).
Ketones are then converted to their ketoximes 727 and 9, cyclisation of which, ultimately leads to the target 1,2-benzisoxazoles (10-22) (Table 1) or their N-oxides (23-34) (Table 2), providing the o-hydroxyaryl group, regioselectively substituted or not, at their C-3 position.
If no substitution is required on the heterocycles then cyclodehydration of 7 or 9 by PPh3/DDQ10 or TsCl/iPr2NEt11 gives 10-12 or 16-18 (Table 1) while oxidative cyclisation of 7 or 9, using lead(IV)acetate (LTA)25 or phenyliodine(III)diacetate (PIDA)26 gives the N-oxides (23-25) or (29, 30) (Table 2), as single isomers in each case. This is clearly the result of having identical cyclisation sites on the oximes.
However, the synthetic potential of the scheme is amply demonstrated if regioselective substitution on the target heterocycles is desired.28
It is of interest to note that substitution patterns can be built up symmetrically (Table1, entries 17, 18 and Table 2, entries 29, 30) or unsymmetrically (Table 1, entries 13-15, 19-21 and Table 2, entries 26-28, 31-33). In the former case, cyclisation sites in 8 or 9 are identical, again, consequently, only a single isomer was obtained. On the contrary, an unsymmetrical substitution pattern gives rise to the two possible isomers, emanating from the alternative cyclisation modes of 8 or 9, in varying yields (Tables 1 and 2).
An interesting outcome arises from the cyclisation of the NO2-bearing oxime to give the corresponding 22 or 34. Apparently, the strongly electron withdrawing NO2 group engages its p-disposed OH group into a mesomeric interaction, thus, hampering its participation in the cyclization process, leading to the isolation of only one isomer.29
A molecular modeling analysis30 was performed on 17 and 23. Clustering of the results led to the lowest energy conformers (Figures 1 and 2).
Conformers A and B in 17 reveal an intramolecular H-bonding-like contact among phenol OH and ring N atom (Figure 1). A dihedral angle τ1 of ca. 133o and a 0.002Å elongation of OH bond, point to a rather weak O-H--N interaction. Apparently, it is this interaction and the relief of some C-3/C-13 steric congestion that force the phenol ring out of 1,2-benzisoxazole plane, locking, in a way, the structure into these particular conformations. The expected highly deshielded at ca. δ = 9.5ppm 1H signal in the 600 MHz 2D COSY spectrum of 17 appeared to be obscured. A similar H-bonding has been observed in the 1,3-benzoxazole isomer.31
A what appears to be a very weak intramolecular H-bonding interaction (δOH = 8.25 ppm and τ2 of ca. 125o) is also evident in conformers A and B in 23, among phenol OH and the O atom of the N-O dipole (Figure 2).
In conclusion, the reactivity profile of xanthone allows for a simple and efficient protocol developed for the synthesis of C-3- regioselectively substituted 1,2-benzoxazoles and their N-oxides. Todate this is the only available methodology to obtain these pharmacologically valuable structures. A diverse and virtually unlimited array of derivatives can thus be accessible.
EXPERIMENTAL
Melting points were measured on an Electrothermal IA9000 Series apparatus and are uncorrected. Infrared spectra were recorded or an FT/IR-5300 spectrometer as KBr discs. Elemental analyses were performed on a Carlo Erba 1106 analyser. NMR spectra were measured on a Bruker Avance 400 MHz and a Varian 600 MHz spectrometers, in CDCl3 or DMSO-d6 solutions. Mass spectra were recorded by Micromass-Platform LC or JEOL JMS-AX505W low or high resolution instruments. Analytical TLC was run on Fluka Silica Gel F254. Preparative Flash Chromatography was run on MERCK 9385 Silica Gel. Reagents were used as commercially purchased while solvents such as CH2Cl2, EtOAc, hexane and MeOH were purified and dried according to standard procedures.
