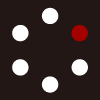
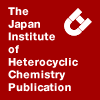
HETEROCYCLES
An International Journal for Reviews and Communications in Heterocyclic ChemistryWeb Edition ISSN: 1881-0942
Published online by The Japan Institute of Heterocyclic Chemistry
e-Journal
Full Text HTML
Received, 15th February, 2011, Accepted, 15th March, 2011, Published online, 25th March, 2011.
DOI: 10.3987/COM-11-12175
■ Feasible Synthesis of the GPR40 Antagonist by Constructing 2-Thiouracil Ring via Acid Mediated Cyclization
Yongfeng Zhao and Jiayu Liao*
Department of Bioengineering, Bourns College of Engineering, University of California at Riverside, 900 University Avenue, Riverside, CA 92521, U.S.A.
Abstract
GW1100 is an antagonist of GPR40 identified by high throughput screening recently. The feasible synthesis of GW1100 has been developed in mild conditions. The key step involves the cyclization of the 2-thiouracil heterocycle under acidic conditions at room temperature.GPR40 is a G-protein coupled receptor that is highly expressed in the beta cell of pancreatic islets, and its activation by long-chain free fatty acids enhances glucose-stimulated insulin secretion.1 The gene knockout mice of GPR40 show resistance to high fat diet-induced diabetic symptoms.2 Therefore it has been identified as a possible novel target for the treatment of type 2 diabetes.
There is no clear understanding to date whether agonist and antagonist of GPR40 would provide beneficial effects for the treatment of type 2 diabetes. To learn more about the pharmacology of GPR40 and about the implications of receptor activation and inhibition, the development of a synthetic agonist and antagonist would be very helpful in GPR40 proof-of-concept studies as its functional mode.
Recently, the first selective antagonist of GPR40, GW1100, was identified by high-throughput screening (HTS).3 At first, this compound was developed as phosphodiesterase A2 inhibitor.4 This compound was shown to completely inhibit the enhancement of glucose-stimulated insulin secretion mediated by agonist and to partially inhibit the enhancement mediated by nature ligand (e.g. linoleic acid).
GW1100 (11) is an attractive molecular tool to further study the biological activities of GPR40 in vitro and in vivo. The structure-activity relationship (SAR) of 11 with its receptor GPR40 has not been explored. Large scale synthesis of compound is also demanded to study the pharmacological effects of GPR40 in animals. Therefore, facile synthetic route is urgently needed to prepare 11. However, there is no full report of synthesis of 11 in the literature.
To synthesize 11, the key step is to construct a 2-thiouracil ring. Though there are many studies show that substituted 2-thiouracil analogue have remarkably contributed to biological and medicinal chemistry, few works have reported the synthesis of N-substituted 2-thiouracil ring, and strong basic conditions and high temperature have usually been used.4-6 In this work, we described the total synthesis of 11 using acidic conditions for ring closure. To the best of our knowledge, this is the first paper reporting feasible total synthesis of 11.
The synthesis of 11 started with the inexpensive 5-bromo-2-chloropyrimidine 1 (Scheme 1). Thus, nucleophilic displacement of 1 afforded 5-bromo-2-ethoxypyrimidine 2 according to modification of procedures in the literature.7,8 Our method for preparation of 2, using freshly prepared sodium ethoxide and absolute alcohol as a solvent to improve the solubility of reactant, considerably improved the yield to over 95%. The coupling reaction of 2 with ethyl acrylate was conducted in the presence of a catalytic amount of the palladium diacetate-triphenylphosphine complex. The reaction mixture was heated at 140 oC in a sealed tube to afford ethyl 3-(2-ethoxypyrimidin-5-yl)acrylate 3 in 64% yield.9,10 The reduction of 3 was conducted in ethanol at room temperature to give ethyl 3-(2-ethoxypyrimidin-5-yl)propionate 4 in a yield of 80%.
