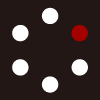
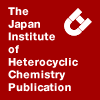
HETEROCYCLES
An International Journal for Reviews and Communications in Heterocyclic ChemistryWeb Edition ISSN: 1881-0942
Published online by The Japan Institute of Heterocyclic Chemistry
e-Journal
Full Text HTML
Received, 7th February, 2011, Accepted, 6th April, 2011, Published online, 8th April, 2011.
DOI: 10.3987/REV-11-701
■ Synthetic Routes towards Pyrimido[1,2-a][1,3,5]triazines (Review)
Anton V. Dolzhenko*
School of Pharmacy, Curtin University of Technology, GPO Box U1987, Perth, Western Australia 6845, Australia
Abstract
The present review summarizes information on the synthetic approaches to compounds with pyrimido[1,2-a][1,3,5]triazines, 1,3,5-triazino[2,1-b]quinazolines (benzofused pyrimido[1,2-a][1,3,5]triazines), and other polyfused heterocyclic systems bearing these scaffolds. Data concerning potential applications of the pyrimido[1,2-a][1,3,5]triazines, particularly as biologically active agents, are also discussed.CONTENTS
1. INTRODUCTION
2. SYNTHESIS OF PYRIMIDO[1,2-a][1,3,5]TRIAZINES BY ANNELATION OF THE 1,3,5-TRIAZINE RING ONTO A PYRIMIDINE SCAFFOLD.
2.1. Synthesis of pyrimido[1,2-a][1,3,5]triazines using Mannich condensation.
2.2. Synthesis of pyrimido[1,2-a][1,3,5]triazines using multicomponent reactions of pyrimidine derivatives with heterocumulenes.
2.3. Synthesis of pyrimido[1,2-a][1,3,5]triazines via reactions of 2-aminopyrimidines with bielectrophilic C-N-C triatomic synthons.
2.4. Synthesis of pyrimido[1,2-a][1,3,5]triazines via 1,3,5-triazine ring annelation on 2-substituted pyrimidines using one-carbon inserting reagents.
2.5. Synthesis of pyrimido[1,2-a][1,3,5]triazines via intramolecular cyclization of substituted pyrimidines.
3. SYNTHESIS OF PYRIMIDO[1,2-a][1,3,5]TRIAZINES BY ANNELATION OF THE PYRIMIDINE RING ONTO A 1,3,5-TRIAZINE SCAFFOLD.
3.1. Synthesis of 1,3,5-triazino[2,1-b]quinazolines (benzofused pyrimido[1,2-a][1,3,5]triazines) via reactions of chloro substituted 1,3,5-triazines with anthranilic acid derivatives.
3.2. Synthesis of pyrimido[1,2-a][1,3,5]triazines via reactions of 2-amino-1,3,5-triazines with bielectrophilic C-C-C triatomic synthons.
3.3. Synthesis of pyrimido[1,2-a][1,3,5]triazines via pyrimidine ring annelation on 2-substituted 1,3,5-triazines using one-carbon inserting reagents.
3.4. Synthesis of pyrimido[1,2-a][1,3,5]triazines via intramolecular cyclization of substituted 1,3,5-triazines.
4. SYNTHESIS OF PYRIMIDO[1,2-a][1,3,5]TRIAZINES VIA FORMATION OF PYRIMIDINE AND 1,3,5-TRIAZINE RINGS IN ONE REACTION.
5. REARRANGEMENTS IN PYRIMIDO[1,2-a][1,3,5]TRIAZINES WITH RECYCLIZATION OF THE SYSTEM.
6. CONCLUSION
REFERENCES
1. INTRODUCTION
In continuation of the series of reviews on fused 1,3,5-triazines,1 the present review focuses on existing methods of the synthesis of compounds comprising pyrimido[1,2-a][1,3,5]triazine nucleus (Figure 1) as well as their biological activity and applications.
The first publication on the synthesis of pyrimido[1,2-a][1,3,5]triazine derivative appeared 50 years ago.2 Ziegler and Nölken2 reported the reaction of 2,4-diamino-1,3-5-triazine (1) with bis-(2,4-dichlorophenol) ester of 3-benzylmalonic acid (2) that afforded pyrimido[1,2-a][1,3,5]triazine 3 (Scheme 1). However, they were not able to differentiate between two possible regioisomeric structures with amino group in position 2 or 4.
Until now, a number of effective synthetic procedures has been developed for the preparation of diversely substituted pyrimido[1,2-a][1,3,5]triazines and their polyfused analogues. During last decade, an interest has been developed towards the biological activity investigation of compounds with the pyrimido[1,2-a][1,3,5]triazine heterocyclic core. The attempted herein systematization of the available synthetic methods for the preparation of these compounds aims to facilitate further investigation in this emerging field of research.
The general methods of synthesis of pyrimido[1,2-a][1,3,5]triazines can be categorized into: (1) annelation of the 1,3,5-triazine ring onto a pyrimidine scaffold, (2) annelation of the pyrimidine ring onto a 1,3,5-triazine scaffold, (3) formation of both pyrimidine and 1,3,5-triazine rings in the same reaction, and (4) rearrangements in pyrimido[1,2-a][1,3,5]triazines with recyclization of the system.
2. SYNTHESIS OF PYRIMIDO[1,2-a][1,3,5]TRIAZINES BY ANNELATION OF THE 1,3,5-TRIAZINE RING ONTO A PYRIMIDINE SCAFFOLD
The annelation of the 1,3,5-triazine ring onto a pyrimidine scaffold is the most explored and developed approach for the synthesis of pyrimido[1,2-a][1,3,5]triazines and their polyfused analogues. This section of the review is further subdivided on the basis of the reaction type and structure of the building blocks used in this approach.
2.1. Synthesis of pyrimido[1,2-a][1,3,5]triazines using Mannich condensation
The synthesis of pyrimido[1,2-a][1,3,5]triazines 5 was achieved via Mannich condensation of 2-ethylaminopyrimidine 4 with formaldehyde and variety of amines (Scheme 2).3 The products were claimed as fungicides against plant-pathogenic fungi.