Bromination of xanthone. General Procedures. Method A: Xanthone (1) (8.6 g, 50 mmol) in CS2 (20 mL) is mixed with aluminium chloride (14.5 g). Bromine (2.0 mL) is added dropwise and the mixture is stirred at room temperature for 24 h. Water is then added, followed by extraction with CH2Cl2 (3X80 mL). The combined extracts are repeatedly washed with water, dried over sodium sulphate, concentrated and chromatographed (EtOAc: pet. ether 4:1) to give 2-bromoxanthone (2b) (5.64 g, 45%) and 2,7-dibromoxanthone (2c) (4.95 g, 30%). Method B: Bromine (3.2 mL) in acetic acid (16 mL) is added dropwise to a solution of xanthone (7.8 g, 40 mmol) in acetic acid (24 mL) at 110 oC. After 4 h, the reaction is quenched with ice water and a precipitate is formed. This is collected by filtration, washed repeatedly with water, dried and chromatographed (EtOAc: pet. ether 4:1) to give 2b (8.5 g, 75%) and 2c (1.5 g, 10%).
2b: Mp 176 oC. IR: 1696, 1314 cm-1. 1H NMR (400 MHz, CDCl3): δ 8.45-8.35 (d, J = 2.4 Hz, 1H), 8.30-8.25 (dd, J = 8 Hz, 1.6 Hz, 1H), 7.75-7.65 (m, 2H), 7.45-7.40 (d, J = 8 Hz, 1H), 7.35-7.25 (m, 2H). 13C NMR (100 MHz, CDCl3): δ 115.9, 117.5, 118.5, 121, 123.4, 124.5, 126, 134.0, 135.7, 138.6, 154.6, 155.6, 175.2. ESMS (M+H): m/z 276. Anal. Calcd for C13H7BrO2: C, 56.72; H, 2.54. Found: C, 56.55;H, 2.40%.
2c: Mp 217 oC. IR: 1710, 1291 cm-1. 1H NMR (400 MHz, CDCl3): δ 8.45-8.35 (m, 2H), 7.9 (dd, J = 8.8 Hz, 3.2 Hz, 2H). 7.86-7.76 (m, 2H). 13C NMR (100 MHz, CDCl3): δ 116, 116.4, 118.3, 118.5, 123, 123.5, 133.6, 140, 138.6, 154.7, 175. ESMS (M+H: m/z 355. Anal. Calcd for C13H6Br2O2: C, 44.06; H, 1.69. Found: C, 43.88; H, 1.56%.
Nitration of xanthone. This was effected according to a literature method23 to give 2d, yield 58%. Mp 206 oC (lit.,23 207 oC). IR: 3080, 1670, 1612, 1531, 1338, 1280 cm-1. 1H NMR (400 MHz, CDCl3): δ 8.85 (d, J = 2.46, 1H), 8.60 (dd, J = 2.65 Hz, 2.71 Hz, 1H), 8.20 (d, J = 7.68 Hz, 1H), 7.91 (m, 2H), 7.71 (d, J = 8.45 Hz, 1H), 7.55 (t, J = 7.40 Hz, 7.57 Hz, 1H). ESMS (M+H): m/z 242.
Arylation of 2-bromoxanthone (2b). To a stirred solution of 2-bromoxanthone (2.5 g, 2 mmol) in toluene (45 mL), ethanol (45 mL) and aqueous 2M Na2CO3, phenylboronic acid (2.25 g, 4.16 mmol) and Pd (PPh3)4 (210 mg) are added at room temperature, under an Argon atmosphere and the mixture is heated to reflux for 2h. EtOH (100 mL) and H2O (10 mL) are then added, the organic layer is repeatedly washed with brine (3X50 mL), dried over sodium sulphate and chromatographed (EtOAc/petroleum ether 9:1 v/v) to give 2-phenylxanthone (2e) (2.3 g, 90%).
Mp 159 oC, Rf = 0,85. IR: 1687, 1325 cm-1. 1H NMR (400 MHz, CDCl3): δ 8.51 (d, J = 2.4 Hz, 1H), 8.29 (dd, J = 8 Hz, 1.6 Hz, 1H), 7.91 (dd, J = 8.8 Hz, 2.4 Hz, 1H), 7.78-7.61 (m, 4H), 7.55-7.25 (m, 5H). 13C NMR (100 MHz, CDCl3): δ 117.9, 118.3, 121.1, 121.5, 124.8, 125.9, 127.9, 128.1, 128.2, 130.1, 130.8, 130.9, 132.9, 134.6, 135.6, 140.8, 154.8, 155.8, 175.6. ESMS (M+H): m/z 273. Anal. Calcd for C19H12O2: C, 83.80; H, 4.41. Found: C, 83.62; H, 4.34%.