Compound 4 was subjected to formylation reaction using methyl formate and sodium ethoxide as a base,11,12 then the resultant was methylated by dimethyl sulfate to obtain ester 5 in a total yield of 53%. We found that the application of freshly prepared sodium ethoxide can depress the unexpected by-products which showed high polarity comparing to the product. Compound 5 was obtained as the mixture of 2:3 ethyl and methyl ester which was determined by 1H NMR after isolation.
As shown in Scheme 2, the resulting ester mixture 5 was hydrolyzed directly under 2 M LiOH to give crystalline acid 6 in 88% yield. The latter acid 6 was treated with oxalyl chloride in the presence of a drop of DMF to provide the acid chloride 7.13,14 The acid chloride 7 was then coupled with KSCN to give the corresponding isothiocyanate derivative 8.15,16 After changing the solvent into DMF, the compound 8 was reacted immediately with 4-aminobenzoate to give intermediate 9. This intermediate then underwent next step without isolation.
In the literature, strong basic conditions and high temperature have been usually applied for similar cyclization reaction.3-5 For this case (Scheme 3), we conducted ring-closure reaction under acidic conditions. After acidifying the solution of intermediate 9,17 compound 10 was synthesized in a total yield of 27% from 6. This step was the key step for the synthesis of target molecule.
Finally, compound 10 was subjected to S-alkylation with 4-flurobenzyl bromide to give target product 11 in moderate yield.5 The 1H NMR match the data reported in reference 4.
In conclusion, we have developed the acid mediated-cyclization route for the synthesis of GW1100. To the best of our knowledge this is the first report of feasible synthesis of GW1100. Significantly, the route requires very mild conditions and utilizes inexpensive starting material 5-bromo-2-chloropyrimidine 1. Application of this methodology to synthesize various pyrimidine derivatives can be explored in the future.
EXPERIMENTAL
General
The 1H NMR and 13C NMR were recorded on Varian VRX 300, 400 spectrometers, and chemical shifts were expressed in parts per million (δ) relative to internal CDCl3. Melting points were determined on Buchi Melting Point B-545 and are uncorrected.
THF, CH2Cl2 and toluene were distilled prior to use. DMF and MeCN was anhydrous solvent obtained from Aldrich. All other reagents and solvents were obtained from commercial sources and were used without any further purification. Reactions were routinely carried out under an atmosphere of dry argon with magnetic stirring. Flash chromatography was generally performed on silica gel. The yields were calculated after isolation.
5-Bromo-2-ethoxypyrimidine (2)
Sodium (0.220 g, 9.56 mmol) was put into dry EtOH (40 mL) under 0 °C, and the mixture was stirred at rt until the sodium disappeared. To the resulting solution, 5-bromo-2-chloropyrimidine (1.5 g, 7.5 mmol) was added. The mixture solution was stirred at rt overnight. After removing of EtOH under reduced pressure, the residue was partitioned between water and ethyl ether. The organic phase was washed with brine and dried over anhydrous Na2SO4. After evaporation of solvent, the residue was purified by flash chromatography on silica gel (Hexanes : Et2O = 5 : 2) to give compound 2 as white solid (1.44 g, 7.1 mmol, 95%). 1H NMR (400 MHz, CDCl3, 25 oC) δ 1.43 (t, J = 7.2 Hz, 3H), 4.40 (q, J = 7.2 Hz, 2H), 8.51 (s, 2H); 13C NMR (100 MHz, CDCl3, 25 oC) δ 14.3, 64.2, 111.6, 159.5, 163.8.