The reaction of 5,5-diethyl substituted 2-imino analogues of barbituric acid 6 with formaldehyde and aliphatic amines was reported to result in the 1,3,5-triazine ring formation providing 7 (Scheme 3).4,5 Similar reaction was performed using condensation of formaldehyde with 6 and a variety of anilines (Scheme 4). The yields of pyrimido[1,2-a][1,3,5]triazines 8 varied from 6 to 77% depending on the structure of the anilines used for the reaction.6 The pharmacological screening of 8 led to the identification of one compound (R = Et, Ar = 2-ClC6H4) with antifungal activity against Microsporum canis (10-6 < IC50 < 10-5 M) and another one (R = n-Bu, Ar = 4-CF3C6H4) with average affinity for 5-HT1A and 5-HT2B receptors (10-8 < IC50 < 10-7 M).
The formation of two 1,3,5-triazine rings were observed when pyrimidine 9 possessing two imino groups underwent Mannich condensation with formaldehyde and amines (Scheme 5).4,5 Tricyclic products 10 underwent biological investigation; 10d and 10e showed some anthelmintic activity against Nippostrongylus brasiliensis.5
The reactions of nucleic bases with formaldehyde in presence of amines have been a subject of extensive explorations. Mannich condensation of different guanine derivatives were studied in various conditions. Treatment of 9-methylguanine (11a) with formaldehyde and methylamine provided 12 as a product of the 1,3,5-triazine ring annelation and hydroxymetylation (Scheme 6).7
Takahashi and Hashimoto8 reported synthesis of Mannich bases 13 using deoxyguanosine (11b), formaldehyde and butylamine or aniline (Scheme 7). This reaction was suggested as a possible model of the modification of DNA with carcinogenic amines in the formaldehyde presence.
To get a better understanding of carcinogenic and mutagenic properties of formaldehyde itself, the formaldehyde-induced cross-linking of deoxynucleosides with amino acids was investigated.9 Mannich bases 15 and 16 were isolated together with another cross-linked product 14 from the reaction of deoxyguanosine (11b), formaldehyde and protected lysine (Scheme 8). The similar behavior was also observed for peptides containing lysine and trinucleotides containing deoxyguanosine (11b).
The treatment of guanosine (11c) with formaldehyde in alkaline conditions resulted in the formation of N2-hydroxymethylguanosine (17), bis-(N2-guanosyl)methane (18), and tricyclic product 19 (Scheme 9).10 For the structure assignments, 13C- and 15N-labelled 19 were also prepared using [13C]formaldehyde and [15N]guanosine. This reaction pathway was proposed to contribute to the genotoxic properties of formaldehyde.
2.2. Synthesis of pyrimido[1,2-a][1,3,5]triazines using multicomponent reactions of pyrimidine derivatives with heterocumulenes
Several types of reactions with different mechanisms involved in the 1,3,5-triazine ring annelation process were reported for pyrimidine derivatives and isocyanates (most commonly used heterocumulenes). The key element of these reactions is cycloaddition, which may be accompanied by other processes depending on the substrate structure and the reaction conditions. The most explored approaches include: [2+2+2] cycloaddition of two iso(thio)cyanate molecules to substituted pyrimidine, reactions of pyrimidines having a leaving group in position 2, and reactions of 2-aminopyrimidines or their derivatives with heterocumulenes.
4-Pyrimidinone (20) in the reaction with methyl isocyanate in presence of base afforded mixture of 1:2-cycloadduct 21 and its methylcarbamoyl derivative 22 (Scheme 10).11 Substantial amount (45%) of unreacted 20 was also recovered.
Similarly, cycloadduct 25 was the main product of the reaction of 4-quinazolinone (23) with methyl isocyanate (Scheme 11).11 Adducts 24 and 26 with 52% of unreacted 23 were also isolated from the reaction mixture.
Under mild conditions, treatment of 3,4-dihydroquinazolines 27 with phenyl isocyanate led to the [2+2+2] cycloaddition providing 28 (Scheme 12).12 The structure of the product was supported by X-ray crystallographic data for 28b. Using higher temperatures in this reaction resulted in the formation of more complex cycloadducts.
Richter13 found that reaction of cyclic amidine 29 with phenyl and 4-chlorophenyl isocyanates in benzene under mild conditions afforded 30f and 30i, respectively (Scheme 13). Later, Arya and Shenoy14 extended scope of this reaction for the synthesis of 30 applying isocyanates of diverse structure. Using DMF as a reaction media was also reported15 for the preparation of 30a.
Analogous synthesis of 32 was performed by treatment of amidine 31 with isocyanates in DMF (for 32a-c)15 or benzene (for 32d-j)14 (Scheme 14). These reactions were used in the invention16 of heat-resistant coating and molding materials.
The cycloaddition of two molecules of methyl isothiocyanate to 2-methyl-1,4,5,6-tetrahydropyrimidines (33) resulted in the formation of pyrimido[1,2-a][1,3,5]triazines 34 (Scheme 15).17 The reaction time was increased from 1-3 h for the synthesis of 34a,b to 2 days for the preparation of phenyl substituted 34c. The later was also obtained in 38% yield using chloroform as a solvent.
Similar reaction of cyclic amidine 31 with methyl isothiocyanate afforded 35 (Scheme 16).15
The reaction of 2-benzylthio-1,4,5,6-tetrahydropyrimidine (36) with phenyl isocyanate at ambient temperature led to the formation of pyrimido[1,2-a][1,3,5]triazin-2,4-dione 37a (Scheme 17).18 The benzylthio group served as a leaving group in this reaction. Similarly, a variety of isothiocyanates was successfully applied for the preparation of 37b-f.18-20 Using excess of isothiocyanates significantly improved yields of the products. However, attempts to prepare 37f in benzene failed and despite excess of the isothiocyanate, only 5% of the product was isolated from the reaction in DMF.20
Dianion 39, generated from hexahydropyrimidin-2-thione (38), reacted with alkyl isothiocyanates also providing pyrimido[1,2-a][1,3,5]triazines 37 as main products. Moreover, tricyclic compounds 40 with hypervalent sulfur in the structure were formed at the same time (Scheme 18).21 In case of the reaction of 39 with ethyl isothiocyanate, 1:1 adduct 41 was also isolated.