Arylation of 2c. The method described above was repeated and 2,7-diphenylxanthone 2e was obtained. Yield 78%. Mp 194 oC, Rf = 0.47. ESMS (M+H): m/z 349. Anal. Calcd for C25H16O2: C, 86.20; H, 4.59. Found: C, 85.99; H, 4.42%.
Nucleophilic cleavage of xanthones (2 and 5). General procedure: In a solution of xanthone (1.96 g, 10 mmol) in DMSO (20 mL), an aqueous solution of 12N KOH (30 mL) is added and the reaction mixture is heated under reflux for 9 h (cleavage of 2a may be accomplished by heating in 6N KOH for 8 h). The solvent is removed in vacuo and residue is treated with ice-water, slowly acidified with 10N HCl to pH=3 and exhaustively extracted with CH2Cl2 (5X50 mL). Combined extracts are repeatedly washed with water and brine, dried over sodium sulphate, concentrated and residue is triturated with Et2O/petroleum ether to give 2,2′-dihydroxybenzophenones 6 and 8.
Nucleophilic cleavage of xanthone 1. A solution of xanthone (1.0 g) in a sodium alkoxide RONa (R=Me, Et) (20 mL) was heated at 110 oC in a sealed tube for 9 h. The reaction mixture after ice cooling was concentrated and triturated as described above to give 2-hydroxy-2′-alkoxybenzophenones (6b) (48%) and (6c) (51%) as viscous oils.
IR: 3420, 3190, 1640 cm-1. 1H NMR (400 MHz, CDCl3): δ 10.48 (s, 1H) 7.71 (d, J = 2.44 Hz, 1H), 7.61-7.51 (m, 3H), 7.9 (d, J = 8.35 Hz, 1H) 7.01-6.96 (m, 3H). ESMS (M+H): m/z 215.
2-Hydroxy-2′-Methoxybenzophenone (6b): Yield 51%. Mp 112 oC. Rf = 0.84. IR (KBr) 3420, 1615cm-1. 1H NMR (400MHz, CDCl3): δ 10.10 (br, 1H, OH), 7.80-6.90 (m, 8H, aromatic), 3.80 (s, 3H, OMe). 13C NMR (100MHz, CDCl3): δ 157.5, 155.0, 148.1, 135.4, 131.2, 129.1, 128.0, 126.0, 125.8, 124.6, 122.2, 119.8, 118.2, 57.4. ESMS (M+H): m/z 229.
2-Hydroxy-2′-Ethoxybenzophenone (6c): Yield 48%. Mp 90 oC. Rf = 0.49 IR. (KBr) 3420, 1615cm-1. 1H NMR (400MHz, CDCl3): δ 10.10 (br, 1H, OH), 7.80-6.90 (m, 8H, aromatic), 4.2 (q, 2H, CH2Me), 3.80 (s, 3H, OMe). 13C NMR (400MHz, CDCl3): δ 157.5, 155.0, 148.1, 135.4, 131.2, 129.1, 128.0, 126.0, 125.8, 124.6, 122.2, 119.8, 118.2, 57.4. ESMS (M+H): m/z 243.
Oximes (7 and 9). Prepared and characterized according to literature methods.25,26
3-o-Hydroxyaryl-1,2-benzisoxazoles (10-22). Prepared by analogy to literature methods.10,11
3-[2′-Hydroxyphenyl]-1,2-benzisoxazole (10). Isolated as oil. Yield 76%, Rf = 0.86. IR (KBr): 3280, 1615 cm-1. 1H NMR (400 MHz, CDCl3): δ 6.93-8.27 (m, 8H aromatic), 9.73 (s, 1H, OH). 13C NMR (100 MHz, CDCl3): δ 155.7, 153.1, 136.4, 135.3, 131.4, 128.3, 123.0, 121.9, 121.2, 118.7, 116.8, 111.9. ESMS (M+1): m/z 212. Anal. Calcd for C3H9NO2 C, 73.93; H, 4.26; N, 6.63. Found: C, 73.79; H, 4.10; N, 6.40%.