Ethyl 3-(2-ethoxypyrimidin-5-yl)acrylate (3)
A mixture of 2 (0.300 g, 1.48 mmol), triphenylphosphine (0.0093 g, 0.0356 mmol), palladium (II) acetate (0.0043 g, 0.0192 mmol), ethyl acrylate (0.6 g, 5.25 mmol) and triethylamine (1 mL) were heated at 140 °C with stirring under argon for 16 h. After cooling to room temperature, the ethyl acrylate was removed and the residues were partitioned between EtOAc and water. The organic phase was washed with brine and dried over anhydrous Na2SO4. After evaporation of solvent, the residue was purified by flash chromatography on silica gel (Hexanes : Et2O = 1 : 1) to give compound 3 as white solid (0.210 g, 0.945 mmol, 64%). 1H NMR (400 MHz, CDCl3, 25 oC) δ 1.34 (t, J = 6.8 Hz, 3H), 1.45 (t, J = 7.2 Hz, 3H), 4.27 (q, J = 7.2 Hz,, 2H), 4.45 (q, J = 6.8 Hz, 2H), 6.43 (d, J = 16.4 Hz, 1H), 7.56 (d, J = 16.4 Hz, 1H), 8.65 (s, 2H); 13C NMR (100 MHz, CDCl3, 25 oC) δ 14.2, 14.3, 60.8, 64.1, 118.8, 122.1, 137.3, 158.4, 165.7, 166.2.
Ethyl 3-(2-ethoxypyrimidin-5-yl)propionate (4)
A suspension of 3 (0.210 g, 0.945 mmol) in EtOH (2 mL) and 10% Pd/C (0.012 g), triethylamine (0.2 mL) was hydrogenated at 50 psi overnight. The catalyst was filtered off and the filtrate was evaporated. The residue was dissolved in CH2Cl2, washed by saturated aqueous NH4Cl and brine respectively, and dried over anhydrous Na2SO4. After evaporation of solvent, the residue was purified by flash chromatography on silica gel (Hexanes : Et2O = 1 : 1) to give compound 4 as colorless oil (0.170 g, 0.758 mmol, 80%). 1H NMR (400 MHz, CDCl3, 25 oC) δ 1.23 (t, J = 7.2 Hz, 3H), 1.42 (t, J = 6.9 Hz, 3H), 2.60 (t, J = 6.9 Hz, 2H), 2.86 (t, J = 7.2 Hz, 2H), 4.25 (q, J = 7.2 Hz, 2H), 4.39 (q, J = 7.2 Hz, 2H), 8.35 (s, 2H); 13C NMR (100MHz, CDCl3, 25 oC) δ 14.4, 14.7, 24.8, 35.5, 60.6, 63.5, 126.7, 159.2, 164.4, 172.2.
2-(Methoxymethylene)-3-(2-ethyoxypyrimidin-5-yl)propionic acid ethyl/methyl ester (5)
To a stirring suspension of freshly made EtONa (0.118 g, 1.74 mmol) in THF (0.5 mL) was added dropwise a solution of 4 (0.100 g, 0.446 mmol) and methyl formate (0.100 g, 0.793 mmol) in THF (0.5 mL) at 0 °C. After the mixture was stirred at rt overnight and cooled to 0 °C, the dimethyl sulfate (0.1 mL) was added slowly. After 4 h the solvent was evaporated and the residue was treated with water and extracted with EtOAc. The extracts were washed with water, dried over anhydrous Na2SO4. After evaporation of solvent, the residue was purified by flash chromatography on silica gel (Hexanes : EtOAc = 1 : 1) to give compound 5 as colorless oil 2-(ethoxymethylene)-3-(2-methyoxypyrimidin-5-yl)propionic acid ethyl ester (0.030 g, 0.0945 mmol, 21%) and methyl ester (0.03 g, 0.142 mmol, 32%). 1H NMR (400 MHz, CDCl3, 25 oC) Ethyl ester: δ 1.25 (t, J = 6.9 Hz, 3H), 1.41 (t, J = 7.5 Hz, 3H), 3.44 (s, 2H), 3.88 (s, 3H), 4.15 (q, J = 7.5 Hz, 2H), 4.37 (q, J = 6.9 Hz, 2H), 7.37 (s, 1H), 8.37 (s, 2H); Methyl ester: δ 1.40 (t, J = 7.2 Hz, 3H), 3.42 (s, 2H), 3.66 (s, 3H), 3.85 (s, 3H), 4.36 (q, J = 7.2 Hz, 2H), 7.35 (s, 1H), 8.35 (s, 2H); 13C NMR (100 MHz, CDCl3, 25 oC) δ 14.5, 23.7, 51.4, 61.7, 63.1, 109.1, 126.8, 159.0, 159.6, 163.8, 167.9.