The treatment of 2-amino-1,4,5,6-tetrahydropyrimidine (42) with isocyanates was claimed22 to afford pyrimido[1,2-a][1,3,5]triazin-2,4-diones 43 possessing herbicidal properties (Scheme 19).
Heating N,N-dimethyl-N’-(pyrimidin-2-yl)formamidine (44) with excess of aryl isocyanates in benzene gave 3-arylpyrimido[1,2-a][1,3,5]triazin-2,4-diones (46) (Scheme 20).23 As a possible mechanistic explanation of the formation of 46, [4+2] cycloaddition of presumable intermediate pyrimidin-2-yl isocyanate (45) and aryl isocyanate molecule was suggested.
Pyrimidin-2-yl isocyanate (45) was also proposed as an intermediate in the reaction of pyrimidin-2-yliminotriphenylphosphorane (47) with phenyl isocyanate (Scheme 21).24
However, competing synthetic pathway involving another intermediate - carbodiimide 48 increased complexity of the process. The preferred pathway, structure and composition of products were found to strongly depend on the reaction conditions. At ambient temperature, two products 46a (minor) and 49 (major) were isolated from the reaction of 47 with excess of phenyl isocyanate. Their formation was explained via [4+2] cycloaddition of corresponding intermidiates 45 and 48 to the phenyl isocyanate molecule. Heating was found to reverse chemoselectivity of the process promoting formation of 46a. Using only slight excess of phenyl isocyanate or its equimolar quantity and prolong reaction time resulted in the isolation of the carbodiimide 48 dimerization product 50, mainly or even exclusively (when heating was applied). Interesting results were obtained in the hydrolysis study of 49. After initial pyrimidine ring opening, the product was further hydrolyzed in presence of acid with the triazine ring cleavage and the pyrimidine ring recyclization.25
The reaction of substituted 2-aminopyrimidin-4-one and its acyl derivatives 51 with phenyl isocyanate in presence of base was reported26 to provide pyrimido[1,2-a][1,3,5]triazin-2,4,6-trione 52 (Scheme 22).
Analogously, treatment of t-butyldimethylsilyl protected deoxyguanosine (11d) with phenyl isocyanate resulted in the 1,3,5-ring closure leading to tricyclic nucleoside 53 (Scheme 23).26
Another tricyclic 1,2,4-triazolo[1',5':3,4]pyrimido[1,2-a][1,3,5]triazine 55 was prepared in a similar manner from acetamide 54 and phenyl isocyanate (Scheme 24).27
The reaction of 47 with diphenylcarbodiimide provided 56 (Scheme 25).28 The proposed reaction mechanism involved initial formation of carbodiimide 48 followed by the cycloaddition of another diphenylcarbodiimide molecule. The polymerization, observed during the reaction, was a reason for the low isolated yield of 56.
It was reported that treatment of 2-chloropyrimidine 57 with ammonium thiocyanate, followed by the [4+2] cycloaddition of intermediate isothiocyanate 58 and phenyl isocyanate resulted in the formation of 2-thioxopyrimido[1,2-a][1,3,5]triazin-4-one 59 (Scheme 26).29 The regioselectivity of the cycloaddition step is questionable due to similar environment of the endocyclic pyrimidine nitrogen atoms of 57, but no alternative structure was discussed in the paper.29
2.3. Synthesis of pyrimido[1,2-a][1,3,5]triazines via reactions of 2-aminopyrimidines with bielectrophilic C-N-C triatomic synthons
This synthetic approach allows convenient preparation of a variety of functionalized pyrimido[1,2-a][1,3,5]triazines from readily available 2-aminopyrimidines. The careful selection of the C-N-C triatomic synthons provides an opportunity for annelation of the 1,3,5-triazine ring with desirable substitution pattern. However, regiochemistry of the reaction should be always considered. Two endocyclic and one exocyclic nitrogen atoms are nucleophilic centers of 2-aminopyrimidines competing for two electophilic centers of the C-N-C synthons. Small changes in the reagent structure or variations of the reaction conditions may result in the preparation of any of four possible in general case isomeric pyrimido[1,2-a][1,3,5]triazines or their mixture. The investigations of the reactions of methyl N-cyanoformimidate (61) with 2-aminopyrimidines by Leonard’s group30-34 demonstrated complexity of this regioselectivity issue. Moreover, small variations in the reaction parameters also changed dramatically the chemoselectivity of the process.
Two isomeric pyrimido[1,2-a][1,3,5]triazines 63 and 65 were obtained in the ratio 2:1 upon treatment of isocytosine (60) with methyl N-cyanoformimidate (61) in hexamethylphosphoramide (HMPA) at 50-55 °C (Scheme 27).30 The imidate group of 61 reacted with the exocyclic amino group of 60 followed by the 1,3,5-triazine ring closure to either of the endocyclic nitrogen atoms. However, the reaction products were different from expected amines 62 and 64. It was suggested that cyanamide, produced in the reaction via an alternative process, reacted with formed as intermediates 62 and 64 to give corresponding guanidines 63 and 65.
Changing the reaction conditions made the direction of the 1,3,5-triazine ring closure selective, but amino group of intermediate 64, formed after the initial annelation, underwent condensation with another molecule of 61 providing sodium salt 66 (Scheme 28).31 Using lesser quantities of imidate 61 did not change the product composition, but just decrease the yield of 66. The side chain of 66 was cleaved and structure of resulted 4-aminopyrimido[1,2-a][1,3,5]triazin-8-one (64) was determined using X-ray diffraction analysis of its triflate salt.
The opposite regiochemistry of the 1,3,5-triazine ring annelation was observed when 6-substituted isocytosine analogues 67 were treated with 61 (Scheme 29).31 Furthermore, even with a substantial excess of imidate 61 only amines 68 were isolated from the reaction.
Similarly, guanine nucleosides and their analogues (11) reacted with 61 affording fused tricyclic compounds 69 (Scheme 30).32-34 The structure of 69 was confirmed by X-ray diffraction analysis of 69c32,33 and deoxyguanosine derivative 69f.34 The N15 NMR spectroscopic study, determination of pKa, and assessment of hydrolytic stability of the glycosidic bond of guanosine derivative 69d were also performed.35
Isocyanates bearing at the nitrogen atom another reactive electrophilic group found an application as C-N-C triatomic synthons allowing annelation of 1,3,5-triazine ring with at least one carbonyl group. The nature of the electrophilic group attached to the isocyanate determines other types of substitutuents on the created triazine ring as well as governs regioselectivity of the ring closure.