3-[2′-Methoxyphenyl]-1,2-benzisoxazole (11). Isolated as oil. Yield 48%, Rf = 0.78. IR (KBr): 1610 cm-1. 1H NMR (400 MHz, CDCl3): δ 6.81-8.12 (m, 8H, aromatic), 3.81 (s, 3H, OCH3). 13C NMR (100 MHz, CDCl3): δ 155.4, 153.3, 135.8, 133.1, 133.4, 128.4, 123.1, 121.6, 121.2, 119.0, 116.6, 114.4, 111.8, 57.4. ESMS (M+1): m/z 226.
3-[2′-Ethoxyphenyl]-1,2-benzisoxazole (12). Isolated as oil. Rf = 0.72. 1H NMR (400 MHz, CDCl3): δ 6.80-8.14 (m, 8H, aromatic), 4.12 (q, 2H, OCH2CH3), 1.30 (t, 3H, OCH2CH3). 13C NMR (100 MHZ CDCl3): δ 155.3, 152.8, 135.6, 133.4, 133.1, 128.4, 123.1, 121.6, 121.2, 119.0, 116.6, 114.4, 111.8, 61.0, 42.4. ESMS (M+H): m/z 240.
3-[2′-Hydroxyaryl]-1,2-benzisoxazoles (13-15, 19-21). Not isolated to their individual isomers. Identified by their 1H NMR spectra compared with those of the other derivatives and relevant lit. data.23,32
3-[2′-Hydroxy-3′-methylphenyl]-7-methyl-1,2-benzisoxazole (16). IR: 3360, 1600cm1 1H NMR: 7.40-7.65 (m, 3H), 7.25 (dd, J = 8.20, 7.50, 1.30 Hz, 1H), 7.10 (dd, J = 8.0 Hz, J = 1.35 Hz, J = 1.10 Hz, 1H), 6.85 (dd, J = 8.10 Hz, J = 7.30 Hz, J = 1.20 Hz, 1H), 6.75 (dd, J = 8.20 Hz, J = 1.30 Hz, J = 0.90 Hz, 1H), 2.35 (s, 3H, Me), 2.15 (s, 3H, Me). 13C NMR (100 MHz, CDCl3): δ 152.6, 148.8, 128.4, 126.0, 125.4, 124.8, 120.8, 120.0, 119.5, 116.0, 110.0, 108.1, 102.4, 43.5. 42.5. ESMS (M+H): m/z 240. Anal. Calcd for C15H13NO2: C, 75.31; H, 5.43; N, 5.85. Found: C, 75.05; H, 5.18; N, 5.60%.
3-[2′-Hydroxy-4′bromophenyl]-5-bromo-1,2-benzisoxazoles (17). Viscous oil. Yield 72%. ΙR: 3275, 1637 cm-1. 1H NMR (400 MHz, CDCl3): δ 8.50 (s, 1H), 7.70-7.65 (m, 2H), 7.55-7.50 (m, 2H), 7.45-7.40 (d, J = 11 Hz, 1H), 7.35-7.25 (d, J = 11 Hz, 1H), 7.28-7.18 (d, J = 12 Hz, 1H). 13C NMR (100 MHz, CDCl3): δ 162.5, 153.3, 147.7, 134.5, 125.5, 124.9, 122.6, 122.4, 119.6, 118.8, 116.5, 108.5, 81. ESMS (M+H): m/z 291. Anal. Calcd for C13H7Br2NO2: C, 42.27; H, 1.89; N, 3.79. Found: C, 42.05; H, 1.72; N, 3.64%.
3-[2′Hydroxy-4′phenyl]-5-phenyl-1,2-benzisoxazoles (18). Isolated as an off-white powder. Yield 69%. Mp 118-119 oC, Rf = 0.84. IR (KBr): 3270, 1610 cm-1. 1H NMR (400 MHz, CDCl 3): δ 7.90-8.10 (m, 3H), 7.30-7.65 (m, 10H), 6.70-6.85 (m, 3H), 9.70 (s, 1H). 13C NMR (100 MHz, CDCl3): δ 155.5, 155.3, 153.1, 152.4, 150.6, 149.8, 141.9, 139.8, 135.4, 131.6, 130.8, 128.3, 128.1, 127.3, 124.1, 121.8, 118.8, 118.3, 116.4, 115.8, 114.4, 114.1, 110.8, 110.2. ESMS (M+1): m/z 364. Anal. Calcd for C25H17NO2: C, 82.64; H, 4.68; N, 3.85. Found: C, 82.48; H, 4.52; N, 3.60%.