2-(Methoxymethylene)-3-(2-ethyoxypyrimidin-5-yl)propionic acid (6)
A suspension of the ester 5 (0.23 g, 0.86 mmol) in 2 M aqueous lithium hydroxide (2 mL) was stirred at rt overnight. The resulting clear yellow solution was acidified by 1 M HCl to pH = 3 and extracted with CH2Cl2, and dried over anhydrous Na2SO4. White solid 6 (0.18 g, 0.756 mmol, 88%) was obtained after evaporation of solvent. 1H NMR (400 MHz, CDCl3, 25 oC) δ 1.41 (t, J = 7.2 Hz, 3H), 3.44 (s, 2H), 3.91 (s, 3H), 4.37 (q, J = 7.2 Hz, 2H), 7.48 (s, 1H), 8.39 (s, 2H).
1-(4-Ethoxycarbonylphenyl)-5-(2-ethoxypyrimidin-5-ylmethyl)-2-thiouracil (10)
To the solution of 6 (0.086 g, 0.361 mmol) in dry CH2Cl2 (2 mL) one drop of DMF, then oxalyl chloride (0.28 mL) was added slowly at 0 oC. After stirring at rt for 2 h, the solvent was removed and additional toluene was added and removed in vacuum. The solid was slurried in dry MeCN (0.5 mL), and the resulting mixture was dropped into the slurry solution of KSCN (0.070 g, 0.720 mmol) in MeCN (0.2 mL) at 0 oC. After the mixture was stirred at rt for 20 h, the solvent was removed in vacuum, and the resulting solid was dissolved in DMF (0.5 mL). The resulting solution was added to the solution of ethyl 4-aminobenzoate (0.074 g, 0.448 mmol) and triethylamine (0.096 mL) in DMF (0.5 mL). After stirring at rt overnight, 2 M H2SO4 (4 mL) was added and the slurry solution was stirred at rt for 5 h. The resulting mixture was extracted with CH2Cl2 three times and the organic phase was dried over anhydrous Na2SO4. After evaporation of solvent, the residue was purified by flash chromatography on silica gel (Hexanes : EtOAc = 1 : 2) to give compound 10 as pale yellow solid (0.040 g, 0.097 mmol, 27%); Melting point: 206-208 oC; 1H NMR (400 MHz, CDCl3, 25 oC): δ 1.37 (m, 6H), 3.61 (s, 2H), 4.45 (m, 4H), 7.11 (s, 1H), 7.44 (d, J = 6.6 Hz, 2H), 8.23 (d, J = 6.6 Hz, 2H), 8.43 (s, 2H); 13C NMR (100 MHz, CDCl3, 25 oC) δ 14.3, 14.4, 26.9, 61.5, 63.6, 118.4, 123.5, 127.4, 131.2, 141.4, 145.2, 159.4, 164.5, 165.1, 175.9; HRMS (ESI+) (m/z): [M + H]+ Calcd. For C20H21N4O4S: 413.1284; Found: 413.1292.
1-(4-Ethoxycarbonylphenyl)-2-(4-fluorobenzyl)thio-5-(2-ethoxypyrimid-5-ylmethyl)pyrimidin-4-one (11)
To the solution of 10 (0.040 g, 0.097 mmol) and 4-fluorobenzy bromide (0.025 g, 0.13 mmol) in CH2Cl2 (0.5 mL) was added the diisopropylethylamine (0.025 mL). The solution was stirred at rt overnight. After evaporation of solvent, the residue was purified by flash chromatography on silica gel (EtOAc : Hexanes = 4 : 1, then elute with EtOAc : MeOH = 10 : 1) to get 11 (0.024 g, 0.0461 mmol, 48%) as white solid; Melting point: 208-210 oC; 1H NMR (400 MHz, CDCl3, 25 oC) δ 1.40 (m, 6H), 3.67 (s, 2H), 4.39 (m, 6H), 6.95 (m, 3H), 7.26 (m, 2H), 7.37 (d, J = 8.4 Hz, 2H), 8.16 (d, J = 8.4 Hz, 2H), 8.45 (s, 2H); 13C NMR (100 MHz, CDCl3, 25 oC) δ 14.2, 14.4, 27.8, 36.0, 61.7, 63.5, 115.5, 115.6, 121.2, 124.4, 127.4, 131.1, 131.4, 132.8, 139.5, 142.2, 159.5, 162.3, 163.6, 164.4, 164.8, 167.2; HRMS (ESI+) (m/z): [M + H]+ Calcd. For C27H26FN4O4S: 521.1659; Found: 521.1639.