Chlorocarbonyl isocyanate (71) is the most popular reagent for the annelation of 1,3,5-triazin-2,4-dione to 2-aminopyrimidines. Pyrimido[1,2-a][1,3,5]triazin-2,4-dione (72) was conveniently prepared by the treatment of 2-aminopyrimidine (70) with 71 in presence of base at ambient temperature (Scheme 31).36
Isocytosine (60) reacted with 71 affording pyrimido[1,2-a][1,3,5]triazin-2,4,8-trione (73) in 91% yield (Scheme 32).37 The product (73) was further alkylated and X-ray crystallographic study of methyl and benzyl derivatives 74 and 75 was used to confirm the regiochemistry of the ring closure.
Analogously, the 1,3,5-triazin-2,4-dione was annelated to 2-amino-4-phenylquinazolines (76) with the ring closure to N-1 of the quinazoline nucleus (Scheme 33).36 The theoretically possible regioisomeric structure, alternative to 77, was not mentioned.
Compound 79, prepared by the treatment of 78 with chlorocarbonyl isocyanate (71) (Scheme 34), was shown to possess central nervous stimulant activity comparable with penthylenetetrazole.38
Similarly, benzoyl protected guanosine 11e was smoothly converted to 80 (Scheme 35).39 However, deprotection attempts were unsuccessful and resulted in the triazine ring cleavage.
Greco and Gala40 reported the reaction of 2-alkylaminopyrimidines (81) with phenoxycarbonyl isocyanate (82) affording corresponding mesoionic 1-alkyl substituted pyrimido[1,2-a][1,3,5]triazin-2,4-diones (83) (Scheme 36).
The condensation of 4,6-diaryl substituted 2-aminopyrimidine 84 and aroyl isothiocyanate 85 (Scheme 37), in principle, might result in the formation of four regioisomeric pyrimido[1,2-a][1,3,5]triazines depending on the regioselectivity of the process. However, the isolation of one product 86 was reported41 with no conclusive evidence supporting this structure.
2.4. Synthesis of pyrimido[1,2-a][1,3,5]triazines via 1,3,5-triazine ring annelation on 2-substituted pyrimidines using one-carbon inserting reagents
This approach for the 1,3,5-triazine ring annelation was applied for pyrimidines with various functional groups including amidines, guanidines, ureas, and thioureas etc. Diverse choice of one-carbon inserting reagents opens an avenue for introducing different substituents (e.g. alkyl, aryl, hetaryl, amino, carbonyl, thiocarbonyl groups) into position 4 of the 1,3,5-triazine ring. The regioselectivity of the ring closure remains an important issue in some of the reactions utilizing this synthetic approach.
Pyrimido[1,2-a][1,3,5]triazines 89, claimed42 as GSK3-β inhibitors with potential for the treatment of neurodegenerative diseases, were prepared by the ring closure carbonylation of amidine 87 with phosgene followed by the alkylation of intermediate 88 (Scheme 38). However, no details of the synthetic procedure were provided.
The thermal cyclocondensation of guanidines 90 with aldehydes was used for the synthesis of 91 (Scheme 39).43,44 The X-ray diffraction study44 of 91 (R1 = CF3, R2 = 4-BrC6H4) proved the side of the ring closure.
Similarly, the synthesis of benzofused tricyclic analogues 93 was achieved via the 1,3,5-triazine ring annelation to the side b of quinazolinone 92 using a variety of aldehydes (Scheme 40).45
The same regiochemistry was observed in the synthesis of similar compounds 95 (Scheme 41).46 The base catalyzed cyclocondensation of 3,4-dihydroquinazolin-2-yl guanidine (94) with aldehydes resulted in the 1,3,5-ring closure at the N-3 atom of 94 that was confirmed by the 2D NOESY NMR spectral data of 95. Some of products 95 showed activity against lung (A549) and breast (MDA-MB-231) cancer cell lines. Thus, 95f demonstrated IC50 values of 17 and 15 µM against lung and breast cancer cells, respectively.
A variety of one-carbon inserting reagents were applied to construct appropriately substituted 1,3,5-triazine ring on the 3,4-dihydroquinazolin-2-yl guanidine (94) skeleton (Scheme 42).46 Gem-dimethyl substituted compound 96 was prepared using acetone as one-carbon inserting reagent. The condensations of 94 with diethyl ethoxymethylenemalonate or triethyl orthoformate afforded the same product 2-amino-6H-1,3,5-triazino[2,1-b]quinazoline (97). The 2,4-diamino-1,3,5-triazine ring was annelated to 94 via the reaction with trichloroacetoniltile providing 98. The synthesis of 99 was successfully achieved by the ring closure thiocarbonylation of 94 with carbon disulfide.
Unexpected product was obtained when guanidine 100 was treated with triethyl orthoacetate (Scheme 43).47 Instead of expected 2-amino-4,8-dimethylpyrimido[1,2-a][1,3,5]triazin-6-one (101), its 4-amino-2,8-dimethyl substituted isomer 102 was isolated. The formation of 102 can be rationalized by the rearrangement of 101 under the reaction condition via the pyrimidine ring opening of 101 and subsequent recyclization to another nitrogen atom of the 1,3,5-triazine nucleus. The strong intramolecular hydrogen bonding stabilizes rearranged product 102.
The thermolysis of ureas 103 was reported to afford pyrimido[1,2-a][1,3,5]triazin-2,4-diones 105 along with decarbamoylation products 106 (Scheme 44).48 Although the mechanism of the reaction was not investigated and discussed, it might be assumed that both products formed in the concerted process, presumably via the corresponding isocyanate 104 intermediacy.
The synthesis of 6,8-dimethyl-2-thioxopyrimido[1,2-a][1,3,5]triazin-4-one (108) was performed via the ring closure carbonylation of thiourea 107 using ethyl chloroformate (Scheme 45).49
In the condensation with triethyl orthoformate, the S-benzoyl substituted isothiourea 109, derived from 107, was reported49 to produce 6,8-dimethylpyrimido[1,2-a][1,3,5]triazin-2-thione (110) (Scheme 46). Both 108 and 110 demonstrated antibacterial activity against Klebsiella pneumoniae.