3-[2′-Hydroxyphenyl]-5-nitro-1,2-benzisoxazole (22). Isolated as pale yellow flakes. Yield 59%. Mp 131-132 oC, Rf = 0.69. IR (KBr): 1620, 1505, 1470, 1330 cm-1. 1H NMR (400 MHz, CDCl3): δ 8.15-8.74 (m, 3H’s, J = 8.9 Hz, J = 3.1 Hz, J = 0.8 Hz), 6.90-7.90 (m, 4H) 9.70 (s, 1H). 13C NMR (100 MHz, CDCl3): δ 162.6, 155.4, 153.3, 135.4, 133.2, 131.1, 130.4, 124.2, 122.4, 121.6, 118.8, 116.6, 114.4. ESMS (M+1): m/z 257. Anal. Calcd for C13H8N2O4: C, 60.93; H, 3.12; N, 10.93. Found: C, 60.79; H, 3.0; N, 10.69%.
3-[2-Hydroxyaryl]-1,2benzisoxazole 2-oxides (23-34). Prepared by analogy to literature methods.25,26
3-[2′-Hydroxyphenyl]-1,2-benzisoxazoles 2-oxide (23). Isolated as an off-white powder. Yield 57%. Mp 111-112 oC, Rf = 0.71. IR (KBr): 3460, 1605, 1220 cm-1. 1H NMR (600 MHz, CDCl3): δ 7.82-8.05 (m, 4H), 7.30-7.70 (m, 4H, J = 8.6 Hz, J = 2.9 Hz, J = 0.7 Hz), 8.20 (s,1H). 13C NMR (100 MHz, CDCl3): δ 153.3, 150.6, 136.4, 135.1, 131.3, 128.3, 124.1, 123.3, 121.0 120.3, 120.0, 117.5, 116.4. ESMS (M+1): m/z 228. Anal. Calcd for C13H9NO3: C, 68.72; H, 3.96; N, 6.16. Found: C, 68.50; H, 3.80; N, 5.90%.
3-[2′Methoxyphenyl]-1,2-benzisoxazole 2-oxide (24). Isolated as a viscous oil. Yield 54%, Rf = 0.82. IR (KBr): 1600, 1215 cm-1. 1H NMR (600 MHz, CDCl3): δ 7.70-7.95 (m, 4H), 7.30-7.60 (m, 4H), 3.80 (s, 3H, OMe). 13C NMR (100 MHz, CDCl3): δ 152.9, 150.2, 136.6, 134.8, 131.0, 128.0, 124.4, 123.1, 121.1, 119.8, 118.1, 116.9, 116.3, 57.6. ESMS (M+1): m/z 242. Anal. Calcd for C14H11NO3: C, 69.70; H, 4.56; N, 5.80. Found: C, 69.52; H, 4.40; N, 5.52%.
3-[2′-Ethoxyphenyl]-1,2-benzisoxazole 2-oxide (25). Isolated as a viscous oil. 1H NMR (400 MHZ, CDCl3): δ 7.70-7.90 (m, 4H, aromatic), 7.30-7.60 (m, 4H, aromatic), 4.0 (q, 2H, OCH2CH3), 1.30 (t, 3H, OCH2CH3). 13C NMR (100 MHZ, CDCl3): δ 152.6, 150.4, 136.6, 134.8, 131.2, 128.2, 124.4, 123.4, 121.4, 119.6, 118.2, 116.8, 116.4, 59.2, 41.8. ESMS (M+H): m/z 256.