ACKNOWLEDGEMENTS
We are grateful to Dr. Michael Pirrung for generously allowing us to use his lab facilities. We would like to thank Bioengineering department for support of this work.
References
1. Y. Itoh, Y. Kawamata, M. Harada, M. Kobayashi, R. Fujii, S. Fukusumi, K. Ogi, M. Hosoya, Y. Tanaka, H. Uejima, H. Tanaka, M. Maruyama, R. Satoh, S. Okubo, H. Kizawa, H. Komatsu, F. Matsumura, Y. Noguchi, T. Shinohara, S. Hinuma, Y. Fujisawa, and M. Fujino, Nature, 2003, 422, 173. CrossRef
2. P. Steneberg, N. Rubins, R. Bartoov-Shifman, M. D. Walker, and H. Edlund, Cell Metabolism, 2005, 1, 245. CrossRef
3. C. P. Briscoe, A. J. Peat, S. C. McKeown, D. F. Corbett, A. S. Goetz, T. R. Littleton, D. C. McCoy, T. P. Kenakin, J. L. Andrews, C. Ammala, J. A. Fornwald, D. M. Ignar, and S. Jenkinson, Br. J. Pharmacol., 2006, 148, 619. CrossRef
4. F. A. Edward, H. D. M. Bernadette, I. R. John, L. C. Andrew, and S. S. Allan, PCT Int. Appl., 2000, 51; WO0068208.
5. E. Wyrzykiewicz and T. Pospieszny, J. Heterocycl. Chem., 2007, 44, 55.. CrossRef
6. I. Winckelmann and E. Larsen, Synthesis, 1986, 1041. CrossRef
7. C. C. Kofink and P. Knochel, Org. Lett., 2006, 8, 4121. CrossRef
8. D. G. Crosby and A. J. Vlitos, Science, 1958, 128, 480. CrossRef
9. R. F. Heck, J. Am. Chem. Soc., 1969, 91, 6707. CrossRef
10. A. Wada, H. Yasuda, and S. Kanatomo, Synthesis, 1988, 771. CrossRef
11. T. H. Brown, R. C. Blakemore, G. J. Durant, J. C. Emmett, C. R. Ganellin, M. E. Parsons, D. A. Rawlings, and T. F. Walker, Eur. J. Med. Chem., 1988, 23, 53. CrossRef
12. T. Hjelmgaard, I. Sotofte, and D. Tanner, J. Org. Chem., 2005, 70, 5688. CrossRef
13. F. Micheli, A. Pasquarello, G. Tedesco, D. Hamprecht, G. Bonanomi, A. Checchia, A. Jaxa-Chamiec, F. Damiani, S. Davalli, D. Donati, C. Gallotti, M. Petrone, M. Rinaldi, G. Riley, S. Terreni, and M. Wood, Bioorg. Med. Chem. Lett., 2006, 16, 3906. CrossRef
14. C. Sun, X. Zhang, H. Huang, and P. Zhou, Bioorg. Med. Chem. Lett., 2006, 14, 8574. CrossRef
15. T.-B. Wei, Q. Lin, Y.-M. Zhang, and W. Wei, Synth. Commun., 2004, 34, 181. CrossRef
16. O. Hritzova, J. Cernak, P. Safar, Z. Frohlichova, and I. Csoregh, J. Mol. Struct., 2005, 743, 29. CrossRef
17. R. Csuk and Y. v. Scholz, Tetrahedron, 1995, 51, 7193. CrossRef