Several triethyl orthoesters were used as one-carbon inserting reagents for the construction of 1,3,5-triazine ring connecting pyrimidine and pyrazole rings of 111 and therefore converting 111 into tricyclic compounds 112 (Scheme 47).50
The treatment with phosgene was used to introduce a carbonyl group connecting pyrimidine and benzimidazole nitrogen atoms of 2-pyrimidin-2-ylaminobenzimidazole (113) (Scheme 48).51 Tetracyclic product 114 was formed in almost quantitative yield.
Pentaazacyclazine 116 was synthesized by the condensation of 115 with acetic anhydrate (Scheme 49).52 Two 1,3,5-triazine rings were annelated via the three-bond formation cyclization. Acetic anhydride supplied one carbon atom to the 1,3,5-triazine ring having a [1,2-a] junction with the pyrimidine nucleus.
2.5. Synthesis of pyrimido[1,2-a][1,3,5]triazines via intramolecular cyclization of substituted pyrimidines
The thermal cyclization of 117, prepared by the reaction of 2-aminopyrimidines 81 with ethoxycarbonyl isocyanate (cf. Scheme 36), resulted in the formation of mesoionic compounds 83 (Scheme 50).40
The addition of ethoxycarbonyl isothiocyanate to the endocyclic nitrogen atom of 2-aminopyrimidines 81 gave 118, which underwent cyclocondensation in presence of trifluoroacetic acid affording mesoionic compounds 119 (Scheme 51).40
A partial conversion of 120 to 121a was observed in the attempt of crystallization of 120 from methanol. The 1,3,5-triazine ring closure was successfully completed by short heating 120 in DMF (Scheme 52).53
The pyrimidine and 1,3,5-triazine ring closure with three-bond formation affording tetraazacyclazine 123 occurred when pyrimidine 122 was heated in diphenyl ether in the presence of tosylic acid (Scheme 53).54
The sublimation of 1,3,4,6,7-pentaazacycl[3.3.3]azine (125) was achieved as a result of short vacuum pyrolysis of 2,4-bis(N'-cyano-N-formamidino)pyrimidine (124) (Scheme 54).55 The ionization potential of 125 was assessed experimentally and calculated along with the electron distribution and reactivity indexes.
3. SYNTHESIS OF PYRIMIDO[1,2-a][1,3,5]TRIAZINES BY ANNELATION OF THE PYRIMIDINE RING ONTO A 1,3,5-TRIAZINE SCAFFOLD
The first synthesis of pyrimido[1,2-a][1,3,5]triazines was performed by the annelation of the pyrimidine ring to 2,4-diamino-1,3,5-triazine (1) (vide supra Scheme 1).2 Further exploration of this general approach resulted in the development of several effective synthetic methods summarized below.
3.1. Synthesis of 1,3,5-triazino[2,1-b]quinazolines (benzofused pyrimido[1,2-a][1,3,5]triazines) via reactions of chloro substituted 1,3,5-triazines with anthranilic acid derivatives
This group of reaction is based on the nucleophilic substitution of reactive chlorine atom on carbon atom of 1,3,5-triazines by amino group of anthranilic acid derivatives followed by the pyrimidine ring closure to the endocyclic nitrogen atom of the 1,3,5-triazine core. Thus, the reaction of anthranilonitrile (126) with 2-amino-4-chloro-6-phenyl-1,3,5-triazine (127) gave 1,3,5-triazino[2,1-b]quinazoline 128 (Scheme 55).53
The synthesis of 2,4-diamino-1,3,5-triazino[2,1-b]quinazolin-6-ones 131 was performed via the condensation of methyl anthranilate (129) and 2,4-diamino-6-chloro-1,3,5-triazines 130 (Scheme 56).53
The formation of 133 having 1,3,5-triazine ring fused with two quinazoline nuclei was claimed56 to take place when 4,6-dichloro-2-dimethylamino-1,3,5-triazine (132) was heated in anisole with two equivalents of methyl anthranilate (129) (Scheme 57). The reaction yield was 38%, however subsequent overnight heating of the reaction residue in acetic acid allowed additional isolation of 33% of 133 (cf. Scheme 69).
3.2. Synthesis of pyrimido[1,2-a][1,3,5]triazines via reactions of 2-amino-1,3,5-triazines with bielectrophilic C-C-C triatomic synthons
Similarly to the firs report on the synthesis of pyrimido[1,2-a][1,3,5]triazines (vide supra Scheme 1), all reactions described in this section utilized derivatives of malonic acid for the annelation of pyrimidine ring to 2-amino-1,3,5-triazines. The reaction of melamine (134, R1 = R2 = NH2) and its alkyl substituted derivatives with diethyl malonate was used for the preparation of 2,4-diamino-7H-pyrimido[1,2-a][1,3,5]triazin-6,8-diones 135 (Scheme 58), which were claimed57 to be useful as insecticides. The yield in case of 135 with R1 = NH2, R2 = NMe2 was good (93%); no data for other 135 were reported.
The fusion of 136 with 2,4,6-trichlorophenyl 2-alkylmalonates (137) at 180 °C resulted in the formation of mesoionic pyrimido[1,2-a][1,3,5]triazines 138 (Scheme 59).58 Although the annelation of pyrimidine ring might resulted from the ring closure to either of two nonequivalent endocyclic nitrogen atoms of triazines 136, regioselectivity of the process was not discussed and no unequivocal data excluding a structure regioisomeric to 138 were provided. Moreover, the observed low field shift of the amino group signals (12.62-12.73 ppm) of 138 can be attributed to intramolecular hydrogen bonding with neighboring carbonyl group in the isomeric pyrimido[1,2-a][1,3,5]triazine structure.
Tetracyclic pyrimido[1,2-a][1,3,5]triazines fused with benzimidazole nucleus, compounds 14059 and 14260 were synthesized via the annelation of pyrimidine ring to corresponding amino substituted 1,3,5-triazino[1,2-a]benzimidazoles 139 and 141 using diethyl ethoxymethylenemalonate (Schemes 60 and 61). The regioselectivity of the later reaction was assigned using a number of spectroscopic techniques and parameters.