3-[2′Hydroxyphenyl]-1,2-benzisoxazole 2-oxides (26-28, 31-33). Not isolated to their individual isomers. Identified by their 1HNMR spectra compared with those of the other derivatives and relevant lit. data25,26,32
3-[2′-Hydroxy-3′-methylphenyl]-1,2-benzisoxazole 2-oxide (28). IR: 3420, 1615, 1590, 1215cm-1. 1H NMR (400 MHZ, CDCl3): δ 7.25-7.60 (m, 3H, aromatic), 7.20 (dd, J = 8.29, J = 7.46 Hz, J = 1.27 Hz, 1H), 7.10 (dd, J = 8.01 Hz, J = 1.27 Hz, J = 0.99 Hz, 1H), 6.80 (dd, J = 8.01 Hz, J = 7.40 Hz, J = 1.22 Hz, 1H), 6.69 (dd, J = 8.19 Hz, J = 1.20 Hz, J = 0.90 Hz, 1H), 2.38 (s, 3H, Me), 2.14 (s, 3H, Me). 13C NMR (100 MHz, CDCl3): δ 151.4, 149.6, 128.4, 127.1, 126.2, 123.6, 120.7, 119.4, 118.8, 114.1, 109.4, 108.0, 102.4, 104.4, 43.6, 42.2. ESMS (M+H): m/z 242. Anal. Calcd for C14H11NO3: C, 69.70; H, 4.56; N, 5.80. Found: C, 69.48; H, 4.39; N, 5.52 %.
3-[2-′Hydroxy-4′-bromophenyl]-5-bromo-1,2-benzisoxazole 2-oxide (29). Isolated as yellowish microcrystals. Yield 64%. Mp 193 oC, Rf = 0,75. IR: 3439, 1615, 1215 cm-1. 1H NMR (400 MHz, CDCl3): δ 8.23 (s, 1H), 7.69 (d, J = 2.4 Hz, 1H), 7.64 (d, J = 1.9 Hz, 1H), 7.58 (dd, J = 8.8, 1.9 Hz, 1H), 7.42 (dd, J = 8.5, 2.4 Hz, 1H), 7.14 (d, J = 8.8 Hz, 1H), 7.00 (d, J = 8.5 Hz, 1H). 13C NMR (100 MHz, CDCl3): δ 156.7, 148.7, 134.6, 132.2, 131.2, 128.1, 125.5, 123.4, 122.4, 118.7, 116.2, 110.8, 108.5. ESMS (M+H): m/z 386. Anal. Calcd for C13H7Br2NO3: C, 40.51; H, 1.81; N, 3.63. Found: C, 40.30; H, 1.70; N, 3.45%.
3-[2-′Hydroxy-4′-phenyl]-5-phenyl-1,2 Benzisoxazole 2-oxide (30). Isolated as amorphous solid. Yield 60%. Mp 151 oC, Rf = 0.79. IR (KBr): 3430, 1610, 1220 cm-1. 1H NMR (400 MHz, DMSO-d6): δ 7.75-7.95 (m, 3H), 7.30-7.60 (m, 10H), 6.70-6.85 (m, 3H), 8.25 (s, 1H). 13C NMR (100 MHz, DMSO-d6): δ 155.3, 155.0, 153.1, 152.4, 150.4, 148.1, 145.5, 141.9, 138.5, 135.4, 131.5, 130.8, 128.3, 128.0, 126.6, 124.1, 121.6, 118.8, 118.3, 116.1, 114.8, 114.4, 114.0, 110.8, 110.1. ESMS (M+1): m/z 380. Anal. Calcd for C25H17NO3: C, 79.15; H, 4.48; N, 3.69. Found: C, 78.95; H, 4.26; N, 3.44%.
3-[2′-Hydroxyphenyl]-5-nitro-1,2-benzisoxazole 2-oxide (34). Isolated as pale yellow microcrystals. Yield 52%. Mp 162-163 oC, Rf = 0.68. IR (KBr): 1615, 1220 cm-1. 1H NMR (400 MHz, CDCl3-DMSO-d6): δ 7.2-8.45 (m, 3H,) 7.0-7.60 (m, 4H), 8.20 (s,1H). 13C NMR (400 MHz, CDCl3-DMSO-d6) δ: 159.4, 153.4, 151.1, 144.0, 135.4, 133.2, 131.6, 125.4, 123.1, 121.4, 120.1, 119.4, 117.5. ESMS (M+1): m/z 273. Anal. Calcd for C13H8N2O5: C, 57.35; H, 2.94; N, 10.29. Found: C, 57.15; H, 2.76; N, 10.08%.