3.3. Synthesis of pyrimido[1,2-a][1,3,5]triazines via pyrimidine ring annelation on 2-substituted 1,3,5-triazines using one-carbon inserting reagents
This type of the pyrimido[1,2-a][1,3,5]triazine synthesis is represented by the condensation of triazine 143 with phosphoryl chloride activated Michler's ketone affording 144 (Scheme 62) useful as blue dyes.61
3.4. Synthesis of pyrimido[1,2-a][1,3,5]triazines via intramolecular cyclization of substituted 1,3,5-triazines
The intramolecular cyclization of N2,N2-diallyl substituted melamine (145) upon treatment with trifluoroperacetic acid resulted in the tetrahydropyrimidine annelation providing 146 (Scheme 63).62 The analogues of 146 with alkyl group other than allyl were also claimed to be preparable by this method from the corresponding N2-alkyl-N2-allylmelamines. The central nervous system depressant activity was mentioned for 146, but no details were provided.
The base-induced isoxazolone ring opening of 147 followed by the intramolecular cyclization with the pyrimidine ring formation was reported to give 148 (Scheme 64).63 The rearrangement appeared to be general and proceed via similar mechanism64 for various related heterocyclic molecules with the isoxazolone moiety.
A number of intramolecular cyclizations based on the reactions of anthranilic acid derivatives with 1,3,5-triazine ring attached to the amino group has been described in the literature. This approach was efficiently used for the preparation of heterocyclic systems of different complexity. Thus, heating 149a above its melting point allowed preparation of 4-amino-2-phenyl-1,3,5-triazino[2,1-b]quinazolin-6-one (121a) (Scheme 65).53,65
More efficient synthesis of 121a and its analogues was achieved by the treatment of 149 with sodium hydrate in DMF (Scheme 66).53
In the attempt to convert the carboxylic group of anthranilic acid derivative 150 into the amide with tosylate of D-alanine benzyl ester, one of the methoxy groups of 150 underwent nucleophilic substitution while the activated carboxylic group of 150 participated in two processes: the 1,3,5-triazine ring closure affording tricyclic 151 and the expected amide formation providing 152 (Scheme 67).66
The cyclization of cyclazine 153 was achieved by heating in acetic anhydrate (Scheme 68).67 The reaction was found to give only one polycyclic product 154, which was distinguished from its possible regioisomer on the basis of spectral data.
The decarboxylation together with the intramolecular cyclization took place when triazine 155 was heated in acetic acid (Scheme 69). Pentacyclic 133 was obtained56 from the reaction along with 11% of the byproduct, to which structure 156 or 157 was proposed.68 The separation of 133 and 1,3,5-triazino[2,1-b]quinazoline (156 / 157) was performed using different solubility of the products.
The quinazoline and triazine rings were formed when triazine 158 was treated with pivaloyl chloride affording tetracyclic structure 159 (Scheme 70).69
4. SYNTHESIS OF PYRIMIDO[1,2-a][1,3,5]TRIAZINES VIA FORMATION OF PYRIMIDINE AND 1,3,5-TRIAZINE RINGS IN ONE REACTION
A few methods have been developed for the construction of both pyrimidine and 1,3,5-triazine rings of the pyrimido[1,2-a][1,3,5]triazine system in one reaction. The reaction of methyl anthranilate (129) with potassium N,N1-dicyanobenzamidine and its analogues (160) resulted in the formation of two new rings affording 2-substituted 4-amino-1,3,5-triazino[2,1-b]quinazolin-6-ones 121 (Scheme 71).53,65
Ceder and Vernmark70 reported annelation of the pyrimidine and 1,3,5-triazine rings to 2-amino-6-picoline (161) using condensation with ethyl N-cyanoformimidate (162) (Scheme 72). However, 1,3,4-triazacycl[3.3.3]azine (163) was isolated in very low yield.
The one-pot solid-phase synthesis of 168 having pyrimido[1,2-a][1,3,5]triazine core fused with benzimidazole ring was performed on p-methylbenzhydrylamine (MBHA) resin using Houghten’s “tea-bag” method71 (Scheme 73).72 Resin-bound 2-aminobenzimidazoles 164 were converted to their corresponding iminophosphoranes 165 followed by the treatment with 3-chloropropyl isocyanate. The annelation of 1,3,5-triazine ring occurred via the Aza-Wittig reaction concerted with the addition of the second isocyanate molecule to the endocylcic nitrogen atom of benzimidazole ring and the subsequent heterocyclization. The intramolecular nucleophilic substitution of chlorine atom at one of the side chains of 166 resulted in the pyrimidine ring closure affording 167. After cleavage from the resin, 168 were isolated as major regioisomers with 93-96% regioselectivity of the process and 80-85% purity of the products. The structure assignments were done on the basis of 2D NMR spectroscopy, particularly the HMBC data.
5. REARRANGEMENTS IN PYRIMIDO[1,2-a][1,3,5]TRIAZINES WITH RECYCLIZATION OF THE SYSTEM
Two rearrangements of pyrimido[1,2-a][1,3,5]triazines leading to pyrimido[1,2-a][1,3,5]triazines of different structure were reported.18,31 In the investigation of the hydrolysis of 37b, formation of 170 was observed after treatment of 37b with inorganic base (sodium acetate, sodium carbonate or sodium hydroxide) in water under mild conditions (0 °C) (Scheme 74).18 It was found that initial step of the reaction involved the hydrolytic pyrimidine ring opening followed by the recyclisation of triazine 169 via the intramolecular nucleophilic substitution of thioxo group. The structure of 170 was unambiguously established by X-ray crystallography.73
It was reported31 that 4-amino-8-methylpyrimido[1,2-a][1,3,5]triazin-6-one (68a) underwent thermally induced rearrangement to 4-amino-6-methylpyrimido[1,2-a][1,3,5]triazin-8-one (172) via intermediacy of carbodiimide 171, resulted from the triazine ring opening of 68a (Scheme 75). Heating 68a or 172 in aqueous DMF was reported to give formamidine 173 as a result of the hydrolytic 1,3,5-triazine ring opening. However, from the analysis of the provided 1H NMR spectral data, one can suggest an alternative pathway of the rearrangement with the corresponding structure corrections. For example, the ~1.2 ppm downfield shift of the methine proton signal on the triazine ring after the rearrangement should be encountered for the deshielding effect of the neighboring carbonyl group. Therefore, the structure of 2-amino-8-methylpyrimido[1,2-a][1,3,5]triazin-6-one (175), formed from ketene 174 via pyrimidine ring opening of 68a, can be proposed for the rearranged product (cf. Scheme 43). Similarly, hydrolytic cleavage of the pyrimido[1,2-a][1,3,5]triazine system of 68a was likely to proceed via the pyrimidine ring opening providing acid 176, theoretically more stable than formamidine 173. The spectral data also better correspond to structure 176 rather than 173. Further investigations are required for complete understanding of this interesting rearrangement.
6. CONCLUSION
A variety of effective synthetic approaches to the preparation of pyrimido[1,2-a][1,3,5]triazines and their polycyclic analogues has been developed. The biological activity of pyrimido[1,2-a][1,3,5]triazines has not been systematically studied. However, some reported biological activity data show a good potential for the future exploration in the medicinal and agricultural chemistry of pyrimido[1,2-a][1,3,5]triazines. The present review aims to set a background for the research in this area.
References
1. Part 4 in the series of reviews “Fused 1,3,5-triazines.” For Parts 1-3 see: A. V. Dolzhenko, A. V. Dolzhenko, and W. K. Chui, Heterocycles, 2006, 68, 1723; CrossRef A. V. Dolzhenko, A. V. Dolzhenko, and W. K. Chui, Heterocycles, 2008, 75, 1575; CrossRef A. V. Dolzhenko, Heterocycles, 2011, 83, 695. CrossRef
2. E. Ziegler and E. Nölken, Monatsh. Chem., 1961, 92, 1184. CrossRef
3. I. T. Kay, Pat. DE 2452893, 1975 (Chem. Abstr., 83, 97363).
4. M. G. Karmouta, O. Lafont, C. Combet-Farnoux, and M. Miocque, Compt. Rend., Ser. C: Sci. Chim., 1977, 285, 25.
5. M. G. Karmouta, O. Lafont, C. Combet-Farnoux, M. Miocque, M. C. Rigothier, B. Louchon, and P. Gayral, Eur. J. Med. Chem., 1980, 15, 341.
6. L. Lucry, F. Enoma, F. Estour, H. Oulyadi, S. Menager, and O. Lafont, J. Heterocycl. Chem., 2002, 39, 663. CrossRef
7. V. S. Volkov, A. M. Poverennyi, and E. D. Sverdlov, Bioorg. Khim., 1987, 13, 204.
8. H. Takahashi and Y. Hashimoto, Bioorg. Med. Chem. Lett., 2001, 11, 729. CrossRef
9. K. Lu, W. Ye, L. Zhou, L. B. Collins, X. Chen, A. Gold, L. M. Ball, and J. A. Swenberg, J. Am. Chem. Soc., 2010, 132, 3388. CrossRef