References
1. P. Conti, C. Dallanoce, M. De Amici, C. De Micheli, and K.-N. Klotz, Bioorg. Med. Chem., 1998, 6, 401; CrossRef D.-H. Ko, M. F. Maponya, M. A. Khalili, E. T. Oriaku, Z. You, and H. J. Lee, J. Med. Chem., 1998, 8, 313; A. R. Katritzky, S. Wang, M. Zhang, and P. J. Voronkov, J. Org. Chem., 2001, 66, 6787; CrossRef M. Lautens and A. Roy, Org. Lett., 2000, 2, 555; CrossRef D. Giomi, F. M. Cordero, and F. Machetti, Comprehensive Heterocyclic Chemistry III, Vol 4, Ed, by A. R. Katritzky, C. A. Ramsden, E. F. V. Scriven, and R. J. K. Taylor, 2008, p. 365.
2. D. P. J. Curran, J. Am. Chem. Soc., 1983, 105, 5826. CrossRef
3. B. H. Kim, Y. J. Chung, and E. J. Ryu, Tetrahedron Lett., 1993, 34, 8465. CrossRef
4. A. P. Kozikowski and P. D. Stein, J. Am. Chem. Soc., 1982, 104, 4023. CrossRef
5. D. P. Curran and B. H. Kim, Synthesis, 1986, 312. CrossRef
6. N. Maugein, A. Wagner, and C. Mioskowski, Tetrahedron Lett., 1997, 38, 1547. CrossRef
7. G. A. Lee, Synth. Commun., 1982, 12, 508.
8. U. S. Sørensen, E. Falch, and P. Krogsgaard-Larsen, J. Org. Chem., 2000, 65, 1003. CrossRef
9. M. A. P. Martin, A. F. C. Flores, G. P. Bastos, A. Sinhorin, H. G. Bonacorso, and N. Zanatta, Tetrahedron Lett., 2000, 41, 293. CrossRef
10. N. Iranpoor, H. Firouzabadi, and N. Nowrouzi, Tetrahedron Lett., 2006, 47, 8247. CrossRef
11. T. J. Dale, A. C. Sather, and J. Rebek, Jr., Tetrahedron Lett., 2009, 50, 6173. CrossRef
12. K. F. Shelka, S. B. Sapkal, N. V. Shitole, B. B. Shingata, and M. S. Shingare, Org. Commun., 2009, 2, 72.
13. S. Udd, R. Jokela, R. Franzén, and J. Tois, Tetrahedron Lett., 2010, 51, 1030. CrossRef
14. A. V. Dubrovskiy and R. C. Larock, Org. Lett., 2010, 12, 1180. CrossRef
15. E. Comanita, I. Popovici, G. Roman, G. Robertson, and B. Comanita, Heterocycles, 1999, 51, 2139. CrossRef
16. H. Suh, S. Jeong, Y. N. Han, H. Lee, and J. Ryu, Biorg. Med. Chem. Lett., 1997, 7, 389. CrossRef
17. A. Nuhrich, M. Varache-Lembege, J. Vercauteren, R. Dokhan, P. Renard, and G. Devaux, Eur. J. Med. Chem., 1996, 31, 957. CrossRef
18. A. Villalobos, J. E. Blake, C. K. Biggers, T. W. Butler, D. S. Chapin, Y. L. Chen, J. L. Ives, S. B. Jones, D. R. Liston, A. A. Nagel, D. M. Nason, J. A. Nielsen, I.A. Shalaby, and W. F. White, J. Med. Chem., 1994, 37, 2721. CrossRef
19. J. T. Strupczewski, K. J Bordeau, Y. Chiang, E. J. Glamkowski, P. G. Conway, R. Corbett, H. B. Hartman, M. R. Szewczak, C. A. Wilmot, and G. C Helsey, J. Med. Chem., 1995, 38, 1119; CrossRef N. J. Hrib, J. G. Jurcak, K. L. Burgher, P. G. Conway, H. B. Hartman, L. L. Kerman, J. E. Roehr, and A. T. Woods, J. Med. Chem., 1994, 37, 2308. CrossRef
20. A. R Katritzky, S. A. Belyakov, Y. Fang, and J. S. Kiely, Tetrahedron Lett., 1998, 39, 8051; CrossRef H. V. Meyers, G. J. Dilley, T. L. Durgin, T. S. Powers, N. A. Winssinger, H. Zhu, and M. R. Pavia, Molec. Diversity, 1995, 1, 13. CrossRef
21. Angiotensin(II) increases the activity of oxidative enzymes, mainly NAD(P)H oxidase, causing injury to vascular endothelium, thus, various diseases (see: H. Mollnau, M. Wendt, K. Szöcs, B. Lasségue, E. Schulz, M. Oelze, H. Li, M. Bodenschatz, M. August, A. L. Kleshy, N. Tsilimingas, V. Walter, V. Försterman, T. Meinertz, K.Griendling, and T. Münzel, Circ. Res., 2002, 90, E58; CrossRef E. M. Mervada, Z. J. Cheng, I. TikkanEn, R. Lapatto, K. Nurminen, H. Vapaatalo, D. N. Muller, A. Fiebeler, V. Ganten, D. Ganten, and F. C. Luft, Hypertension, 2001, 37, 414).