10. G. Kennedy, P. K. Slaich, B. T. Golding, and W. P. Watson, Chem.-Biol. Interact., 1996, 102, 93. CrossRef
11. M. Sawada, Y. Furukawa, Y. Takai, and T. Hanafusa, Heterocycles, 1984, 22, 501. CrossRef
12. H. Tietz, O. Rademacher, and G. Zahn, Eur. J. Org. Chem., 2000, 2105. CrossRef
13. R. Richter, Chem. Ber., 1968, 101, 3002. CrossRef
14. V. P. Arya and M. S. J. Shenoy, Indian J. Chem., Sect. B., 1976, 14B, 763.
15. H. Oediger and F. Möller, Pat. DE 2640964, 1978 (Chem. Abstr., 88, 190910).
16. A. Nishikawa, Pat. JP 63162718, 1988 (Chem. Abstr., 110, 25475).
17. A. C. Veronese, C. Di Bello, F. Filira, and F. D'Angeli, Gazz. Chim. Ital., 1971, 101, 569.
18. F. D'Angeli, C. Di Bello, and V. Giormani, Gazz. Chim. Ital., 1964, 94, 1342.
19. F. D'Angeli, C. Di Bello, and V. Giormani, Gazz. Chim. Ital., 1965, 95, 735.
20. C. Di Bello, A. C. Veronese, C. Toniolo, F. Filira, and F. D'Angeli, Gazz. Chim. Ital., 1970, 100, 86.
21. N. Matsumura, M. Tomura, R. Mando, Y. Tsuchiya, and S. Yoneda, Bull. Chem. Soc. Jpn., 1986, 59, 3693. CrossRef
22. I. T. Kay, Pat. DE 2451899, 1975 (Chem. Abstr., 83, 97391).
23. R. Richter and H. Ulrich, Chem. Ber., 1970, 103, 3525. CrossRef
24. J. Bödeker, K. Courault, A. Köckritz, and P. Köckritz, J. Prakt. Chem., 1983, 325, 463. CrossRef
25. J. Bödeker, K. Courault, and H. Köppel, Z. Chem., 1982, 22, 445.
26. P. Camus, M. F. Lhomme, and J. Lhomme, Tetrahedron Lett., 1989, 30, 467. CrossRef
27. I. A. Shehata, J. Saudi Chem. Soc., 2003, 7, 207.
28. J. Bödeker, P. Köckritz, and K. Courault, Z. Chem., 1979, 19, 59.
29. M. S. Amine, Egypt. J. Chem., 1998, 41, 267.
30. R. S. Hosmane and N. J. Leonard, J. Org. Chem., 1981, 46, 1457. CrossRef
31. Y. S. Agasimundin, F. T. Oakes, and N. J. Leonard, J. Org. Chem., 1985, 50, 2474. CrossRef
32. Y. S. Agasimundin, F. T. Oakes, L. J. Kostuba, and N. J. Leonard, J. Org. Chem., 1985, 50, 2468. CrossRef
33. N. J. Leonard, R. S. Hosmane, Y. S. Agasimundin, L. J. Kostuba, and F. T. Oakes, J. Am. Chem. Soc., 1984, 106, 6847. CrossRef
34. S. Kumar, F. T. Oakes, S. R. Wilson, and N. J. Leonard, Heterocycles, 1988, 27, 2891. CrossRef
35. C. Glemarec, Y. Besidsky, J. Chattopadhyaya, J. Kusmierek, M. Lahti, M. Oivanen, and H. Lonnberg, Tetrahedron, 1991, 47, 6689. CrossRef
36. A. Kamal and P. B. Sattur, Synthesis, 1985, 892. CrossRef
37. M. Sawada, T. Tanaka, Y. Furukawa, T. Toda, Y. Takai, and T. Hanafusa, Bull. Chem. Soc. Jpn., 1988, 61, 2217. CrossRef
38. S. I. Nagai, T. Ueda, A. Nagatsu, K. Nakaoka, N. Murakami, J. Sakakibara, M. Fujita, and Y. Hotta, J. Heterocycl. Chem., 1998, 35, 329. CrossRef
39. S. Kumar and N. J. Leonard, J. Org. Chem., 1988, 53, 3959. CrossRef
40. C. V. Greco and K. J. Gala, J. Chem. Soc., Perkin Trans. 1, 1981, 331. CrossRef
41. M. R. Mahmoud, M. S. Abd-El-Halim, A. E. F. Ebrahim, and A. M. Radwan, Indian J. Chem., Sect. B., 1996, 35B, 915.
42. A. Lochead, M. Saady, and P.Yaiche, Pat. WO 2138488, 2009 (Chem. Abstr., 152, 119689).
43. N. Sachdeva, A. V. Dolzhenko, and W. K. Chui, ECSOC-12, 2008, a0027. http://www.usc.es/congresos/ecsoc/12/ECSOC12.htm.
44. A. V. Dolzhenko, N. Sachdeva, G. K. Tan, L. L. Koh, and W. K. Chui, Acta Crystallogr., 2009, E65, o684.
45. A. V. Dolzhenko, A. V. Dolzhenko, and W. K. Chui, J. Heterocycl. Chem., 2008, 45, 173. CrossRef
46. A. V. Dolzhenko, M. C. Foo, B. J. Tan, A. V. Dolzhenko, G. N. C. Chiu, and W. K. Chui, Heterocycles, 2009, 78, 1761. CrossRef
47. N. Sachdeva, A. V. Dolzhenko, G. K. Tan, L. L. Koh, and W. K. Chui, Acta Crystallogr., 2010, E66, o2050.
48. F. Ishikawa, A. Kosasayama, S. Nakamura, and T. Konno, Chem. Pharm. Bull., 1978, 26, 3658.
49. M. A. Waly and M. I. Abou Dobara, Pol. J. Chem., 2009, 83, 1601.
50. I. Lalezari and S. Sadeghi-Milani, J. Heterocycl. Chem., 1978, 15, 171. CrossRef
51. R. Bossio, S. Marcaccini, V. Parrini, and R. Pepino, J. Heterocycl. Chem., 1985, 22, 1147. CrossRef
52. J. T. Shaw, M. E. O'Connor, R. C. Allen, W. M. Westler, and B. D. Stefanko, J. Heterocycl. Chem., 1974, 11, 627. CrossRef
53. J. T. Shaw, D. M. Taylor, F. J. Corbett, and J. D. Ballentine, J. Heterocycl. Chem., 1972, 9, 125. CrossRef
54. O. Ceder and K. Rosen, Acta Chem. Scand., 1973, 27, 2421. CrossRef
55. M. A. Rossman, N. J. Leonard, S. Urano, and P. R. LeBreton, J. Am. Chem. Soc., 1985, 107, 3884. CrossRef
56. R. A. E. Winter and T. J. Villani, Pat. US 3886157, 1975 (Chem. Abstr., 83, 193110).
57. K. Hoegerle and H. Brechbühler, Pat. DE 2450119, 1975 (Chem. Abstr., 83, 97390).
58. Y. A. E. S. Issac, Bull. Chem. Soc. Jpn., 1999, 72, 503. CrossRef
59. M. S. R. Murty, T. Ramalingam, and P. B. Sattur, J. Heterocycl. Chem., 1990, 27, 949. CrossRef
60. A. V. Dolzhenko, W. K. Chui, and A. V. Dolzhenko, J. Heterocycl. Chem., 2006, 43, 1513. CrossRef
61. H. Balli, S. Gunzenhauser, I. J. Fletcher, and D. Bedekovic, Pat. DE 3314195, 1983 (Chem. Abstr., 100, 87256).
62. W. C. Anthony, Pat. US 3354159, 1967 (Chem. Abstr., 68, 29727).
63. R. H. Prager, T. K. Rosenzweig, and Y. Singh, Aust. J. Chem., 1992, 45, 1825. CrossRef
64. C. Donati, W. K. Janowski, R. H. Prager, M. R. Taylor, and L. M. Vilkins, Aust. J. Chem., 1989, 42, 2161. CrossRef
65. J. T. Shaw and J. Ballentine, J. Chem. Soc. D, 1969, 1040. CrossRef
66. I. Sosič, B. Štefane, A. Kovač, S. Turk, D. Blanot, and S. Gobec, Heterocycles, 2010, 81, 91. CrossRef
67. J. T. Shaw, J. E. Babin, L. A. Sensenig, G. F. Acciai, M. C. Fair, T. W. Coffindaffer, and W. M. Westler, J. Heterocycl. Chem., 1985, 22, 255. CrossRef
68. R. A. E. Winter and T. J. Villani, Pat. US 3887554, 1975 (Chem. Abstr., 83, 147505).
69. J. T. Shaw, R. J. Thomas, S. A. Herold, G. A. Gfesser, and R. A. Isovitsch, J. Heterocycl. Chem., 1991, 28, 1835.
70. O. Ceder and K. Vernmark, Acta Chem. Scand., Ser. B, 1977, 31, 235.
71. R. A. Houghten, Proc. Natl. Acad. Sci. USA, 1985, 82, 5131.
72. C. E. Hoesl, A. Nefzi, and R. A. Houghten, J. Comb. Chem., 2004, 6, 220.
73. A. Del Pra, C. Di Bello, and M. Mammi, Ric. Sci., 1967, 37, 754.