22. M. Odrowaz-Sypniewski, P. G. Tsoungas, G. Varvounis, and P. Cordopatis, Tetrahedron Lett., 2009, 50, 5981. CrossRef
23. M. Pickert and A. W. Frahm, Arch. Pharm. Med. Chem., 1998, 331, 177; CrossRef W. G. A. Ibrom and A. W. Frahm, Arzeim Forsch./Drug. Res., 1997, 47, 662; A. A. Goldberg and H. A. Walker, J. Chem. Soc., 1953, 1349; S. N. Dhar, Ibid., 1920, 117, 1057.
24. B. Reese, Q. Song, and H. Yan, Tetrahedron Lett., 2001, 42, 1789; CrossRef C. B. Reese and H. Yan, J. Chem. Soc., Perkin Trans. 1, 2001, 1807. CrossRef
25. A. J. Boulton and P. G. Tsoungas, J. Chem. Soc., Chem. Commun., 1980, 421; CrossRef A. J. Boulton, P. G. Tsoungas, and C. Tsiamis, J. Chem. Soc., Perkin Trans. 1, 1986, 1665. CrossRef
26. R. M. Moriarty, B. A. Berglund, and M. S. C. Rao, Synthesis, 1993, 318; CrossRef P. Supsana, P. G. Tsoungas, and G. Varvounis, Tetrahedron, 2001, 57, 3445; CrossRef A. Kotali, I. S. Lafazanis, and P. A. Harris, Molbank, 2008, M572; CrossRef A. Kotali, Ibid., 2008, M573. CrossRef
27. The 2D COSY and a 2D NOESY 1H NMR spectra (in DMSO-d6 at 25 °C) of 7α show that the deshielded C-13 OH (δ=11.6 ppm) and NOH (δ=11.4 ppm) protons form intramolecular H-bonding in the Z-configuration.
28. Electrophilic Substitution on the rings to provide entries for further transformations, cannot be performed selectively on any of the structures 6-34.
29. Cyclization involving the p-NO2 bearing phenol is feasible.25 In the present case, however, it is outrun by the easier alternative cyclization mode.
30. Molecular modeling analysis was performed with Macromodel (Schrödinger: http://www.schrodinger.com) software and OPLS_2005 force field. Dielectric constant (ε) was set to 4.8 to simulate CDCl3 solvent used in NMR experiments. The first step in the conformational analysis was to construct a preliminary 3D model which was geometry optimized and was then subjected to Conformational Search (Random Sampling) using mixed torsional/low mode sampling with 5000 as maximum number of steps. Each one of the 64 derived conformers was energy minimized using Truncated Newton Conjugate Gradient (TNCG) algorithm with 5000 maximum iterations and converge on gradient with 0.001 threshold.
31. (a) A. Kumar and D. Kumar, ARKIVOC, 2007, (xiv), 117; (b) W.-H. Chen and Y. Pang, Tetrahedron Lett., 2009, 50, 6680; CrossRef (c) W.-H. Chen and Y. Pang, Ibid., 2010, 51, 1914. CrossRef
32. P. G. Tsoungas and B. De Costa, Magn. Res. Chem., 1988, 26, 8. CrossRef